Fig. 5.1
The normal lens (a) examined by a slit lamp (biomicroscopy). No incident light is reflected by the lens tissue, as would be the case with a lenticular opacity (cataract). This shows the gross cross-sectional features of a lens (b) from a 50-year-old man without a significant clinical cataract. The lens contour is ovoid rather than spherical as found in more elderly individuals. The lens doubles in volume between birth and age 70 years
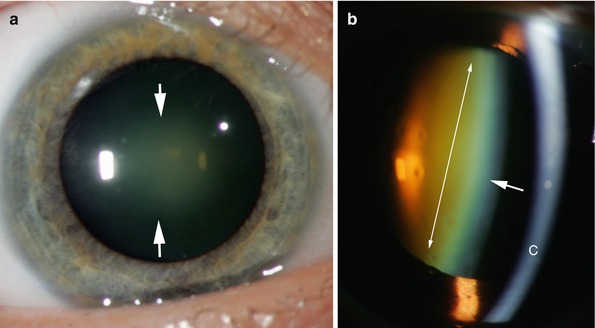
Fig. 5.2
A nuclear sclerotic cataract (a) as seen by direct illumination. The central portion of the lens (nuclear) has become opaque and is reflecting light (arrows). This degree of opacification may be associated with decreased visual acuity. A nuclear sclerotic cataract (b) as seen by slit beam illumination. The nuclear area of the lens (double arrows) has become brown-orange because of the deposition of adrenochrome pigment. The anterior lens capsule is indicated by the single arrow. The cornea is at the level indicated by C. The area between is the fluid-filled anterior chamber. The aqueous normally does not reflect light and the space is “optically empty”
A primary cataract is a lens opacity developing as an expected event in aging. Age-related cataracts many have variable clinical and histopathologic features such as nuclear sclerosis, cortical opacities, or posterior subcapsular opacities. A secondary cataract is a lens opacity developing as part of a systemic disease process or an external event. Examples include developmental (persistent fetal vasculature, or persistent hyperplastic primary vitreous), trauma (posterior subcapsular cataract), endocrine (galactosemic cataract), toxic (siderotic cataract), and neoplasia (zonal cataract from mechanical compression of a ciliary body melanoma). There are no absolute differentiating histopathologic features among the various groups; however, certain patterns many be suggestive of a possible cause. For example, posterior subcapsular cataracts are associated with the use of steroids, severe diabetes mellitus, and intraocular or idiopathic inflammation.
Generally, surgical removal of either a primary or secondary cataract restores functional vision. The opaque natural lens is replaced with an intraocular lens (IOL) made of biocompatible synthetic material (e.g., polymethylmethacrylate [PMMA], acrylic, or silicone) (Fig. 5.3). The lens is placed within the native lens capsule in the posterior chamber of the eye (Fig. 5.4).
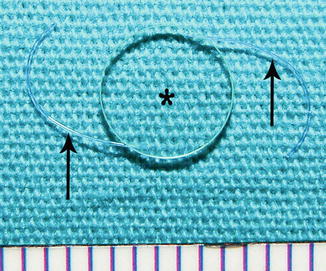
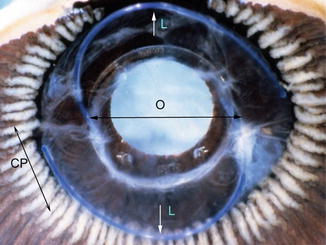
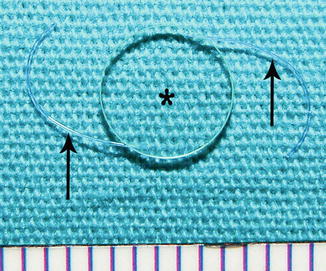
Fig. 5.3
At the time of surgery the cataractous lens is replaced by a synthetic intraocular lens (IOL). Generally only the lens cortex is removed. The lens capsule is left in place and is used as a repository for the replacement lens. There are many different styles; however one of the most commonly used lens is this three-piece, posterior chamber intraocular lens. The central optic focuses light on the retina to form an image. The lens is supported by two lens loops (arrows) within the lens capsule
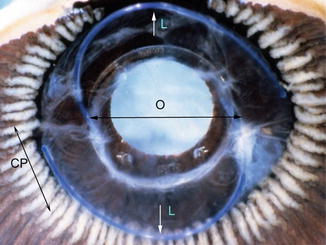
Fig. 5.4
Posterior view of an intraocular lens in situ. Ciliary processes (CP double arrow) are present in the peripheral posterior chamber. The intraocular lens optic (O double arrow) is roughly centered over the pupil. The tips of the arrows mark the insertion of the lens loops (L single arrow) into the lens optic. The lens loops are within the lens capsule, which is translucent except in an opaque region at the loop which has undergone focal fibrous metaplasia of equatorial lens epithelium
The current procedure for removal of a cataract is to use a double-lumen cannula with an ultrasound tip to fragment the lens cortical fibers while leaving the surface capsule intact. Irrigation fluid is introduced through the outer cannula lumen, and fragmented tissue is aspirated though the central lumen of the cannula. The IOL is positioned permanently within the original lens capsule. The fragmented lens tissue is usually of no diagnostic value and is not submitted for histopathologic evaluation.
The pathologist may encounter specimens containing lens material in cases of enucleation (removal of the entire eye), evisceration (removal of the cornea and intraocular contents), and exenteration (removal of all of orbital tissue including the globe). In many cases, evaluation of alteration of the lens position and character is helpful in determining mechanisms of end-stage glaucoma, a common final pathway leading to enucleation of the eye. Lens tissue may be an important finding in extruded intraocular contents from a ruptured globe, a vitreous aspirate, or surgical removal of a nonfunctioning IOL (Fig. 5.5). Malignant transformation of crystalline lens material has not been observed [3].
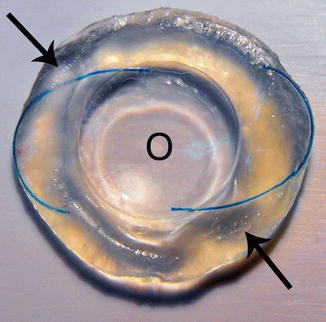
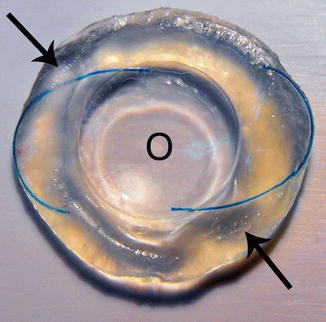
Fig. 5.5
This illustration demonstrates the desired position of an intraocular lens within the lens capsule. The optic (O) is placed in the visual axis. The intraocular lens loops (arrows) are encased in the residual cortex. The IOL is entirely within the lens capsule. This specimen is from an eye removed as part of an exenteration procedure in a case of adenoid cystic carcinoma of the lacrimal gland. The eye was not directly affected by the malignancy
5.2 Embryology, Anatomy, and Development
The crystalline lens is formed from the surface ectoderm at the 28th day of gestation at a point of physical contact with elements of neuroectoderm that have extended laterally from the anterior neural groove. The surface ectodermal cells elongate and penetrate internally to form a sphere of cells (the lens vesicle), which detaches from the surface. With the support of surrounding neural crest tissue, the lens matures by elongating the cells of the posterior hemisphere, which come to occupy the central lumen of the sphere to form a solid cellular structure. As the posterior cells elongate to form the primary lens fibers, most of the cell organelles and the entire lens nucleus involute creating an “anucleate” cell with homogeneous cytoplasm. These cell structure characteristics remain throughout life and contribute to the ability of lens tissue to efficiently transmit light [4].
The anterior hemisphere cells remain and function in proliferation of new lens fibers from stem cells near the lens equator and are essential for maintaining diffusion gradients for glucose and other molecules necessary to sustain lens fibers (Fig. 5.6). Initially, development of the lens is maintained by diffusion from surrounding tissues. As the structure enlarges, there is a transient vascular supply with contributions from the primitive iris vasculature and the posterior hyaloid artery system. Near the time of birth, this transient vascular system, the tunica vasculosa lentis, involutes by apoptosis, as the primary fibrovascular vitreous is replaced by acellular secondary vitreous (Figs. 5.7 and 5.8). Tight adhesions form between the lens capsule and the anterior vitreous in focal areas. Nutrition supplied by the temporary vascular system is replaced by a transudate of plasma (aqueous fluid which contains no red blood cells) produced by the nonpigmented epithelium of the ciliary processes. At this point of development, the fundamental organizational anatomy of the crystalline lens is complete and changes very little throughout life.
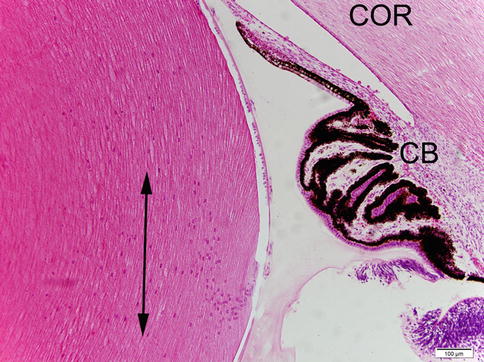
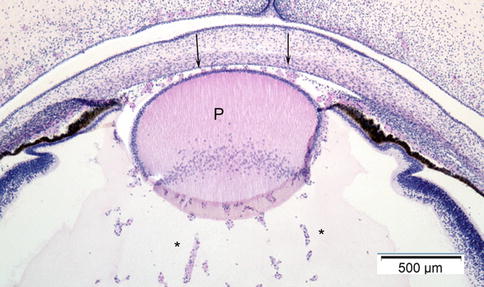
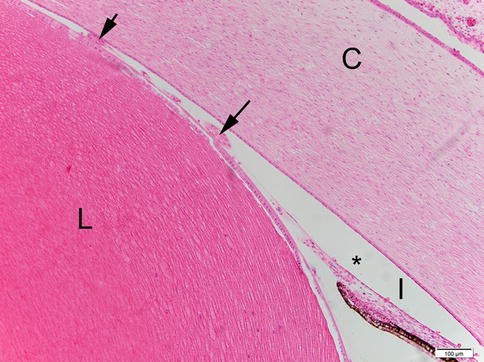
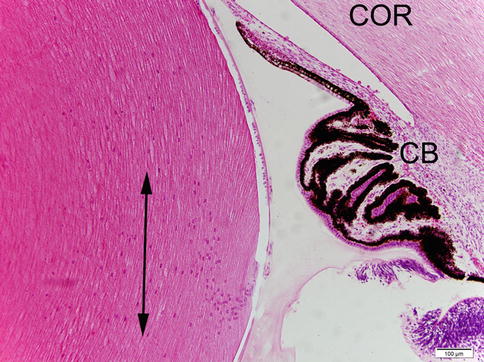
Fig. 5.6
In the developed lens, epithelial cells are only present on the anterior hemisphere. The posterior hemisphere is tightly adherent to the anterior vitreous face. New lens fibers are produced at the lens equator in the region of zonular insertions where cell nuclei separate from the lens capsule. These lens stem cells form a curvilinear arrangement, the lens “bow” indicated by the double headed arrow. COR indicates position of the cornea. CB indicates the position of the ciliary body
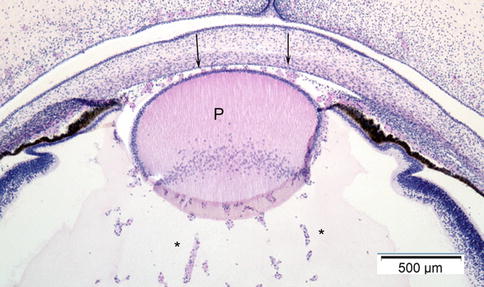
Fig. 5.7
The developing eye at an early stage of embryogenesis. The posterior lens epithelial cells have migrated anteriorly as primary lens fibers (P) to obliterate the cavity of lens vesicle. At this point nutrition to the lens is supplied by the anterior tunica vasculosa lentis (arrows) and the posterior tunica vasculosa lentis
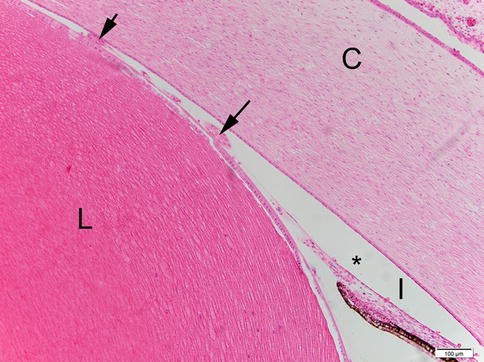
Fig. 5.8
Early in the development of the eye, the nutritional support of the lens (L) is provided by a network of vessels (tunica vasculosa lentis) (arrows) until aqueous is produced by the ciliary body. The tunica vessels of the anterior hemisphere originate from the vascular system of the iris (I) via channels from the minor arterial circle of the ciliary body. The position of the cornea is indicated by (C)
The crystalline lens is located in the anterior segment of the eye bordered anteriorly by the cornea, laterally by the trabecular meshwork and the ciliary body, and posteriorly by the anterior vitreous face (Fig. 5.9). The anterior segment is divided into anterior and posterior chambers by the iris diaphragm. The pupil of the iris diaphragm allows aqueous fluid formed by the ciliary processes in the posterior chamber to circulate to the anterior chamber and exit the eye through the trabecular meshwork. The pupil also has optical functions in adjusting the quantity and quality of light entering the eye. The crystalline lens measures, in the adult, approximately 9–9.5 × 9–9.5 in diameter and ×3.5–4.5 from the anterior to the posterior surface. The lens is suspended in the posterior chamber by delicate, acellular elastic fibers, the zonules, which originate at the vitreous base and portions of the ciliary processes. The zonules insert directly to the lens capsule anteriorly and posteriorly near the equator but do not involve the central visual axis of the lens (Fig. 5.10a, b) [3].
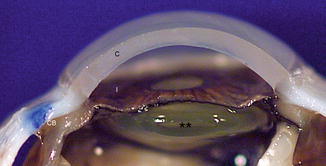
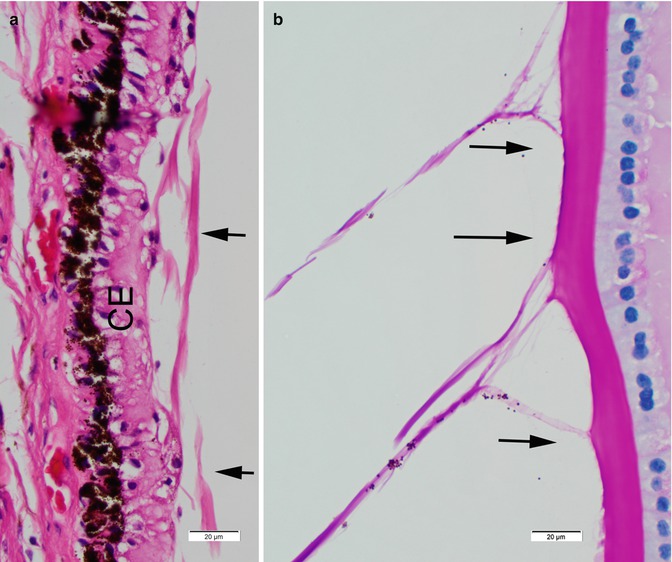
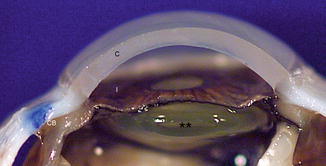
Fig. 5.9
The lens (**) is located in the posterior chamber behind the iris diaphragm (i) and within the ring of the ciliary body (CB). The cornea (C) is the most powerful optical element of the eye. There is no cataract in this case
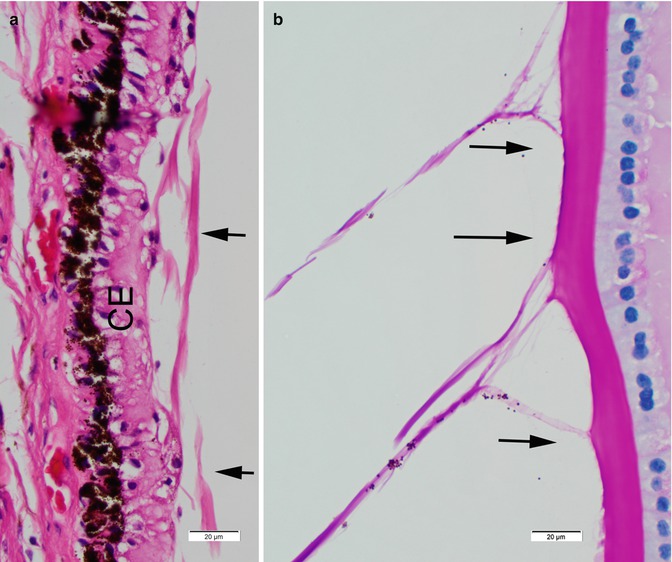
Fig. 5.10
The zonules suspend the lens in the posterior chamber. These acellular fibers (arrows) arise from the nonpigmented epithelium of the pars plana (CE) just anterior to the vitreous base (a) and pass through the fluid-filled posterior chamber. The zonular fibers insert onto the surface of the lens capsule (b) in the region of the lens equator. In addition, the zonules along with the muscles of the pars plicata participate in changing the lens contours in accommodation
The lens capsule, one of the thickest basement membranes of the body, is composed of type IV collagen, laminin, and heparin sulfate. This semipermeable membrane is thickest in the region of zonular insertion laterally and is thinnest at central anterior surface and the central posterior surface to maximize transmission of light. The anterior capsule thickens throughout life by the addition of basement membrane secreted by the anterior hemispheral epithelial cells. There are no similar cells on the posterior surface of the lens; therefore the posterior lens capsule remains thin throughout life (Fig. 5.11a, b) [5].
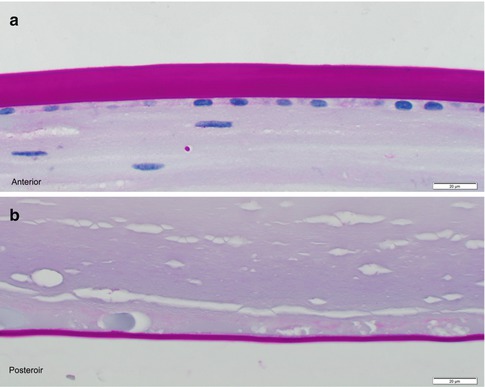
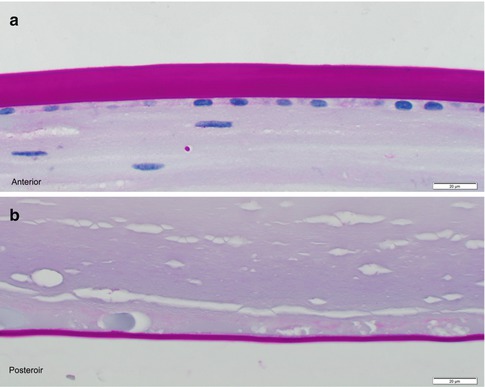
Fig. 5.11
The lens capsule of the anterior hemisphere (anterior) is associated with active lens epithelial cells, which add to the capsular thickness throughout life. The lens capsule of the posterior hemisphere is not associated with active lens epithelial cell and remains thin throughout life
The overall configuration of the lens is that of a biconvex oval that becomes more spherical with advancing age. The structure is completely cellular with essentially no extracellular space. There is no innervation. There is no direct vascular supply indicating that the metabolic demands of the lens are met completely by diffusion across the lens capsule from surrounding aqueous. Interruption of aqueous flow will cause degeneration of the lens as is observed in conditions such as acute narrow angle glaucoma.
The original intraocular lens was developed during World War II when surgeons caring for injured Spitfire pilots noted that intraocular foreign body fragments from the cockpit canopy material were well tolerated and appeared to be biostable. The material, Perspex or polymethyl methacrylate (PMMA), was used to fabricate a replacement lens of roughly the same dimensions as the native crystalline lens. The type of surgery commonly used during that time, extracapsular cataract extraction, allowed the lens to be placed in the posterior chamber without additional support. The lens, however, was quite heavy and tended to dislocate into the anterior chamber or into the vitreous. Despite these problems, many of the IOLs were retained and provided serviceable vision for decades. Over the last 50 years, the design of IOLs and the method of placement have improved considerably. A folded IOL is now injected through a large-bore cannula via a small (2.6 mm clear corneal incision) to spontaneously uncurl and position within the posterior chamber and within the lens capsule. Dislocation from the posterior chamber is currently an infrequent occurrence. Visual recovery from cataract surgery now generally restores vision to the full potential of the person’s retina. Coexisting macular degeneration is one of the major limiting factors to full vision rehabilitation [6].
Intraocular lenses are generally one of two basic designs (Fig. 5.12). The first is a lens constructed of three pieces: a central optic fabricated to the optical power needed to correct the cataract patient’s anticipated residual refractive error and two loops swedged into the optic to expand and support the optic within the lens capsule. The second category of design is a plate configuration that is a single flat piece of synthetic material with the optical power located in the center of the lens and the maximal dimensions of the lens calculated to optimally stabilize the device within the lens capsule. There are literally hundreds of other designs some with special applications such as implanting the lens anterior to the iris diaphragm in the anterior chamber [6].
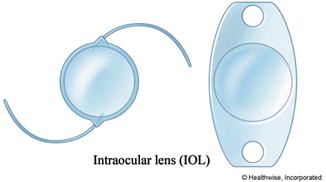
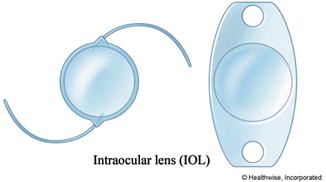
Fig. 5.12
There are two general designs of intraocular lenses used commonly: the three-piece intraocular lens (two loops and a central optic) and a one-piece plate lens. Both of these types of lenses are placed in the lens capsule in the posterior chamber. Another type of lens may infrequently be placed in the anterior chamber
Except for silicone and Supramid, most of the intraocular lens materials are soluble in the organic solvents used for the preparation of paraffin-embedded material. Generally, the presence of an intraocular lens in sectioned tissue depends on recognizing the void in tissue (shadow) left by the intraocular lens material (Fig. 5.13). Generally, there is no tissue reaction to the material itself; however fibrous tissue may form in the immediate environment of the IOL (Fig. 5.14). Occasionally, there may be an inflammatory reaction to the materials of the lens loops (Fig. 5.15).
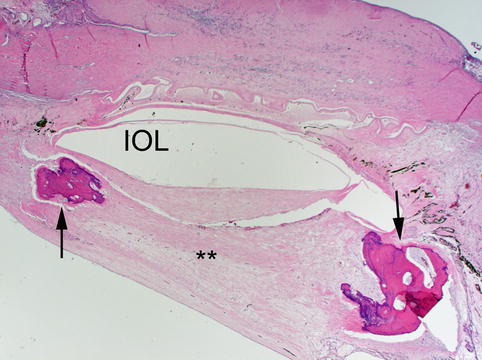
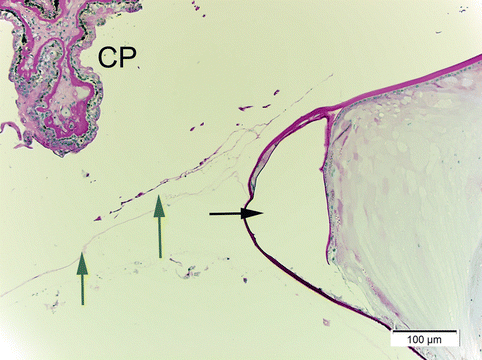
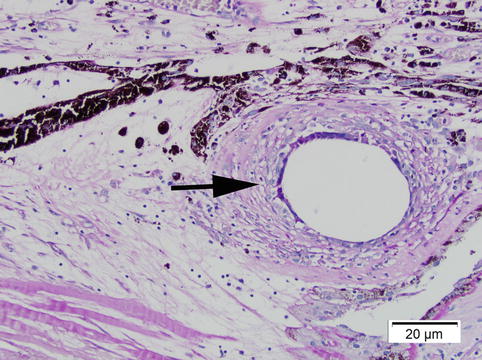
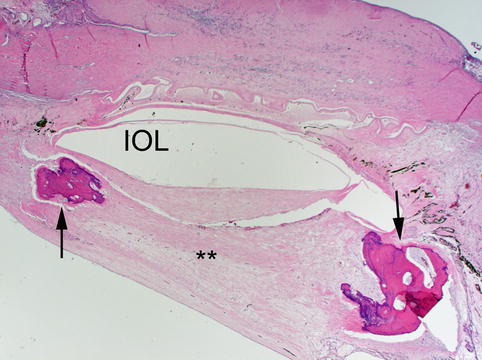
Fig. 5.13
A biconvex intraocular lens optic (IOL) was present in the posterior chamber; however the material was solubilized by the organic solvents used in tissue processing. Fibrous tissue has formed in the posterior capsule and in the space behind the lens (**) resulting in a cyclitic membrane. Osseous metaplasia (arrows) has formed in the abnormal fibrous tissue
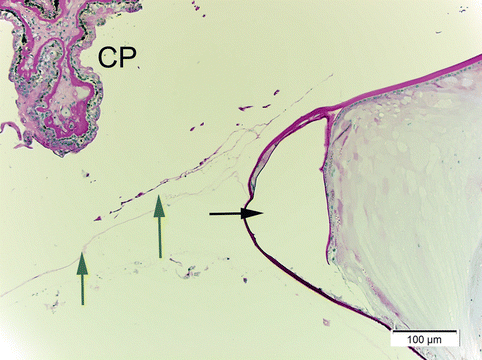
Fig. 5.14
An intraocular lens has been placed in a lens capsule with a moderate amount of lens cortex remaining. The lens loop material has dissolved but the negative image of its cross section is apparent (black arrow). Lens zonules (Green arrows) and the tips of ciliary processes (CP) are evident
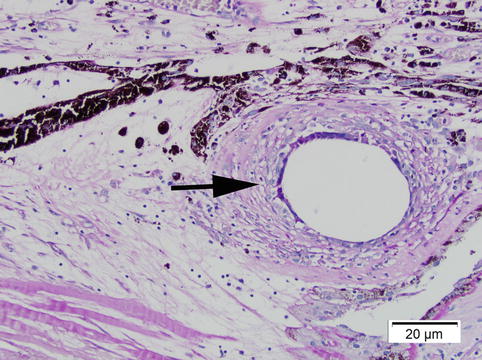
Fig. 5.15
An intraocular lens loop (arrow) has been placed in the posterior chamber posterior to the iris but outside of the lens capsule. The loop material has stimulated an inflammatory response either because of the loop material itself or because of some antigen on the surface of the material
Histopathologic features of congenital lens abnormalities are similar to those resulting from degeneration or injury. Congenital variations of the lens consist of failure of formation of transparent tissue, alteration of contours and refractive index affecting optical power, and abnormal positioning of the lens due to dysfunction of zonular support. Most of these features are readily apparent by clinical examination but are subtle or obscure by histopathologic examination (Fig. 5.16a, b) [7].
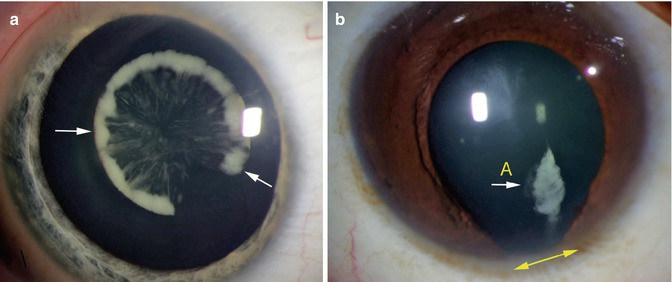
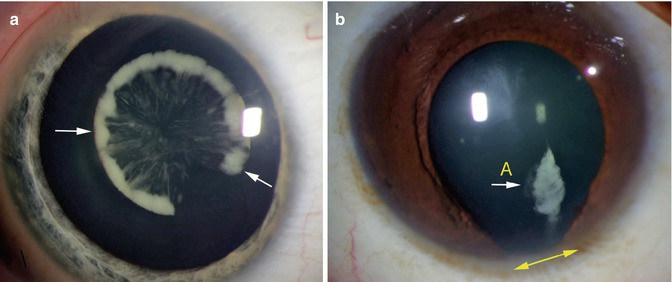
Fig. 5.16
Two cases of congenital cataract (lens opacity present at birth). The opacity in a is at level of the nucleus. The opacity surrounds the margin of the nucleus leaving the periphery relatively clear. The opacity in (b) is in the superficial cortex (A) and is associated with a defect (coloboma) of the iris (double arrow.) Estimating the vision in cases of congenital cataract is difficult; the vision in (a) was 20/30, while the vision in (b) was 20/80
Congenital absence of the crystalline lens in an otherwise normally developed globe has not been observed. The most likely reason is that the lens is one of two fundamental differentiating tissues necessary for eye development. In some cases the lens may have formed but then has degenerated. Malformation of lens fibers leading to focal opacification of the lens during gestation is a relatively common event and is usually dependent on toxic changes or microbial infection of the lens environment. If the event is brief and minimal, the opacity may be seen clinically but may not progress of cause loss of ocular function.
One of the clearest examples of histopathologic verification of a congenital lens abnormality is a phakomatous choristoma (Zimmerman tumor) [8]. This lesion consists of an asymptomatic area of palpable induration of the subcutaneous tissue the lower eyelid, usually nasally, and most often identified in early childhood. The indurated tissue evolves from an ectopic rest of primitive lens material characterized by a prominent PAS-positive membrane surrounding large epithelial cells that are similar in appearance to the cells of a posterior subcapsular cataract (Fig. 5.17). The entity is treated with total surgical excision and does not recur.
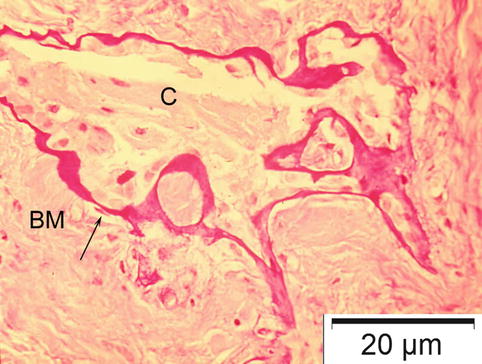
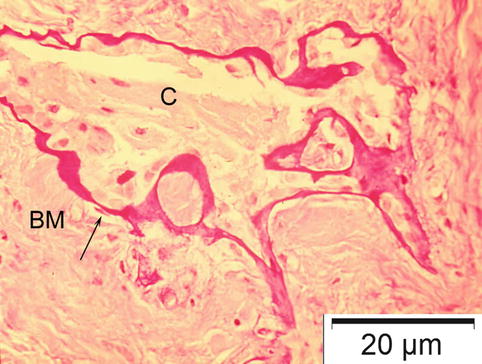
Fig. 5.17
Phakomatous choristoma (Zimmerman tumor) is an ectopic lens tissue in the lower eyelid presenting as an indurated tumor of childhood. It is composed of lens cortical cells (C) surrounded by irregular basement membrane (BM) forming a lens capsule
The most dramatic congenital abnormality of the eye is cyclopia or synophthalmos. This condition results from the failure of development the diencephalon that is most often associated with fetal death. In the autopsy specimens the eye tissue in synophthalmos generally preserves the anterior segments, while there is apparent “fusion” of the posterior segment characterized by major posterior tissue abnormalities. The crystalline lenses may appear to be remarkably normal (Fig. 5.18). Similarly, the lens of a cyclopean eye may have few abnormal features [9].
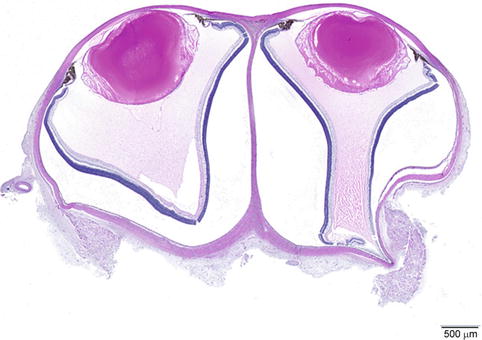
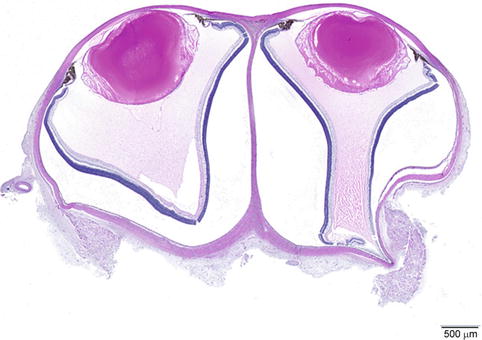
Fig. 5.18
Synophthalmos is a developmental abnormality of the development of the eye early in embryogenesis. There is a fault in the development of the intervening brain making the posterior segment appear to be “fused.” The anterior segment including the lens may be remarkably normal (Courtesy of Alan Prioa, MD, Eastern Ophthalmic Pathology Conference, Duke University, Durham, North Carolina, 2007)
Alteration of the shape of the lens because of abnormalities of the lens capsule can seriously disrupt the refractive function of the lens. One example is lenticonus associated with Alport’s syndrome. Alport’s syndrome is a rare genetically determined disorder generally with an x-linked pattern of inheritance that causes a defect in type IV collagen, a major component of basement membrane including the glomerulus of the kidney and the lens capsule [10]. The weakened capsule allows an increase in the radius of curvature of either the anterior or posterior lens surfaces (Fig. 5.19) causing a marked decrease in visual function. The alteration of curvature is clearly evident by biomicroscopy. The lens may contain a congenital or an acquired cataract. By light microscopy the lens capsular thickness is reduced by two-thirds and appears more fibrillar than the normal lens capsule. By electron microscopy large numbers of partial capsular dissidences are present throughout the lens capsule that contains cellular debris [11].