Spectacle Lens Tints and Coatings
Gregory L. Stephens
Darryl J. Meister
Tints and coatings used on spectacle lenses can make vision more comfortable, improve visibility and contrast, protect the eyes from the effects of harmful radiation, and provide a therapeutic effect. Additionally, tints and coatings can serve a purely cosmetic or fashion purpose. This chapter focuses primarily on the optical principles and visual applications of tints and coatings, with an emphasis on prescription spectacle lenses.
Transmittance and Reflectance
When light passes through a spectacle lens, some is reflected at the front surface, some is absorbed or scattered by the lens material itself, and some is reflected at the lens back surface. Transmittance for a given wavelength is defined as the percentage of the incident light that passes completely through a lens. The transmittance can be expressed by the formula:

where reflectance is the percentage of incident light reflected from the two lens surfaces and absorption is the percentage of incident light absorbed by the lens material itself. A plot of transmittance versus wavelength provides the basic information needed to understand the light filtration properties of a tinted lens.
The sensitivity of the human eye varies with wavelength or color. Because of the physiology of the human photoreceptors, the sensitivity of the eye falls off rapidly for colors in both the blue and red ends of the visible spectrum and is highest for wavelengths near the middle of the spectrum. This means that considerably higher quantities of blue or red light are required to elicit the same sense of brightness as, for instance, yellow-green light. A plot of the relative sensitivity of the eye as a function of wavelength is known as the relative luminous efficiency function. During the day, the eye is maximally sensitive to yellow-green wavelengths near 555 nm; this is the photopic response of the eye (Fig. 1). At night, the maximum sensitivity of the eye shifts slightly toward the blue end of the spectrum near 500 nm, an effect known as the Purkinje shift; this is referred to as the scotopic response of the eye. When the light transmitted by a lens is weighted by the photopic relative luminous efficiency function of the eye, the product is referred to as the luminous transmittance of the lens.1,2
The average of the transmittance values over a given wavelength range is the mean or average transmittance of a lens for that wavelength range. Ultraviolet (UV) and infrared (IR) radiation transmittances are commonly specified as mean transmittance over a given spectral range.
Figure 2 shows transmittance curves for clear (untinted) ophthalmic crown glass and CR-39 plastic. Transmittance across the visible spectrum is approximately the same for all wavelengths, indicating that these lenses have no inherent tint. The maximum transmittance of a clear lens is about 92%. This maximum is limited only by reflectance losses (about 4%) at each surface because clear CR-39 plastic and crown glass absorb essentially no light. It is not possible to increase the transmittance beyond this limiting value unless an antireflective coating is applied. An antireflective coating does not just decrease reflectance, it also increases transmittance. A high-quality antireflective coating can decrease the reflectance at each surface of a lens to approximately 0.5%, providing a lens transmittance of approximately 99%.3
Most prescription tinted lenses are specified both by their color and a number that indicates the approximate luminous transmittance of the lens. Light tints, #1 tints, have transmittance values of about 75% to 85% and are used as fashion tints. Medium, or #2 tints, have transmittances of about 50%. These tints are often too dark for indoor wear and not dark enough to be effective sunglasses. Because of these limitations, their use is uncommon. Dark tints, #3 tints, are commonly prescribed as sunglasses and have transmittances of approximately 20%. It is also possible to provide #4 tints of about 10% to 15% transmittance, but these tints are too dark for many people and must be used with caution.
Transmittance values for nonprescription sunglasses may be described in a different manner. The ANSI Z80.3-2001 nonprescription sunglass standard recommends that sunglasses for general purpose use have a luminous transmittance between 8% and 40%.1 Special purpose sunglasses, as might be worn by a mountain climber or someone spending many hours at the beach, have luminous transmittance values between 3% and 8%. Sunglasses for cosmetic use have luminous transmittance values above 40%. The ANSI Z80.3-2001 standard also includes recommendations for traffic signal visibility when wearing nonprescription sunglasses and the UV protection that should be provided by sunglasses.
Methods of Tinting a Lens
Three methods are commonly used to tint a lens. Plastic lenses are usually tinted by dipping them in a hot, water-soluble dye. The dye penetrates a uniform distance into the lens surfaces, providing a tint with the same color and transmittance at all points on the lens. Almost any color is available. Gradient tints, tints that lighten from the top to the bottom of the lens, are made by dipping only a part of the lens in the dye. Lenses can also be dyed with multiple colors. Dye can be removed almost completely from a tinted lens by dipping it in tint neutralizer or “bleach,” a hot alcohol solution. Plastic lenses vary in their ability to accept a tint, as do “hard” or scratch-resistant coatings. Polycarbonate and some mid-index and high-index plastics do not tint well, so lenses of these materials will only accept a dark tint if they have a tintable hard coating. Also, harder (more scratch-resistant) coatings are more difficult to tint. Because of this variability, reliable and repeatable tinting of plastic lenses is as much art as science.
Glass lenses are commonly tinted by the addition of metals or metal oxides to the molten glass mixture.4 Because these oxides are dispersed uniformly throughout the lens blank, transmittance will vary with lens thickness, so this type of tint should not be used for lenses of high power. A minus-power tinted glass lens is darker at its edge than at its center, and a plus-power lens is darker at its center than at its edge. A high plus-power tinted glass lens can be too dark at its center for its intended use.
Some glass lenses are tinted by the application of a coating, usually to the lens back surface. This coating consists of a thin layer of a metal oxide applied using a vacuum deposition process. The coating is of uniform thickness across the lens surface; therefore, transmittance does not vary with lens power or thickness. The colored layer is soft, so a thin layer of a more durable compound is often applied over this first layer to provide some scratch resistance. Even this protective layer is somewhat soft and can be scratched easily, so application to the lens back surface provides some protection. The protective coating is also extremely thin, so interference effects can occur. Light reflected from the lens back surface will therefore be colored, often red, blue, or purple. This coloring may not be cosmetically acceptable.
The silver-colored or copper-colored mirror coatings found on both glass and plastic lenses are also applied by a vacuum deposition process. When these coatings are applied to a tinted lens, the result can be extremely dark sunglasses. For example, a glass sunglass lens of 20% transmittance coated with a mirror coating of 40% transmittance will have a transmittance of 8% (20% × 40%).
The methods used to provide the variable tint to a photochromic lens can differ considerably from the tinting methods just described. Photochromic lenses will be described in a later section.
Tints for Indoor Use
Lightly tinted lenses with transmittances of about 80% are often prescribed for indoor use, both for cosmetic purposes and for comfort. For many years, pink or flesh-colored tints (Fig. 3) have been recommended for patients who complain of glare and discomfort while working under fluorescent lights. Before the advent of plastic lenses, glass lenses capable of absorbing UV radiation had a pink tint. This glass was used routinely in aphakic spectacle lenses to remove some of the UV radiation that otherwise would reach the aphakic retina. The color had little to do with the effectiveness of the lens. Pink tints began to be associated with many desirable features, including UV protection, comfort, and glare protection. Actually, any light tint is useful for patients working under fluorescent lights. The colors are not strong enough to have any color-filtering properties. Lenses that provide complete UV protection (whether tinted or not) are the best option for the patient with aphakia.
Lightly tinted lenses perform a useful function by reducing the intensity of internal reflections produced by the lens. An overhead light source that is brighter than the foreground, such as a fluorescent light, is reflected from the rear surface of the lens to the front and then back through the rear surface into the eye (Fig. 4). Many more internal reflections occur, but after the first three, the reflections are not usually bright enough to affect vision. The reflected light can reduce contrast, and it can also become an in-focus ghost image. Even a light tint attenuates this stray light because the reflected light passes through the lens multiple times and is absorbed on each passage. Pink or other lightly colored tints may have a placebo effect. Also, because the tints reduce the brightnesses of multiple reflections of the lens bevel around the edges of a high minus-power lens (myopic rings), cosmetic appearance may be improved.
![]() Figure 4. Ghost images of light sources can be created by reflections from lens surfaces. These reflections can be more of a problem for low minus-power lenses. |
Fluorescent lights emit small but measurable amounts of ultraviolet B (UVB) radiation,5,6 the radiation from 290 to 315 nm that has been associated with the development of cataracts. Plastic diffusers may absorb some of this radiation, and “egg-crate” diffusers that block a direct view of the light source also attenuate it. However, patients exposed to massed banks of fluorescent lights should have lenses or tints that absorb UV radiation. UVB protection is automatic with all plastic lens materials. The UV radiation protection provided by ophthalmic lenses will be described in more detail in a later section.
Colors other than pink or brown, such as light grays, blues, and lavenders, have at times been popular as fashion tints. Lenses with pronounced coloring should not be recommended for persons with color deficiencies. Strongly colored lenses, especially red or blue lenses, may affect traffic signal visibility, and long-term wear of these lenses may alter color perception even after the lenses are removed.7
Tints for Outdoor Use (Sunglasses)
Controlling Light Levels
Sunglass lens tints are specified by both transmittance and color. The transmittance of visible light by sunglasses should be such that the luminances (brightnesses) of objects in the visual field when outdoors are brought into a comfort zone of 350 to 2,000 candelas per square meter (cd/m2).8 There is often a sense of glare when the dominant luminance in the visual field exceeds this level. Some people prefer less light, but older patients can often tolerate higher levels. Table 1 lists luminances that may be encountered in various situations where sunglasses or tinted lenses might be helpful. Light-colored pavements, most beaches, and grassy areas have luminances such that a 20% transmittance sunglass lens can bring them into the comfort zone. Standard sunglass lenses typically have transmittances of 15% to 25%, and these sunglasses work well for normal outdoor use and for driving. Shady areas are attenuated by sunglasses to about 60 cd/m2, but this level is still adequate.
Table 1 Selected Outdoor Luminance Ranges | ||||||||||||||||||||||||||
---|---|---|---|---|---|---|---|---|---|---|---|---|---|---|---|---|---|---|---|---|---|---|---|---|---|---|
|
Some activities require darker sunglasses (#4 tints) to bring luminances into the comfort zone. These include winter sports, especially those performed at high altitudes, mountain climbing, and flying above the clouds. Even darker sunglasses, with transmittances of 3% to 5%, are popular for these activities when it is necessary to face the bright sky beneath the sun.8 Side-shields or highly wrapped lens designs should be worn with extremely dark tints to maintain retinal adaptation at the level required by the lens transmittance. Extremely dark sunglasses are not recommended for driving because they blacken shady roadside areas, especially when the driver is entering the shade from a brighter area. A sun visor is a better solution. The ANSI Z80.3-2001 standard for nonprescription sunglasses recommends that tinted lenses with transmittances of less than 8% not be used for driving.1
Sunglasses and Visibility
A patient wearing spectacles loses contrast when viewing a dark object against a bright sky background. This occurs because the bright light from the sky is reflected multiple times within the spectacle lens and is eventually superimposed on light from the foreground object. Sunglasses, or even a lightly tinted lens, can greatly reduce this reflected light and increase contrast because the multiple reflections will be attenuated each time they pass through the lens.
Sunglasses also may be used to maintain night vision after prolonged periods of exposure to bright sunlight. Two or 3 hours of sun exposure can delay by up to several hours the time needed to reach the night vision threshold.9,10 Longer exposures over several days may result in larger threshold elevations. Sunglasses with transmittances of less than approximately 15% preserve night vision, whereas sunglasses with transmittances greater than approximately 35% do not.11 Whenever night vision is critical, such as for driving or flying, dark sunglasses should be worn outdoors during the day to maintain optimal visual performance at night.
Sunglasses or any tinted lens with a transmittance less than about 80% should not be used for driving at night. Although tinted lenses solve the problem of headlight glare by absorbing light from headlights and decreasing the visibility of reflections, the lenses greatly decrease the visibility of other objects in the field of view (e.g., a pedestrian).12,13 When a patient reports problems with headlight glare while wearing spectacles, the best solution may be an antireflective coating. This coating decreases the visibility of multiple reflections from the lens surfaces that contribute to glare. The cause of much headlight glare is light scattered within the eye. This problem becomes worse with age. There is no good solution to the problem, and older patients must usually modify their habits so that they are not forced to drive at night.
Sunglass Lens Color
The color or hue of a sunglass lens depends on the wavelengths it absorbs and transmits. For example, a lens that blocks the red and blue ends of the spectrum by necessity transmits more of the remainder of the spectrum, resulting in a green color. Sunglass color should be chosen to avoid compromising the visibility of traffic signals and adversely affecting color vision. The ANSI Z80.3-2001 nonprescription sunglass standard provides recommendations for the transmittance of a lens as it relates to traffic signal visibility or recognition.1 Neutral gray lenses are usually best because their transmittance is relatively constant across the visible spectrum (Fig. 5). Consequently, gray is by far the most commonly prescribed sunglass color.
![]() Figure 5. Transmittance curves for typical glass, CR-39 plastic, and polycarbonate gray sunglass tints. |
Brown and green are other common colors for sunglass lenses. Brown lenses, which selectively absorb the blue end of the visible spectrum (Fig. 6), tend to increase the subjective impression of contrast by darkening the blue sky relative to foreground objects by decreasing some of the effects of blue haze. This effect is pleasing to many users. These lenses may also be beneficial for certain types of color deficiencies. A patient with protanomaly or protanopia might benefit from a brown lens rather than a gray lens because of the relatively high transmittance of the brown lens in the red end of the spectrum, where the patient is least sensitive. The color vision of deuteranomalous or deuteranopic patients may be degraded by such lenses because a brown tint attenuates green radiation. Green lenses (Fig. 7) have maximum transmittance in the green where the human eye is most sensitive (Fig. 1), while absorbing red and blue light. However, a green tint should not be used for a patient with a color deficiency because it narrows the visual spectrum.
![]() Figure 6. Transmittance curves for typical glass, CR-39 plastic, and polycarbonate brown sunglass tints. |
![]() Figure 7. Transmittance curves for typical glass, CR-39 plastic, and polycarbonate green sunglass tints. |
Choosing a sunglass color for a patient with a color deficiency requires care. The color vision of these patients may benefit from one color of tint and be made worse by another, so it is important to identify the exact color vision deficit that is present. This task is not simple because most color vision tests do not classify deficiencies by type but only indicate whether a color deficiency is present. Exact diagnosis or identification usually requires an anomaloscope, the Farnsworth-Munsell 100 hue test, or the Panel D-15 test,14,15,16 color vision tests that are not available in most clinical practices. A gray sunglass lens is generally the best option for all patients.
Protection from Harmful Solar Radiation
The electromagnetic spectrum includes a large range of wavelengths, from the extremely short wavelength gamma rays and x-rays to the extremely long wavelength radio waves and microwaves. Visible light is just a small portion of this spectrum, extending from about 380 to 780 nm, with UV radiation adjacent on the short wavelength side and IR radiation adjacent on the long wavelength side. The energy of an individual photon of electromagnetic radiation is given by:17

where h is Planck’s constant, ν is the frequency of the radiation, c is the velocity of light, and λ is the wavelength. Radiation of almost any wavelength has the potential to cause damage to the eye, but solar UV radiation has been of most concern because UV photons have the shortest wavelengths and, therefore, the highest energy of all solar photons reaching the earth’s surface. Industrial or non-natural sources of UV radiation are also of concern, and these include mercury vapor lamps, lasers, and welding arcs. There is no evidence that scattered and reflected IR radiation in sunlight is damaging, although industrial sources of IR radiation may be a cause of eye problems. The effects of everyday exposure to visible (blue) light from the sun are more controversial, but direct viewing of the sun at its zenith can damage the retina after about 90 seconds of exposure.18
The Sun as a Radiation Source
The amount of solar radiation that reaches the surface of the earth varies with many factors, including time of day, latitude, longitude, altitude, cloud cover, and the amount of ozone, water vapor, and dust in the atmosphere. Solar irradiance as a function of wavelength at the earth’s surface can be calculated from the irradiance measured outside the atmosphere, with allowances for these factors.19 An example is shown in Figure 8. The shortest solar wavelength that reaches the surface of the earth is about 288 nm. Shorter UV wavelengths are absorbed by molecular and atomic nitrogen and oxygen in the atmosphere and by the ozone layer of the earth.20 UV radiation from 290 to 380 nm is significantly transmitted, with transmittance increasing as wavelength increases, and the visible spectrum from 380 to 780 nm is also highly transmitted. Near IR radiation (or IRA) from 780 to approximately 1,400 nm reaches the surface of the earth in large amounts, but longer wavelengths (1,400 nm to 1 mm) are heavily absorbed by molecules in the atmosphere. IR radiation is responsible for the feeling of heat when the skin is exposed to the sun. The effects of UV radiation on the skin include tanning, sunburn, aging, and skin cancers.21
Effects of Ultraviolet Radiation on the Eye
Cornea and Conjunctiva
The cornea absorbs all UV radiation below approximately 300 nm, with absorption decreasing (transmittance increasing) rapidly above this wavelength (Fig. 9A). Absorbed radiation has the potential to cause damage, and laboratory studies of both animals and humans have shown that the action spectrum for corneal damage from UV radiation extends from approximately 220 to 310 nm.22 The UVB wavelength band, extending from 290 to 315 nm, has the shortest wavelengths and therefore the highest energies of all radiation that reaches the surface of the earth from the sun. UVB radiation is strongly reflected by snow, greatly increasing the levels reaching the eye, even with a relatively weak winter sun.23 Damage to the anterior eye caused by a few hours exposure to high levels of UVB radiation is termed photokeratitis, photophthalmia, actinic keratitis, photokeratoconjunctivitis, or snowblindness.23,24 A patient suffering from photokeratitis may have pain, photophobia, lacrimation, blepharospasm, and an ocular foreign body sensation or gritty feeling.22,25 The skin of the eyelids and face are reddened (sunburn), and fluorescein staining of the cornea shows focal loss of epithelial cells. There is commonly a latent period, with signs and symptoms typically appearing 6 to 12 hours after exposure. Symptoms usually disappear within 48 hours, and permanent damage is rare.
Photokeratitis is not commonly found with other exposures to solar UV radiation because most other surfaces (e.g., grass, sand, concrete) are poor reflectors of UV. Water is a poor UV reflector, but its relatively smooth surface allows it to reflect UV from the entire sky, providing a greater UV exposure to the eye.18 UV levels from overhead can be high, but the eyebrows, eyelids, and eyelashes provide considerable protection. Overhead UV radiation, because of its high angle of incidence, tends to reflect from the cornea rather than be absorbed. People also tend not to look at the bright overhead sky on sunny days. As a result, little direct solar UV radiation reaches the cornea from the hours of 9:00 am to 3:00 pm when UV radiation levels are highest.26 The UV exposure of the cornea is at most about 5% of the UV falling on top of the head.27 Squinting further decreases ocular exposure by decreasing the vertical field of view.28 However, on a cloudy or overcast day UV exposure to the eye may actually be increased because of decreased squinting and because the amount of UV scattered near the horizon may be increased.27 A prone sunbather could conceivably be a victim of photokeratitis if the sky is viewed for extended periods of time, but the conditions under which this could occur would be unusual.29 Reflection from sand can cause photokeratitis, but only with six to eight hours of exposure.26 Sunbathers should wear eye protection.
When photokeratitis is associated with exposure to a welding arc, the condition is often termed welder’s flash.23 Electric welding arcs emit large amounts of visible, IR, and UV radiation, including UVA (315 to 380 nm), UVB, and UVC wavelengths. The UVC extends from 100 to 290 nm and is not part of the solar spectrum at the earth’s surface, but it contributes to the corneal damage by a welding arc. Welders must be protected from this UV, IR, and visible radiation, and the ANSI Z87.1-2003 standard for industrial eye protection provides transmittance requirements for welding filters.30 Bystanders or assistants who are not wearing proper eye protection may also suffer eye injury from welding arc radiation. As with snowblindness, the signs and symptoms of welder’s flash typically appear 6 to 12 hours after exposure and disappear within 48 hours.
Pterygia, climatic droplet keratopathy, and pingueculae have all been associated with long-term solar UV exposure.31,32,33,34,35,36,37,38,39 Other factors, such as dietary deficiency, low humidity, and chronic eye irritation from wind-blown dust, ice and snow may be important in the development of all of these conditions.
Lens
The crystalline lens absorbs most UV radiation below 400 nm, acting as a filter to protect the retina from UV radiation that is transmitted by the cornea (Fig. 9B). Radiation that reaches the lens has been implicated in the development of cataracts. Animal studies indicate that acute exposure to UV radiation from 295 to 320 nm (primarily the UVB) causes lens opacities, but UV radiation of longer wavelengths (the UVA) does not appear to cause damage, even at high exposure levels.40 Many epidemiologic studies41,42,43,44,45,46,47,48 have shown that chronic UVB radiation exposure is associated with the development of cortical cataracts but not nuclear cataracts or posterior subcapsular cataracts, and that long-term UVA exposure does not seem to be associated with the development of cataracts. However, two recent studies have found an association between UVB and nuclear cataract and suggest that exposure to UVB at a relatively young age may be the important variable.49,50
The potential depletion of atmospheric ozone by chlorofluorocarbons may have an impact on the prevalence of cataracts that are associated with UV radiation. As the ozone layer thins, UV radiation levels are expected to increase, and the increase is expected to be greatest for UVB radiation.51 West et al.52 estimate that a 5 to 20% depletion of ozone will result in an increase in cataract prevalence of 1.3 to 6.9%.
Retina
UV radiation reaches the retina of the intact (phakic) eye in small amounts, primarily in a band of wavelengths transmitted by the lens that extends from approximately 300 to 340 nm, with a peak at 320 nm (Fig. 9B). Transmittance at 320 nm is approximately 8% for a young eye, but almost no UV is transmitted by age 22.53 Photons of wavelength 300 to 340 nm are of high energy, and animal studies suggest that this radiation has a low threshold for damaging the retina.54,55 The development of age-related macular degeneration (AMD) could be related to exposure to UV radiation, but epidemiological studies have not consistently demonstrated an association.56 A more likely association may be between visible light and retinal damage because much higher levels of visible light reach the retina.
When the crystalline lens is removed, large amounts of both UVB and UVA radiation reach the retina. Patient reports of erythropsia after cataract surgery are probably related to this increased UV exposure,57,58,59 as is a selective loss of blue cone sensitivity after a number of years of exposure.60 UV wavelengths as short as 365 nm, and possibly shorter, can be detected by the aphakic patient and appear violet in color.61,62 Because of the chromatic aberration of the eye, this radiation is out of focus and decreases image contrast. All intraocular lenses should provide protection against UV radiation, and all aphakic patients without UV-protective intraocular lenses should have UV protection in their spectacles, contact lenses, and sunglasses.
Medications and Exposure to Ultraviolet Radiation
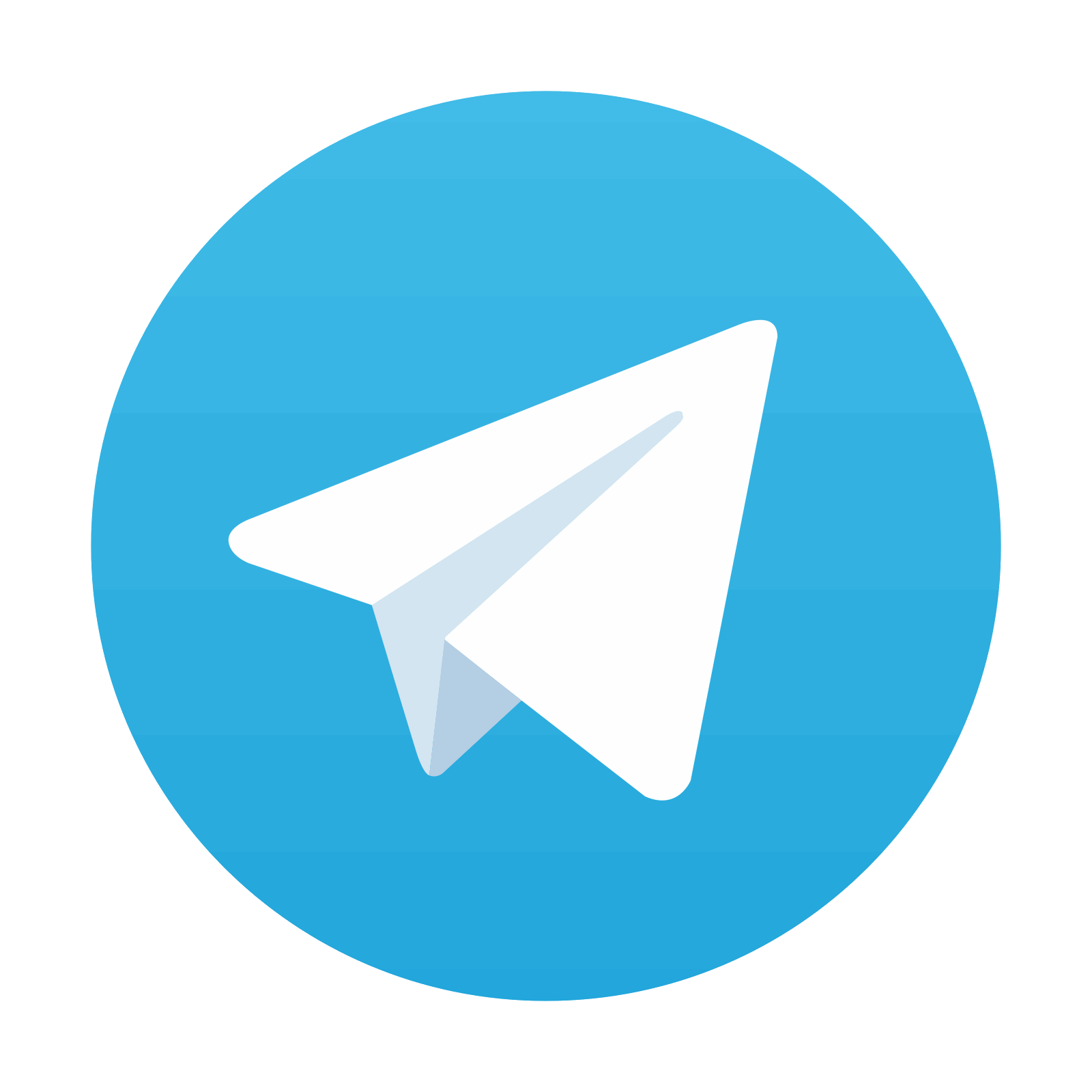
Stay updated, free articles. Join our Telegram channel

Full access? Get Clinical Tree
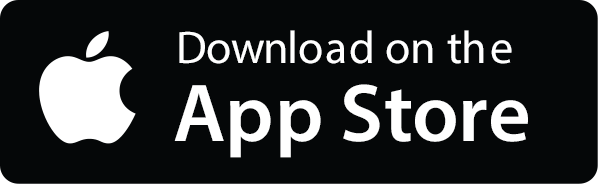
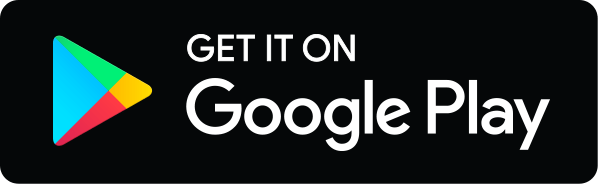
