(1)
Department of Ophthalmology and Visual Sciences, University of Iowa Hospitals and Clinics, Iowa City, IA, USA
For over 150 years, the characteristic ophthalmoscopic findings of central retinal artery occlusion (CRAO) have been well established; however, there is much confusion in the literature about how long it takes for CRAO to produce irreversible retinal damage. This latter information is of paramount importance in its management. In the literature, this length of time is based on anecdotal clinical case reports and experimental studies in the rat, cat, rabbit, and squirrel monkey, as summarized elsewhere [1].
Experimental Studies
A large number of experimental studies have been published on acute retinal ischemia. Most had study designs, which differ fundamentally from clinically seen CRAO in the human. The fundamental prerequisite for any such experimental study is to simulate the type of acute retinal ischemia produced by CRAO in humans. In order to do that, it is imperative that it fulfill the following two basic requirements: (1) the experimental animal must have a central retinal artery (CRA) with a distribution and vascular pattern identical to that seen in humans and (2) the experimental method used must cause CRAO only and not panretinal or panocular ischemia. The relevance of a study of acute retinal ischemia that does not fulfill these two basic requirements is open to serious objections as regards CRAO in patients, although it may be helpful in providing information about other issues on generalized retinal ischemia. The following is a brief summary of the designs of various studies on acute retinal ischemia reported in the literature:
1.
Retinal Vascular Pattern in Experimental Animals: In rabbits, most of the retina is avascular and supplied by the choroid, the CRA supplying only two very narrow horizontal bands of retina nasal and temporal to the optic disc. Hence studies on CRAO in rabbits are of little clinical relevance to man. In the cat, there is no CRA and the entire thickness of the retina is supplied by posterior ciliary artery (PCA) circulation; in man and primates, by contrast, the CRA supplies only the inner layers of the retina, with the outer retina supplied entirely by the PCA circulation. Therefore, findings from acute retinal ischemia in rabbit and cat are not relevant to CRAO in man. Primates have a retinal vascular pattern identical to that seen in man.
Moreover, the retinal survival time in CRAO depends upon the availability of glycogen to maintain anaerobic glycolysis [2–5]. The glycogen content of the retina varies among different species – most abundant in the rabbit, least in the rat and mouse, and between these two extremes in man and the monkey [6]; that must influence the retinal tolerance time to acute ischemia.
2.
Experimental Methods Used to Produce Acute Retinal Ischemia: The following are the main methods that have been used to produce acute retinal ischemia in the reported studies:
(a)
Raising intraocular pressure in the eye to above the systolic arterial blood pressure level: This is the most commonly used method. Histopathological studies have shown that this method produces global ocular ischemia [5, 7–10]. There is no clinical condition in humans with similar acute global ocular ischemia.
(b)
Ligature around the optic nerve behind the eyeball: A review of studies using this method shows that the ligature occludes not only the CRA but also the PCAs lying by the side of the optic nerve, as well as the optic nerve. PCA circulation is the main source of blood supply to the outer retina and in rabbits to most of the retina. Therefore, occlusion of CRA and PCAs results in panretinal ischemia. In contrast to that, the CRAO in man produces acute ischemia of only the inner retinal layers (i.e., nerve fiber, ganglion cell, and inner plexiform layers and inner part of the inner nuclear layer). Therefore, the findings of a model with occlusion of the CRA and PCAs are of little scientific relevance to the clinical situation of CRAO in man.
To find out how long it takes for CRAO to produce irreversible retinal damage, initially I conducted a study in young rhesus monkeys [1, 15, 16]. The findings of that study showed that CRAO of up to 97 min resulted in no irreversible damage. That contradicted the conventional thinking, based on findings in the brain, that irreversible ischemic damage in the retina occurred much sooner since retina is similar to brain. Critics felt that the retina survived as long as it did in that study because the monkeys were young, normal, and healthy and that typical CRAO patients, who are generally old, atherosclerotic, and hypertensive, would probably have a much shorter retinal ischemic tolerance time. To investigate whether that was the case, I developed a cohort of rhesus monkeys, which mimicked CRAO patients – by producing experimental atherosclerosis and chronic arterial hypertension, lasting for many years. By the time I undertook the second experimental study on CRAO [17], the monkeys were indeed old, atherosclerotic, and hypertensive (similar to the CRAO patients).
In both studies, CRAO was produced by lateral orbitotomy in one eye of each monkey, by clamping the CRA for variable lengths of time at its site of entry into the dural sheath of the optic nerve (Fig. 11.1). In all eyes fundus changes were evaluated by ophthalmoscopy and stereoscopic color fundus photography, retinal circulation by fluorescein fundus angiography, and retinal function by recording of electroretinography (ERG) and visual evoked potential (VEP). All these procedures were performed before and during CRA clamping and soon after unclamping the CRA. The same studies were performed serially during follow-up. At the end of follow-up, the eyes were enucleated for histopathology to determine the extent of retinal damage.
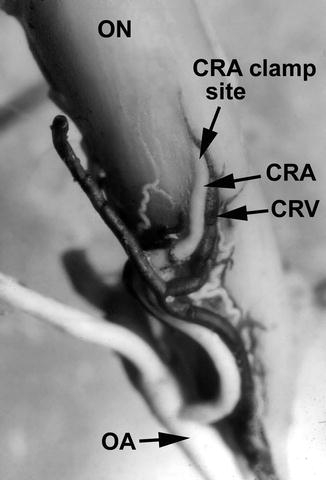
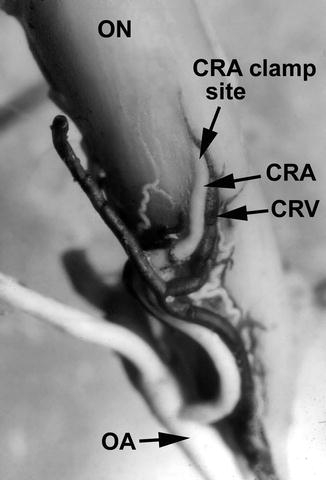
Fig. 11.1
Inferior surface of the intraorbital part of the optic nerve showing central retinal vessels and their site of penetration into the sheath of the optic nerve in the rhesus monkey (Reproduced from Hayreh [21]) Abbreviations: CRA central retinal artery, CRV central retinal vein, OA ophthalmic artery, ON optic nerve
My First Experimental Study in Young, Healthy Rhesus Monkeys [1, 15, 16]
This was conducted in 63 eyes of young rhesus monkeys. In this study, 89 % of the eyes showed a variable amount of residual retinal circulation on fluorescein fundus angiography during CRA clamping, starting anywhere from <4 to >120 (38 ± 56) seconds after the injection of intravenous fluorescein, and the retinal arterioles filled to variable extent in these eyes (see residual circulation below) [16]. There was no definite relationship between the time it took for the retinal arterioles to start filling (after the intravenous injection of fluorescein) and the time that elapsed before the complete/partial filling was finished. There was no retinal venous filling except in six eyes, indicating that when there was filling of the retinal vascular bed, it was mostly minimal while the clamp was on the CRA. After unclamping the CRA, in all the eyes, the retinal circulation was restored to its normal preclamping state. On ophthalmoscopy, at first examination 7 min after CRA clamping, the posterior retina already showed a mild degree of opacity with a pale optic disc. During CRAO, electroretinography showed absent b-wave and normal a-wave, and VEP in the involved eye was absent [1, 15]. On unclamping the CRA, ERG recovered to normal level in all eyes with CRAO up to 135 min. VEP recovered to normal with CRAO of 97–98 min. These studies revealed that ERG is a poor predictor of visual recovery following retinal ischemia because it gives no information about the functional integrity of the retinal ganglion cells and nerve fibers. The study showed that presence of residual retinal circulation, when the CRA was clamped, did not exercise any protective action against ischemic retinal damage. Duration of the ischemia was the principal factor determining severity of damage. Thus, this study showed that CRAO for up to 97 min produced no significant permanent neural damage, but CRAO for 105 min or longer produced irreversible permanent neural damage.
My Second Experimental Study in Old, Atherosclerotic, and Hypertensive Rhesus Monkeys [17]
In this study, transient CRA clamping, varying from 97 to 240 min, was done in 38 eyes. Stereoscopic color fundus photography and fluorescein fundus angiography, ERG and VEP before clamping CRA, during clamping, and immediately after unclamping, were performed. The same studies were performed serially during follow-up. At the end of follow-up, the eyes were enucleated for histopathology to determine the extent of retinal damage. Findings of these tests are given in detail elsewhere [17].
Fluorescein Fundus Angiographic Findings [17]
It showed almost invariably some slow filling of the retinal circulation when CRA was clamped (see residual circulation below). Angiography before CRA clamping showed that the average normal time interval between the start of choroidal filling and the start of CRA filling was about 0.2 s and CRA filled completely in about 2 s. After clamping the CRA, the corresponding median (25th–75th percentile) times were 10 (4–23) and 131 (32–248) seconds, respectively, with no significant difference in these timings for various durations of CRAO. The extent of retinal damage following CRAO, as detected by ERG and histology, was correlated with the amount of this residual retinal circulation to find out if that influenced the retinal damage.
Electrophysiological Findings [17]
To determine the function of the retina in a study related to retinal tolerance to acute ischemia is most important. Electrophysiological study provides that vital information. Therefore, I would like to discuss that in more detail.
In our study [17], a “negative ERG” appeared during CRA clamping. With removal of the CRA clamp, there was b-wave recovery, with differential rates of recovery of ERG – eyes with shorter CRAO recovered sooner than those with longer occlusion. On removal of the clamp, recovery was seen in scotopic 24 db b-wave, photopic 0 db single flash b-wave and 30 Hz flicker, with the b/a ratio of the combined rod and cone response and selective rod response showing statistically significant differences among the shorter and longer periods of CRAO. A delayed normalization of the depressed b/a ratio immediately after CRA reperfusion may indicate high-grade ischemic damage. At the final follow-up test session, no clear-cut derangement of any ERG parameter was seen for any group, with subtotal b-wave amplitude recovery for all groups. Longer CRAO produced incomplete VEP recovery.
Results of our study showed that more severe ischemic damage with longer durations of CRA clamping resulted in lesser likelihood of scotopic 24 db b-wave amplitude recovery. Indeed, all durations of CRA clamping in our study extinguished this measure during the period of CRA clamping, and in the group with CRAO of ≥180 min 5 of 6 eyes did not recover in the immediate reperfusion period, with 7 of 29 eyes of lesser duration CRA clamping recovering. This is not to imply that ischemia does not affect the cone system, as a significant reduction in photopic 0 db 30 Hz flicker, and photopic 0 db b-wave amplitudes was seen for all durations of CRA during CRA clamping. Improvements in these amplitude measures of cone function were noted with immediate CRA reperfusion, and further improvement in amplitude in the weeks following.
The prolongation of the scotopic 0 db a-wave implicit times with CRA clamping was reversed and shortened with CRA reperfusion, reverting fastest to baseline in eyes with CRAO for 97 min versus the longer durations of CRAO. The lengthening of implicit times with ischemia and normalization by shortening with CRA reperfusion seems to be a robust finding for other measures as well. Recovery of implicit times for all the ERG measures at final post-CRA occlusion weeks later suggests that perhaps the prolongation of implicit times is an indicator of ischemia, but not cell death.
It is well known that ERG, while a good indicator of the functional integrity of the photoreceptors and bipolar cells, does not give us any information about the functional status of the ganglion cells. Since ganglion cells are more susceptible to ischemic damage than other neural elements in the inner retina, VEP measuring the function of the ganglion cells may actually provide better information about the function of inner retinal ischemia and visual outcome. When the CRA was clamped, VEP was always a flat line without any noise. There was a significant difference in time to VEP recovery among the four CRAO duration groups (97, 105–120, 150–165 and ≥180 min). In our study the VEP did show that longer periods of CRAO, specifically the 150–165 min and the ≥180 min groups, did not recover fully, at least by 30–35 days after occlusion. Whether they would have recovered fully if observed for a longer period of time is not answerable in this experiment, although our clinical experience of studying 260 eyes with CRAO suggests that there is little recovery of function on a long-term follow-up [22]. It is difficult to speculate whether all or a subset of ganglion cells were selectively and permanently destroyed by the longer periods of CRAO, or whether all or a subset of them are slower to recover. In this connection, it is relevant to point out that retinal tolerance time to ischemia is not an all-or-none phenomenon. Different cells within the retina have different susceptibility to ischemic damage, with the ganglion cells being the most sensitive. However, even among the ganglion cells, there is variation; those in the central, thicker macular region, with 3–5 ganglion cell layers, appear to be more susceptible than those elsewhere in the retina. In our studies on monkeys and patients with CRAO having marked ischemic retinal edema around the cherry-red spot in the central macular region, fluorescein fundus angiography often showed absence of filling of retinal capillaries in that region on restoration of CRA circulation (Figs. 11.2, 11.3, and 11.4) [22, 23]. This “no-reflow phenomenon” [1] is due to compression and occlusion of the capillaries by the marked swelling of the thick ganglion cell layer in that region. Such patients are left with a large absolute central scotoma (Fig. 11.5) because of loss of ganglion cells in that area, with normal peripheral fields.
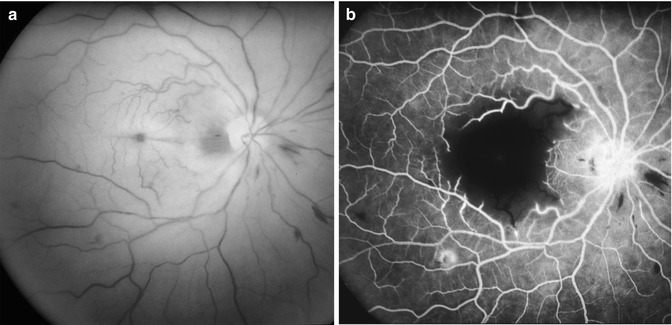
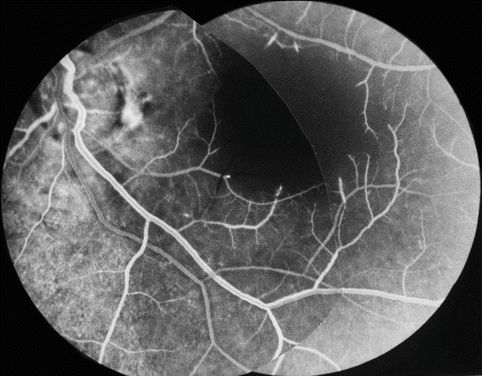
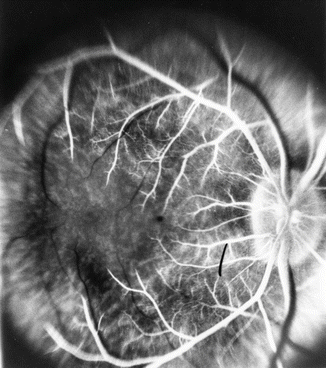
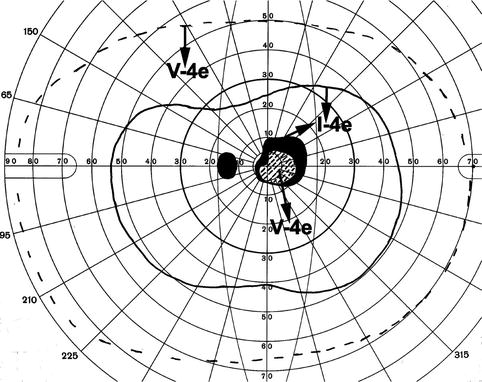
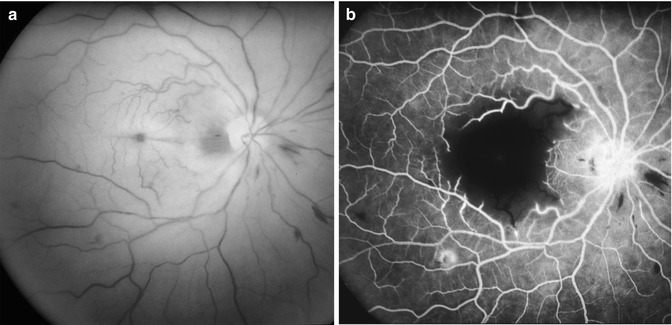
Fig. 11.2
Fundus photograph (a) and fluorescein fundus angiograms (b) of right eye of a patient with CRAO, 10 days after its development, with bare hand motion vision, cherry-red spot with marked retinal swelling in the macular region, and a small cilioretinal artery sparing area temporal to the optic disc. (b) Angiogram 120 s after injection of the dye, showing complete filling of the retinal vascular bed except in the central macular region with marked retinal swelling (Reproduced from Hayreh and Zimmerman [22])
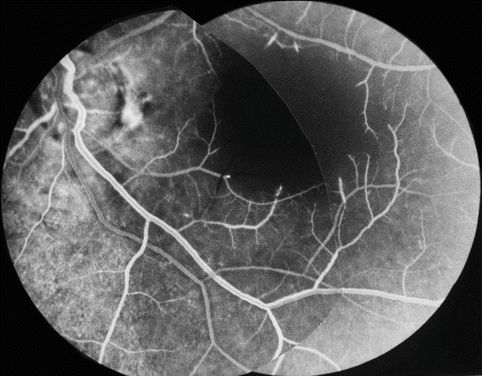
Fig. 11.3
Fluorescein fundus angiogram of left eye of a patient with CRAO 2 weeks after onset, showing almost normal filling of the retinal circulation but no filling of retinal capillaries in the macular region associated with macular edema and cherry-red spot (Reproduced from Hayreh [23])
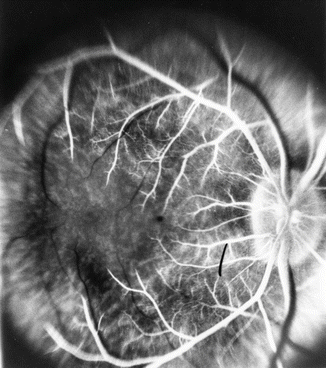
Fig. 11.4
Fluorescein angiograms of right eye with CRAO in rhesus monkey with no filling of retinal capillaries in the macular region associated with macular edema and cherry-red spot
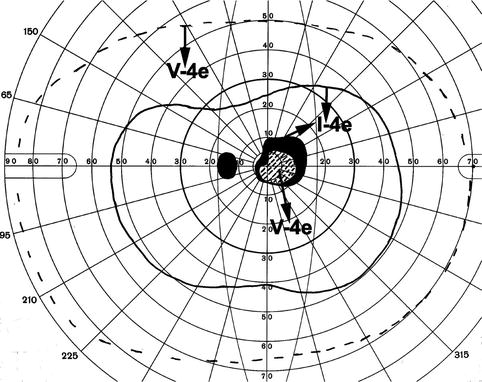
Fig. 11.5
Visual fields of left eye, plotted with a Goldmann perimeter 2 months after the development of transient non-arteritic CRAO. It shows an absolute central scotoma and slightly constricted peripheral visual field with I-4e but normal with V-4e. There was no filling of retinal capillaries in the macular region associated with macular edema and cherry-red spot (Reproduced from Hayreh and Zimmerman [22])
In this study [17], the ERG did not show a permanent extinction of waveforms, nor a massive reduction in amplitudes as might be expected with catastrophic retinal destruction from high-grade ischemia. The various ERG parameters demonstrated different rates of recovery following ischemia, with implicit time consistently returning to baseline levels at the final post-CRA occlusion session, while amplitude measures of both rod and cone systems tended toward lower final values. The same question can be posed regarding true cell death without prospect of recovery versus a prolonged rate of recovery seen as subtotal at the termination of the experiment.
In conclusion, the ERG appears to be a robust and reliable indicator of inner retinal ischemia, with the scotopic 0 db b/a amplitude ratio, a sensitive indicator of ongoing ischemia. The selective rod response, or scotopic 24 db b-wave, was extinguished for all durations of CRA clamping we used, and the photopic 0 db single flash b-wave and 30 hz flicker were also depressed during CRA clamping. With CRA reperfusion on removal of clamp, recovery was seen in these measures, with the b/a ratio of the combined rod and cone response and selective rod response, showing statistically significant differences in the immediate reperfusion among the shorter and longer periods of CRAO. No clear-cut derangement of any ERG parameter at the final test session weeks after removal of CRA clamp could discriminate between the four CRAO-duration groups (97, 105–120, 150–165 and ≥180 min). A delayed normalization of the depressed b/a ratio with CRA reperfusion might indicate high-grade ischemic damage ultimately reflected in ganglion cell death seen histologically. The clinical ERG does not reflect ganglion cell activity, but in the face of a normal ERG, the abnormal VEP with histological evidence of ganglion cell loss in the 150–165 min and ≥180 groups is evidence pointing to a longer retinal tolerance time to ischemia in elderly, hypertensive, atherosclerotic primates [17] than the previously reported 105 min using young, healthy primates [1].
Histopathological Findings
In this study [17], on histopathology, the damage was most marked in the macular retina. The eyes with CRAO for 97 min showed practically no damage, but the duration of CRAO was found to be significantly associated with the amount of damage in the ganglion cell layer (p = 0.009) and inner nuclear layer (p = 0.017). Outer nuclear and plexiform layers and photoreceptors showed no damage at all with CRAO. There was no significant association of the ERG measures and histological changes with any of the residual retinal circulation variables.
Histological studies on selective CRAO in monkeys in the literature deal with changes seen only within the first 24 h after CRAO [11, 12, 14] and showed morphologic changes restricted only to the nerve fiber, ganglion cell, and inner plexiform layers and some of the inner nuclear layer; however, none of these studies is comparable to our study, evaluating changes several weeks after transient CRAO for various periods. There are some autopsy studies in patients with CRAO. For example, Dahrling [24] found diffuse edema of inner retinal layers in one eye 60 h after CRAO, as well as severe reduction of ganglion cells – most striking in the perimacular region, with deeper layers from the inner nuclear outward not affected. Zimmerman [25] in an eye 62 h after CRAO found inner retinal layers extremely edematous, while the outer retinal layers appeared quite well preserved.
In our study [17], the optic nerve showed no abnormality in the eyes with CRAO of 97 min. With CRAO for 105–120, 150–165, and ≥180 min, the optic nerve showed no apparent morphologic abnormality in 86, 81, and 67 %, respectively. Because the severity of optic nerve damage is related to the duration of follow-up after CRAO – the longer the duration, the greater the damage – it is possible that longer follow-up might have shown more abnormal nerves. There is no comparable study in the literature of long-term changes in the optic nerve in CRAO.
Morphometrical Findings
In our study, retinal nerve fiber layer damage and optic disc changes were also assessed by comparing morphometric evaluation of the color fundus photographs taken before CRAO and at the end of the study [26]. There was a significant correlation between duration of CRAO and decreased visibility of retinal nerve fiber layer (p = 0.018) and increasing optic disc pallor (p = 0.014). However, there was a marked interindividual variation between the length of CRAO and degree of increased optic disc pallor and decreased visibility of the retinal nerve fiber layer, even among eyes with similar duration of CRAO. CRAO for 97 min caused no apparent morphometric optic nerve damage; however, CRAO of 105 min but less than 240 min produced a variable degree of damage. CRAO for 240 min produced total or almost total optic nerve atrophy and nerve fiber damage.
The above findings from electrophysiological, histopathological, and morphometric studies showed that the retina of old, atherosclerotic, and hypertensive rhesus monkeys suffered no detectable damage with CRAO of 97 min but suffered progressively more irreversible damage with increase in duration of CRAO. However, there was a marked interindividual variation in the relationship between the length of CRAO and the extent of retinal damage, even among eyes with similar duration of CRAO, for no apparent reason. The study suggests that CRAO lasting for about 240 min results in massive, irreversible retinal damage. These findings are in contrast to my previous study in young, healthy rhesus monkeys which revealed that retinal damage following CRAO is reversible if CRAO lasts for 97–98 min, but the retina suffers irreversible damage after ischemia of 105 min [1, 15, 16]. This new finding in old, atherosclerotic, and hypertensive rhesus monkeys is counterintuitive that the retina in them should survive longer than that of normal, young, healthy monkeys. Naturally, the question arises: why? It could be argued that ketamine hydrochloride, a commonly used anesthetic agent, has been shown as a potent N-methyl D-aspartate (NMDA) antagonist and could attenuate ischemic injury [27]. For sedating monkeys, before inducing general anesthesia with pentobarbitone sodium, I used ketamine hydrochloride in all the monkeys in both studies. Hence, we can rule out that possibility.
Findings of these studies conflict with many of the anecdotal clinical reports and experimental reports in the literature [1]. I saw a patient with CRAO who consulted an ophthalmologist and had his normal vision restored by a massage and paracentesis about 90 min after the onset of the visual loss. The patient was followed for 2½ years with normal visual fields and a visual acuity of 20/20. The study casts serious doubt on the case reports of recovery of vision many hours and even days and weeks after the onset of CRAO.
Both experimental studies [1, 15–17] showed the following three phenomena:
1.
When CRA was clamped, fluorescein fundus angiography almost invariably showed some slow filling of the retinal circulation – residual retinal circulation.
2.
Studies showed that retina tolerated acute ischemia for much longer time than the brain.
3.
As shown above, retinal tolerance to CRAO differed between young and old, atherosclerotic, hypertensive rhesus monkeys.
These three issues are important and need detailed discussion.
Residual Retinal Circulation in CRAO
Almost invariably, filling of a variable amount of the retinal vascular bed on fluorescein fundus angiography is seen soon after the development of CRAO, as shown by my experimental [16–20, 22] and clinical [22, 23] studies. This has led to a very common misconception that in CRAO the occlusion of the CRA is not complete. This misconception requires detailed discussion.
For well over a century, in eyes with CRAO, filling of the retinal arteries on ophthalmoscopy has been described when the patient is first seen within a few days or sometimes even within a few hours after the onset of loss of vision. One remarkable feature seen in my two experimental studies on CRAO is that in spite of complete occlusion of the CRA and massive retinal ischemia (Fig. 11.6), it was not at all uncommon to find on ophthalmoscopy normal-looking retinal vessels [27], misleading an observer to believe that the retinal arteries had a normal or almost normal blood flow. This is a serious drawback in some of the observations reported in the preangiography era. My experimental and clinical studies on CRAO have shown that ophthalmoscopically normal-looking retinal vessels do not rule out a complete CRAO. My experimental studies for the first time also provide information on the resolution pattern of retinal ischemic changes on restoration of retinal circulation [28].
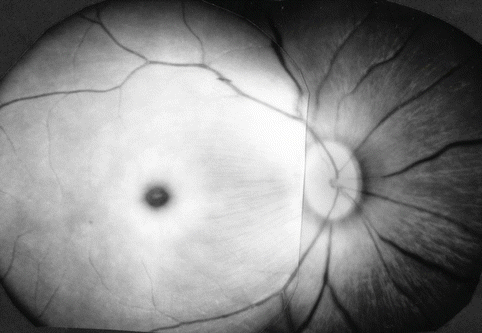
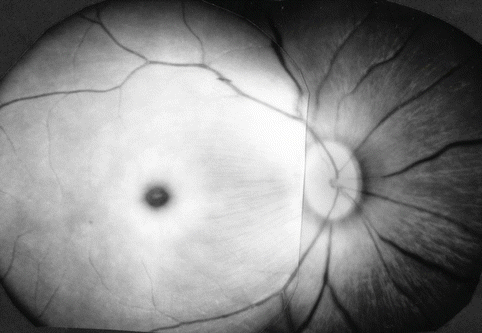
Fig. 11.6
Composite fundus photographs of the right eye of a rhesus monkey 66 min after clamping CRA shows retinal opacity with a well-defined cherry-red spot at the foveola (Reproduced from Hayreh and Weingeist [16])
Findings of fluorescein fundus angiography in my experimental [16–20] and clinical [22, 23] studies on CRAO are highly informative on the subject of residual retinal circulation in CRAO. Angiography in the eyes with CRAO initially almost always shows a variable amount of sluggish filling of the retinal vasculature (Figs. 11.7, 11.8, 11.9, and 11.10), and it is rare to see complete absence of retinal vascular filling in these eyes. This has erroneously led to a widespread misconception that CRA is not completely occluded in CRAO [30–33]. This in turn is used as a justification for the visual improvement claimed with many advocated therapies, even when instituted many hours or even days after the onset of CRAO. For example, Watson [34] very well reflected the prevailing view that if there is even minimal retinal circulation after CRAO, it is possible to obtain a considerable recovery of vision in these eyes.
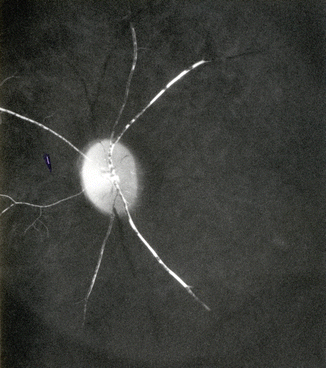
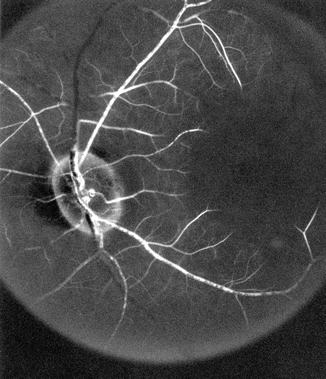
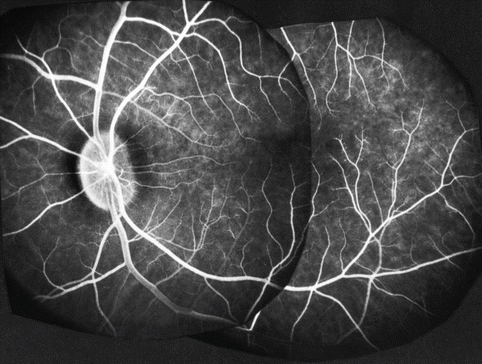
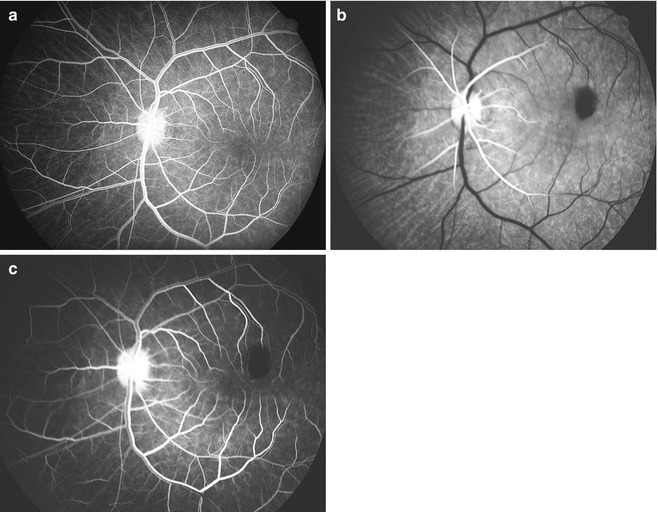
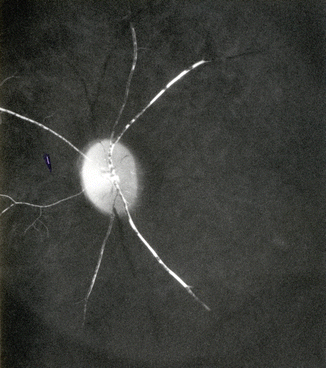
Fig. 11.7
Fluorescein angiogram of the left eye of a rhesus monkey 36 min after clamping CRA shows partial filling of the main retinal arterioles seen posteriorly 408 s after injection of fluorescein (Reproduced from Hayreh and Weingeist [16])
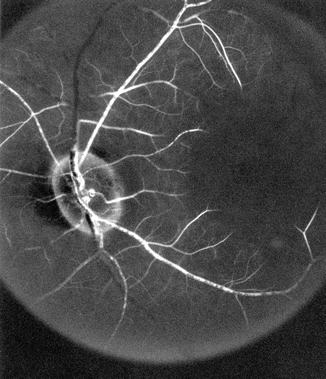
Fig. 11.8
Fluorescein angiogram of the left eye of a rhesus monkey with CRA clamp on, 312 s after the injection of fluorescein, shows slow filling of the retinal arterioles
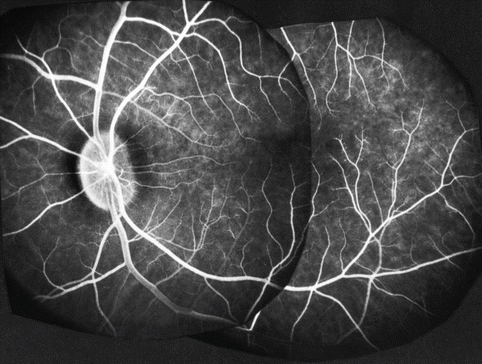
Fig. 11.9
Composite fluorescein angiogram of the left eye of a rhesus monkey 8 min after clamping the CRA shows complete filling of the retinal vascular bed 206 s after injection of dye (Reproduced from Hayreh and Weingeist [16])
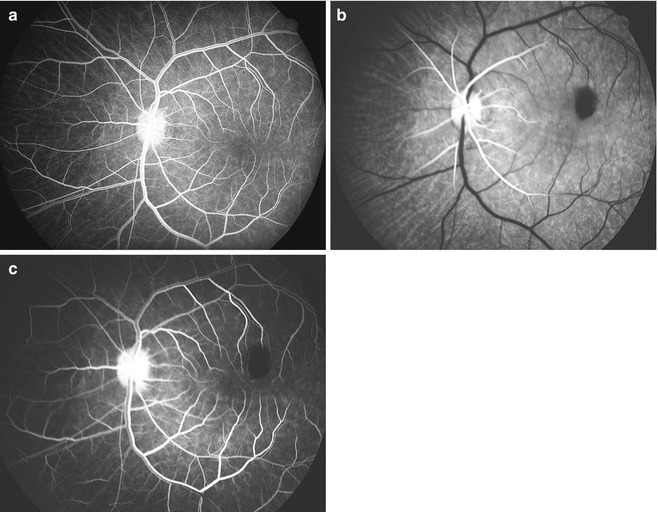
Fig. 11.10
Fluorescein fundus angiograms of the left eye of a rhesus monkey, (a) before and (b, c) immediately after experimental cutting of the central retinal artery at its site of entry into the optic nerve. (a) Before cutting the artery, normal angiogram 14 s after injection of the dye, showing normal retinal vascular filling during the late arteriovenous phase. (b) After cutting the artery, angiogram 14 s after injection of the dye, showing filling of the retinal arteries only up to a short distance away from the disc. (c) After cutting the artery, angiogram 52 s after the injection of the dye, showing retinal vascular filling during the late arteriovenous phase, corresponding to the phase seen in (a) above (Reproduced from Hayreh [29])
As discussed above, in my experimental studies [1, 15–20] in rhesus monkeys, CRAO was produced by either clamping or cutting the CRA where it enters the optic nerve sheath (Fig. 11.1). This is indubitable evidence that there was complete occlusion of the artery; also, these eyes had cherry-red spot and marked retinal infarction clearly indicating massive retinal ischemia (Fig. 11.6). Yet, fluorescein fundus angiography showed some slow filling of the retinal circulation in the vast majority of the eyes, on angiography performed when CRA was clamped or the artery was cut (Fig. 11.7, 11.8, 11.9, and 11.10). I have almost always found identical slow filling of the retinal circulation in my clinical studies on CRAO in 260 eyes, with cherry-red spot and marked retinal infarction [22]. Thus, the presence of retinal circulation on fluorescein angiography in the eyes seen a few hours or days after CRAO does not imply incompleteness of the occlusion.
This naturally raises the issue about the mechanism of filling of retinal circulation in CRAO. That is as follows.
Mechanisms of Filling of Retinal Circulation Soon After CRAO
The following are the two mechanisms:
1.
Collateral Circulation via Cilioretinal Capillary Anastomoses Within the Optic Nerve Head
The optic nerve head has two main sources of blood supply: (a) the superficial layers of the optic nerve head (i.e., the surface of the optic disc) by the CRA circulation and (b) the deeper layers (i.e., prelaminar, lamina cribrosa, and retrolaminar regions) by the PCA circulation [35–37] (Fig. 11.11). The capillary bed in the entire optic nerve is continuous, as shown schematically in Fig. 11.11. In CRAO, this permits blood to flow from the normally filling deeper capillaries of the optic nerve head (supplied by the PCAs) to the adjacent empty capillaries of the superficial layers of the optic disc (supplied by the occluded CRA), and even in the peripapillary retina (Figs. 11.12, 11.13, and 11.14). This blood flow in the capillaries of the surface of the optic disc derived from the deeper ones is one of the sources for the delayed residual retinal circulation seen on fluorescein angiography in these eyes [16–20, 22, 35, 39]. Soon after CRAO, these anastomoses do not seem to contribute to the filling of the CRA to any appreciable extent, but after a few weeks these enlarge sufficiently in some eyes.
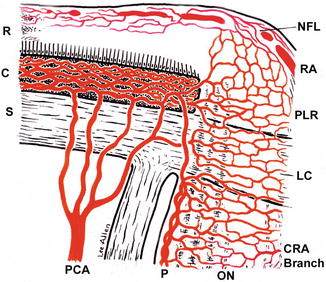
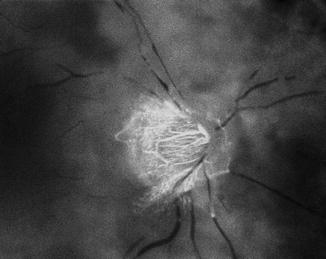
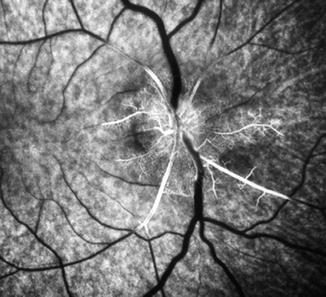
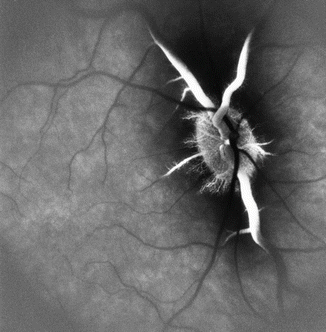
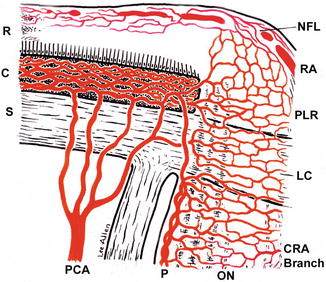
Fig. 11.11
Schematic representation of blood supply of the optic nerve head (Reproduced from Hayreh [38]) Abbreviations: C choroid, CRA central retinal artery, LC lamina cribrosa, NFL nerve fiber layer, ON optic nerve, P pia mater, PCA posterior ciliary artery, PLR prelaminar region, R retina, RA retinal artery, S sclera
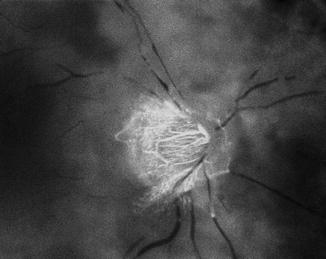
Fig. 11.12
Fluorescein fundus angiogram of right eye of a patient with CRAO, 29 s after injection of the dye, showing normal filling of the choroid and optic disc vessels (supplied by the posterior ciliary artery circulation), but no filling of the retinal vasculature at all as yet. The retinal vessels, particularly the veins, show typical fragmentation of the blood column (the so-called cattle-trucking or boxcarring) (Reproduced from Hayreh [29])
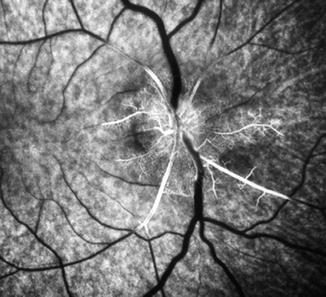
Fig. 11.13
Fluorescein fundus angiogram of the right eye of a rhesus monkey soon after CRAO, showing normal filling of the choroid and optic disc vessels (supplied by the posterior ciliary artery circulation), with slow extension of filling into peripapillary vessels
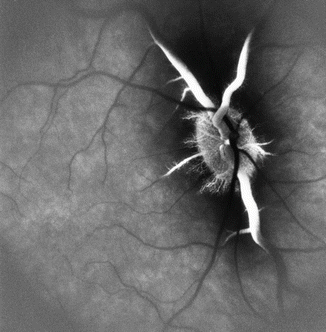
Fig. 11.14
Fluorescein fundus angiogram of the right eye of a rhesus monkey with clamp on the CRA, showing normal filling of the choroid and optic disc vessels (supplied by the posterior ciliary artery circulation), with slow extension of filling into peripapillary veins 177 s after injection of fluorescein
2.
Get Clinical Tree app for offline access
Collateral Circulation via Pial and Intraneural Anastomoses of the CRA
The CRA, at its site of entry into the optic nerve, in the human gives out prominent pial branches in 95 % of eyes, and up to 8 branches while traveling in the optic nerve in 75 % [40, 41] (Fig. 11.15). The great majority of pial branches of the CRA establish very conspicuous anastomoses with pial branches from other orbital arteries (described and illustrated in detail elsewhere [40, 41]) and labeled as “collateral branches” in Fig. 11.15. My anatomical studies on 100 CRAs in the human showed that, contrary to the prevalent impression, the narrowest lumen of the CRA is where the artery pierces the dural sheath of the optic nerve (Fig. 11.16) and NOT at the lamina cribrosa [40, 43]. My clinical studies in CRAO have shown that embolism is the most common cause of CRAO [44, 45]. Therefore, the most likely site for embolic occlusion of the CRA is where it enters the sheath of the optic nerve [39], not, as commonly believed, at the lamina cribrosa. Thus, in both experimental and clinical CRAO, the site of occlusion of CRA is usually outside the optic nerve, so that in spite of complete occlusion of the CRA at its site of entry into the optic nerve dural sheath, all the downstream pial anastomoses of the CRA (Fig. 11.15) are still functioning and fill the intraneural part of the CRA to a variable extent. This was strongly suggested by the fact that the CRA started to fill from its intraneural course (Figs. 11.7, 11.8, 11.9, and 11.10). In my anatomical studies, in some eyes, I ligated the CRA at its site of entry into the optic nerve sheath and injected liquid latex in the ophthalmic artery (Fig. 11.1); in these eyes, the CRA filled in a retrograde fashion, through the various anastomoses of the CRA [40]. In my experimental (Figs. 11.7, 11.8, 11.9, and 11.10) and clinical studies, the speed and extent of filling of the residual retinal circulation varied markedly from eye to eye, depending upon the size of the anastomoses. These observations would strongly suggest that, clinically, (1) when a residual retinal circulation is seen coming from the intraneural part of the CRA (as in Figs. 11.7, 11.8, 11.9, and 11.10), the site of occlusion is probably where the artery pierces the dura mater (i.e., the part of the artery with the narrowest lumen [40, 43]), and (2) in eyes with filling of the capillaries on the surface of the optic disc (Figs. 11.12, 11.13, and 11.14), and not the central retinal artery trunk, the site of occlusion is likely to be in the lamina cribrosa. In eyes with experimental CRAO with filling of capillaries on the surface of the optic disc only (Figs. 11.13 and 11.14), most probably there are no pial or intraneural anastomoses by the CRA.
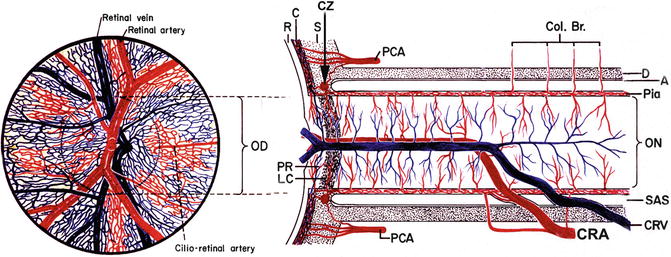
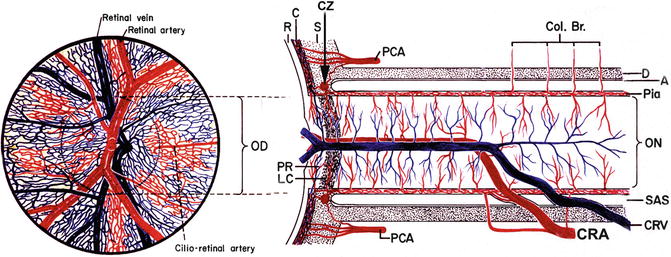
Fig. 11.15
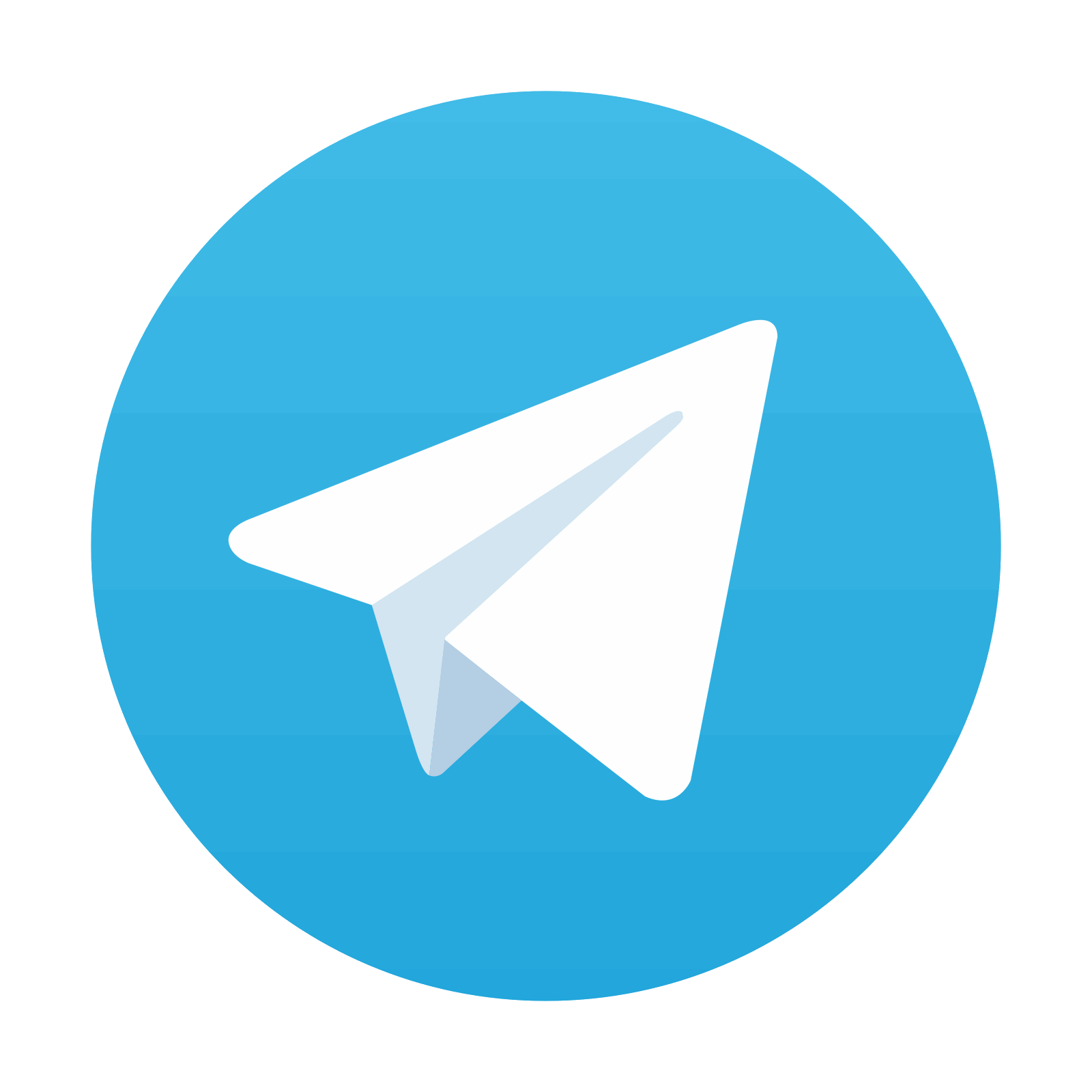
(Right) Schematic representation of blood supply of the optic nerve and of anastomoses established by both the central retinal artery and vein, and (left) ophthalmoscopic view of the vessels on the optic disc and surrounding retina (Modified from Hayreh [42]). Abbreviations: A arachnoid, C choroid, Col. Br. collateral branches arising from the ophthalmic artery or its orbital branches and joining the pial plexus on the optic nerve, CRA central retinal artery, CRV central retinal vein, D dural sheath, LC lamina cribrosa, OD optic disc, ON optic nerve, P pia mater, PCA posterior ciliary artery, PR prelaminar region, R retina, S sclera, SAS subarachnoid space
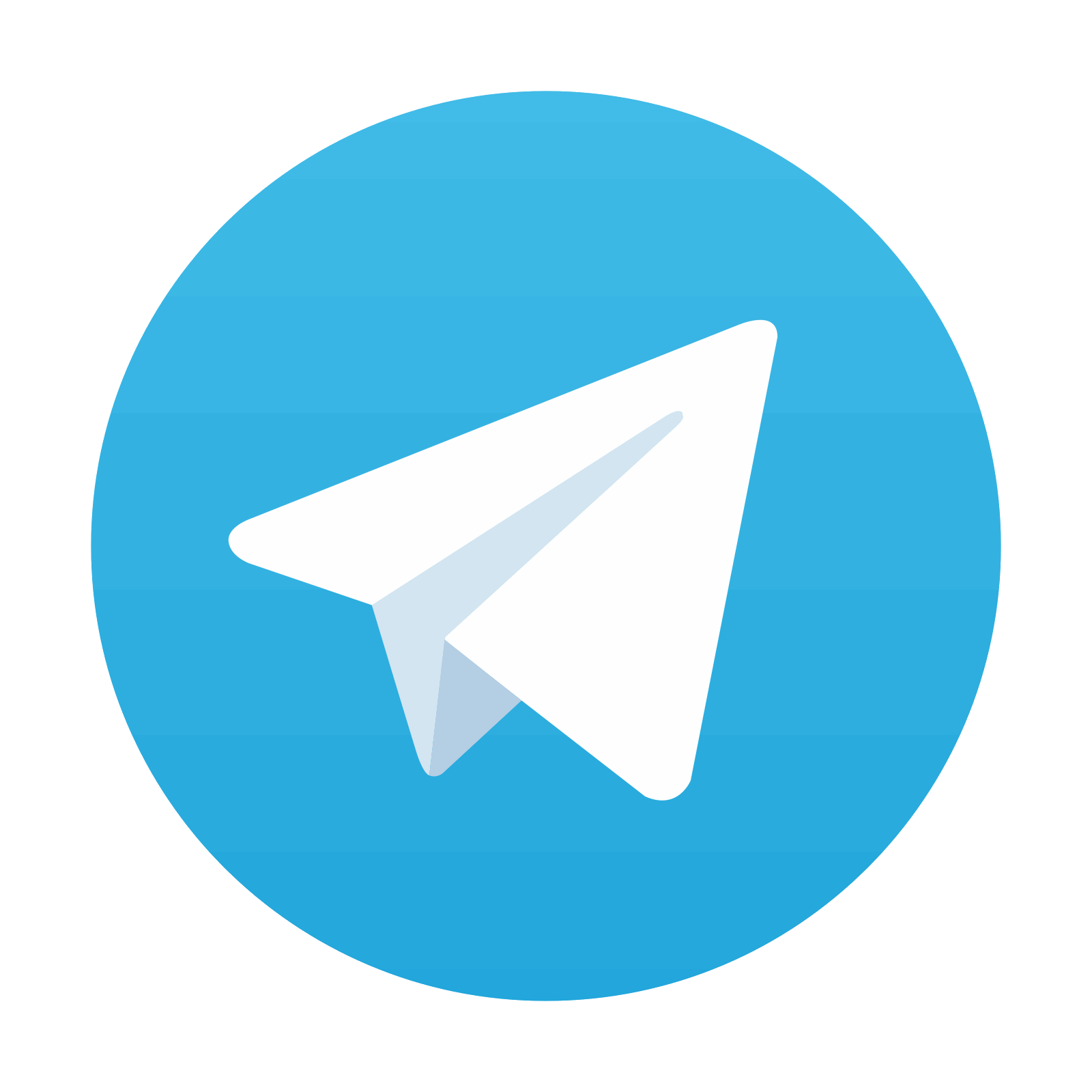
Stay updated, free articles. Join our Telegram channel

Full access? Get Clinical Tree
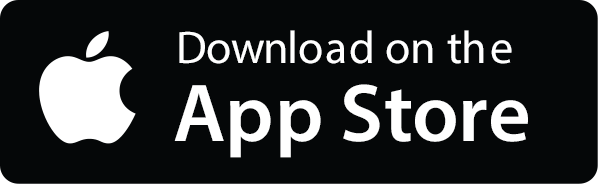
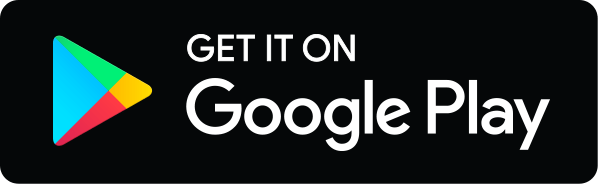
