Taste
Natasha Mirza
The goal of the chemosensory system is the detection and discrimination of foods and the initiation and sustenance of ingestion. This system is present in both vertebrates and invertebrates and well developed even in a newborn infant. It is estimated that more than 2 million Americans have some kind of chemosensory disorder (1). What is proven so far has been that a loss or alteration of taste affects nutritional intake and leads to multiple nutrition-related medical problems (2). For this reason, it is important to understand the mechanism of taste and to identify some of the effects of taste dysfunction and the means of reducing them. This chapter briefly reviews the anatomy and physiology of taste including taste transduction; clinical evaluation of the taste system; the impact of aging, medications, and other disease states; and discusses some of the methods to help decrease some of the harmful effects of taste loss on both nutrition and quality of life.
DEVELOPMENT OF THE TASTE SYSTEM
Morphologic evidence shows that there is a functional taste system present in utero. Even premature infants respond pleasurably to sucrose and glucose. The hedonic system of pleasantness is also well developed in infants (3). The acceptance of sweet and rejection of bitter seem to be hardwired while the effect associated with odors depends much more on experience.
ANATOMY OF THE TASTE SYSTEM
In mammals, taste buds are located throughout the oral cavity, in the pharynx, the laryngeal epiglottis, and at the entrance of the esophagus. Taste buds on the dorsal lingual epithelium are the most numerous (total number of taste buds, all classes, approximately 4,600 per tongue). Here, taste buds are contained within three major classes of papillae, the fungiform, foliate, and circumvallate, while the filiform papillae are nongustatory. Fungiform papillae are most densely distributed on the tip and sides of the tongue. These papillae generally contain one to several taste buds per papilla (4). They are innervated by the chorda tympani branch of the facial (VII cranial) nerve and appear as red spots on the tongue because they are richly supplied with blood vessels. The total number of fungiform papillae per human tongue is around 200. The foliate papillae are situated on the edge of the tongue slightly anterior of the circumvallate line. They are predominantly sensitive to sour tastes and are innervated by the glossopharyngeal (IX cranial) nerve and average five foliate papillae per side of the tongue with approximately 120 taste buds per foliate papillae. Circumvallate papillae are sunken papillae, with a trough separating them from the surrounding wall. The taste buds are in tiers within the trough of the papillae. They are situated on the surface of the circumvallate line and form an inverted V on the posterior of the tongue. They confer a sour/bitter sensitivity to the posterior one-third of the tongue. They are innervated by the glossopharyngeal (IX cranial) nerve. There are 3 to 13 circumvallate papillae per tongue with about 250 taste buds per papillae. The filiform papillae are mechanical and nongustatory. In addition, there are 2,500 taste buds on the epiglottis, soft palate, and laryngeal and oral pharynx.
In mammals, taste buds are aggregations of 30 to 100 individual elongated “neuroepithelial” cells (50 µm in height, 30 to 70 µm in width) (5). At the apex of the taste bud, microvillar processes protrude through a small opening, the taste pore (4 to 10 µm in diameter) into the oral milieu. Just below the taste bud apex, taste cells are joined by tight junctional complexes. Taste cells are bipolar cells, which connect to the epithelial surface of the oral cavity via dendritic processes and to a nerve axon at the base which invades the bud and ramifies extensively, with each fiber
typically synapsing with multiple receptor cells within the taste bud (Fig. 50.1). There are at least three different types of taste cells although the function of these cells overlaps. The lifespan of a mammalian taste cell is approximately 10 days. As a cell ages, a nerve terminal detaches, finds a developing cell, and forms a new synapse. The new cell has to be of the same taste specificity as the old dying cell; in other words, taste receptor cells act as surface markers to guide nerve fibers to the right cellular target. Cells in each taste bud contain the sensory receptor cells, supporting cells, and basal cells that differentiate into new receptor cells. A single taste bud is all that is needed to provide sensation for all the four tastes so that even the decrease in number of lingual papillae does not necessarily lead to a significant loss of taste.
typically synapsing with multiple receptor cells within the taste bud (Fig. 50.1). There are at least three different types of taste cells although the function of these cells overlaps. The lifespan of a mammalian taste cell is approximately 10 days. As a cell ages, a nerve terminal detaches, finds a developing cell, and forms a new synapse. The new cell has to be of the same taste specificity as the old dying cell; in other words, taste receptor cells act as surface markers to guide nerve fibers to the right cellular target. Cells in each taste bud contain the sensory receptor cells, supporting cells, and basal cells that differentiate into new receptor cells. A single taste bud is all that is needed to provide sensation for all the four tastes so that even the decrease in number of lingual papillae does not necessarily lead to a significant loss of taste.
TASTE TRANSDUCTION
It is important to understand some of the basic science that is involved in taste transduction to understand the effects of pathologic conditions on this mechanism (6). Stimuli entering the mouth first interact with sites on the microvilli within the taste pore. Salts and sour acidic stimuli use ion channels while sweet and bitter substances react with protein compounds. Taste receptors trigger transduction cascades, which then activate synapses and cause the excitation of nerve fibers. A signal is produced, which is then carried to the brain, relaying information on the identity and intensity of the gustatory stimulus. Receptor proteins are located on the apical surface of cells and from there the stimulus enters the cell either via an ion channel as it does for the salty and sour tastes or via second messenger systems like cyclic AMP for bitter and sweet. Once the stimulant enters the cell there is a change in the internal electrical state of the cell, which leads to the secretion of a neurotransmitter and activation of a nerve fiber.
Each taste serves a specific purpose in the maintenance of health of an individual. Salt taste guides the intake of sodium chloride and thus the salt-water homeostasis. Sour helps with the recognition of complex foods. At low concentrations, it is pleasant but once it reaches high concentrations it becomes an unpleasant taste and leads to avoidance. It guides acid-base regulation and is sensitive to extracellular pH changes. The sweet receptor is a classical proteinaceous molecule analogous to an olfactory receptor. It responds to soluble carbohydrates in the oral cavity and regulates caloric intake, nutrition, and energy balance. It uses a second messenger like cAMP to activate a cyclic nucleotide cascade. Sweet taste has a strong hedonic effect and is well developed in infants. Bitter taste like sour is bearable and even to some extent pleasant in low concentrations but is repulsive when strong. Bitter receptors belong to the second messenger system of G proteins. They set off a G-protein-coupled receptor (GPCR)-mediated signal that leads to dual signaling of cAMP and cGMP within the cell. Bitter taste serves as a warning system designed by nature to protect against the ingestion of harmful compounds. Even newborn infants are seen to respond to bitter compounds, like quinine, with distaste (7). Umami as a taste was discovered about 100 years ago and is somewhat similar to sweet taste. It is derived from L-glutamate and is the taste present in Monosodium glutamate (MSG), chicken broth, meat extracts, and aging cheese. Umami receptors act much the same way as bitter and sweet receptors (they involve GPCRs), but not much is known about their specific function. It is thought that the amino acid L-glutamate binds to a type of GPCR known as a metabotropic glutamate receptor (mGluR4). This causes the G-protein complex to activate a secondary receptor, which ultimately leads to neurotransmitter release. The intermediate steps are not known. This taste helps guide the intake of peptides and proteins.
INNERVATION OF THE TONGUE
Taste receptor cells do not have an axon. Information is relayed onto terminals of sensory fibers by transmitters. These fibers arise from the ganglion cells of three cranial nerves. Over the anterior tongue the chorda tympani (a branch of the facial nerve) is sensory for taste and the lingual nerve, a branch of the trigeminal nerve serves general sensation. The chorda tympani “hitchhikes” along the lingual nerve travels through the middle ear and joins the facial nerve in the fallopian canal, then to the geniculate ganglion and terminates in the upper portion of the nucleus solitarius.
Over the posterior tongue, taste is conveyed via the glossopharyngeal (IX cranial) with some branches of the vagus providing additional taste sensation. In this region of the tongue, general sensation is also provided by the same two nerves. The IX nerve travels through the jugular foramen and terminating in the tractus solitarius
(gustatory nucleus). The trigeminal nerve is responsible for detecting the burning sensation caused by certain foods, for example, peppers. The superior laryngeal nerve, a branch of the vagus, innervates taste buds on the laryngeal surface of the epiglottis. Its role in taste is unknown (Fig. 50.2).
(gustatory nucleus). The trigeminal nerve is responsible for detecting the burning sensation caused by certain foods, for example, peppers. The superior laryngeal nerve, a branch of the vagus, innervates taste buds on the laryngeal surface of the epiglottis. Its role in taste is unknown (Fig. 50.2).
![]() Figure 50.2 Innervation of the tongue. (From Geriatric Otolaryngology, eds. Eibling and Calhoun, 2006, Published by Taylor and Francis group.) |
Central Pathways
Taste fibers from cranial nerves VII, IX, and X form part of the fasciculus solitarius and synapse centrally in the medulla (in a thin line of cells called the nucleus of the solitary tract). From there, the information is relayed to the somatosensory cortex for the conscious perception of taste and to the hypothalamus, amygdala, and insula, giving the so-called affective component of taste. This is responsible for the behavioral response, for example, aversion, gastric secretion, and feeding behavior. Nature has provided some of the compensation mechanisms that make taste very robust. These depend on the fact that there is inhibition among the central projections of the taste nerves. Taste nerves normally inhibit one another across the midline. Therefore, when a nerve is damaged there is a decrease in inhibitory impulses from that side, which in turn leads to an increase in responses from the opposite side. This disinhibition of the damaged nerve leads to an overall preservation of taste and total ageusia or loss of taste is very rare. On the other hand, the olfactory system depends on one nerve and is therefore more vulnerable to injury. It is also interesting to note that damage to taste releases inhibition on the trigeminal nerve and causes intensification of sensations like oral burning and oral touch and also makes certain foods, for example, fatty foods less palatable (8).
Genetic Variations
Phenylthiocarbamide and 6-n-propylthiouracil (PROP), chemically related compounds, are probes for genetic variation in bitter taste. Tasters and nontasters are identified based on the individual’s ability to perceive PROP. The perceived intensity grades tasters into medium tasters (PROP is bitter) and supertasters (PROP is very bitter). Compared with supertasters, nontasters have fewer taste papillae on the anterior tongue (fungiform papillae) and experience less negative (e.g., bitterness) and more positive (e.g., sweetness) sensations from certain foods and beverages like alcohol.
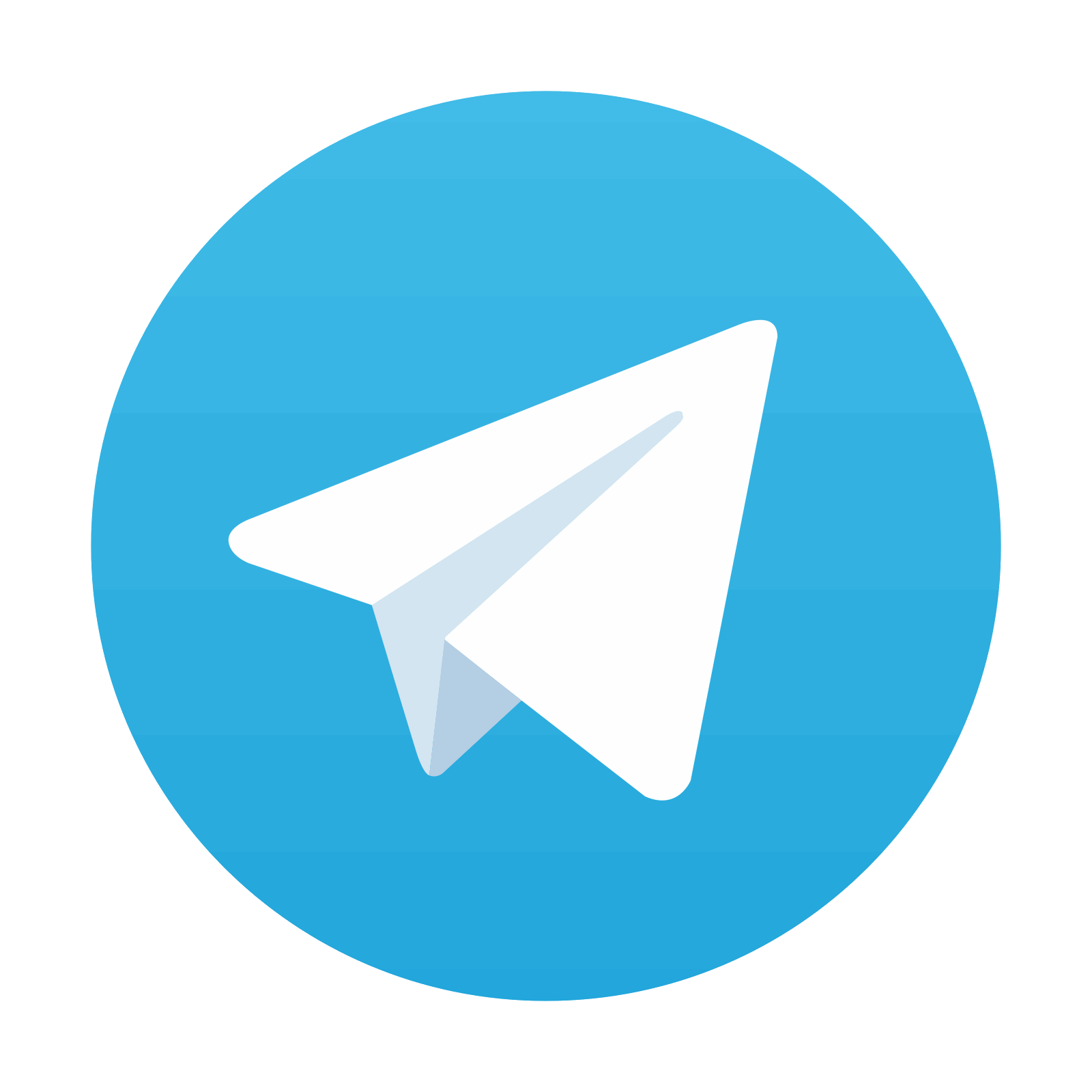
Stay updated, free articles. Join our Telegram channel

Full access? Get Clinical Tree
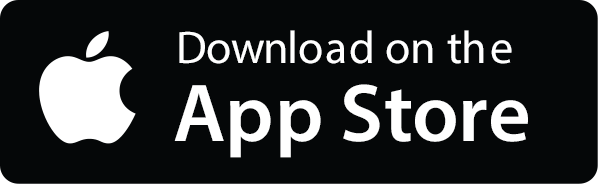
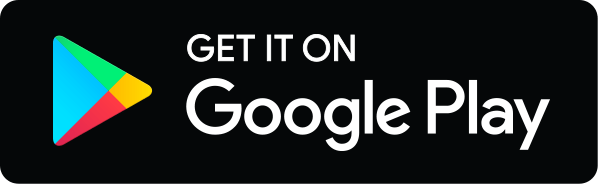