4 Regenerative Medicine for Inner Ear Disease This chapter is written from a basic science perspective with the following conceptual organization. In vitro studies of both embryonic stem (ES) cells and adult mouse inner ear stem cells are presented to define stem cell characteristics and their ability to form hair cell–like cells under specific culture conditions. A brief summary of inner ear morphogenesis follows as a primer for discussing stem cell transplantation studies in the higher vertebrate inner ear. Mouse stem cell transplant experiments are then presented to characterize stem cell plasticity in the developing avian inner ear. An in utero model system for investigating the therapeutic potential of stem cells for hereditary hearing loss is introduced. Lastly, alternative approaches for generating sensory cells for cell replacement strategies are considered. An important aim of this chapter is to convey the limitless translational research potential in the newly emboldened field of regenerative medicine for inner ear disease. Hearing loss is a global health problem. More than 5% of individuals in industrialized countries are affected by significant hearing impairment, ranging in severity from difficulty with speech comprehension to profound deafness.1 Hearing loss is the leading birth defect in the United States with one child in 1000 born profoundly deaf, and another 2 to 3 out of 1000 born with partial hearing loss involving one or both ears. Remarkably, one in every 10 Americans is affected by acquired hearing impairment, and with the aging of the baby boomer generation, it is estimated that 60 million Americans will suffer from hearing loss by 2030. The malfunction or death of cochlear hair cells, or their innervating spiral ganglion neurons, is a frequent cause of deafness in affected individuals.2 A person with significant residual hearing can benefit from the sound amplification provided by digital hearing aids.3 These devices are programmed to amplify frequencies to which the user is least sensitive to stimulate remaining hair cells to respond. Individuals who have lost most or all of their hair cells may benefit from cochlear prostheses.4,5 The damaged cochlear hair cells are functionally bypassed with the implantation of an electrode array into the scala tympani that excites action potentials in neurons tuned to respond to a narrow frequency range. The ultimate treatment to restore the patency of cochlear function is to replace defective or dead sensory cells with new ones.6 Human ES cells were independently isolated and expanded in culture by the Thomson and Gerhart laboratories.5,7 There is considerable interest in the therapeutic potential of stem cells to treat human disease by cell replacement strategies.8,9 However, ES cells from the mouse were isolated 17 years earlier than human ES cells,10 and no stem cell–based proof of concept therapies for murine inner ear disease have been developed. The recent demonstration of sensory hair cell formation from ES cells and adult mouse inner ear stem cells, however, has refocused research into the feasibility of cell replacement strategies for treating hearing impairment. One hallmark of mammalian embryonic development is the formation of a fluid-filled sphere of cells called the blastocyst. In mice and humans, the blastocyst forms 4 to 6 days postfertilization prior to implantation into the uterine lining. The blastocyst consists of an outer layer of trophectoderm and an inner layer of cells called the inner cell mass (ICM). The trophectoderm does not contribute cells to the embryo proper but does form the outer layer of the placenta called the chorion. The ICM gives rise to the three germ layers of the embryo and is the source of ES cells. Embryonic stem cells meet two defining criteria: the capacity for indefinite self-renewal and pluripotency. ES cells undergoing mitotic division generate two daughters with at least one progeny cell retaining stem cell identity. The other daughter may be a stem cell as well, or it may assume a progenitor cell fate. Stem cell–derived progenitors are not able to renew indefinitely, and they typically differentiate into the component cell lineages of the tissue or organ in which they reside. ES cells are genetically programmed narcissists, taking care to ensure that their lineage never dies out. Progenitors are the equivalent of worker bees, tending to the business of elaborating the organism’s developmental program. ES cells are typically grown in culture media containing the cytokine leukemia inhibitory factor (LIF), which sub-serves the ES cell self-renewal program.11 Embryonic stem cells are pluripotent and can give rise to multiple cell types within the body. Pluripotency was initially demonstrated by transplanting marked ES cells into an organism, allowing it to develop, and then assaying for differentiated cells that bear the ES cell label.12 The data indicate that ES cells can give rise to lineages from the three primary germ layers of the embryo: ectoderm (i.e., neurons), mesoderm (i.e., cardiac cells and connective tissues), and endoderm (i.e., liver). Progenitor cells may also give rise to multiple cell types within the body, but they cannot indefinitely self-renew. Sensory hair cells are mechanoreceptors that transduce the energy of sound and gravity into electrochemical signals interpretable by the brain.13 The human cochlea has 16,000 hair cells that are not replaced after damage or death.14 Cell replacement strategies for inner ear disease require a renewable source of sensory hair cells for the development of novel reparative therapies.6 Remarkably, hair cells were recently generated by the stepwise differentiation of ES cells in vitro.15 ES cells were manipulated in culture to produce hair cells through a three-phase process: embryoid body formation, expansion, and differentiation. The experiments began with three genetically labeled stem cell lines that were maintained either on a mitotically inactivated feeder cell layer, or without feeder cells but with media supplemented with LIF.16 Embryoid bodies, or clumps of undifferentiated cells, were formed in hanging drop cultures with 300 ES cells per media droplet. Expansion of stem cell–derived progenitors was achieved in two steps. First, embryoid bodies were plated onto adhesive culture dishes in media containing epidermal growth factor (EGF) and insulin-like growth factor 1(IGF-1) for 10 days.17,18 Second, the enriched population of progenitors was treated with basic fibroblast growth factor (bFGF) for an additional 8 days. Differentiation was triggered by the removal of bFGF. A subpopulation of enriched progenitors differentiated into cells that expressed the hair cell markers Math1,19,20 myosin VIIA,21 espin,22,23 or parvalbumin 3.24 A subset of differentiated cells coexpressed Brain3.125 and Math1, or myosin VIIA and Math1. These data represent the first demonstration of a stepwise guidance protocol to generate a differentiated cell type in vitro whose molecular profile is consistent with that of sensory hair cells. Adult stem cells, in contrast to ES cells, are undifferentiated cells in a mature tissue or organ that retain the ability to generate component cells of the tissue in which they reside. Stem cells have been identified in many tissues and organs including the central nervous system,26 the blood,27 the skin,28 and the eye.29 Neural stem cells in the brain, for instance, give rise to neurons proper as well as two nonneural lineages, the astrocytes and oligodendrocytes.30 Epithelial gastrointestinal stem cells generate the full panoply of resident cells within the gut including adsorptive, goblet, Paneth, and enteroendocrine cells.31 Our understanding of adult stem cell plasticity, however, is evolving. Bone marrow-derived cells (BMDCs), for example, can contribute to regeneration in the liver, heart, and brain following bone marrow transplantation. Initially, transdifferentiation of BMDCs was thought to generate the diversity of differentiated cell types observed, though more recent work has shown that spontaneous cell fusion can account for cell fate switching.32 Many adult stem cell types grown in culture form clonal, free-floating colonies called spheres. This sphere-forming property has recently been exploited to identify stem cells in the sensory epithelium of the adult mouse utricle.33 The stem cell isolation started with dissection of the utricle to isolate its sensory epithelium, the macula. Several maculae were dissociated by mild trypsin treatment and strained to remove cell aggregates and debris. Spheres formed by plating at low density in untreated plastic Petri dishes in the absence of growth factors. It was previously shown that specific growth factors promote the proliferation of the vestibular sensory epithelium after damage.17,18 Li and colleagues33 took advantage of this observation to augment sphere formation by the addition of EGF, IGF-1, or bFGF to the culture media. EGF and IGF-1 cotreatment bolstered sphere formation threefold over non-growth factor-treated controls. Adult stem cells have a limited capacity for self-renewal. Li and colleagues33 demonstrated the high proliferative capacity of stem cell-derived spheres by the incorporation of a uridine analogue into the component cells. Self-renewal was demonstrated by dissociation of a primary sphere into single cells and assaying for secondary sphere formation. Approximately 2.5 secondary spheres form from a dissociated primary sphere, indicating that the stem cell lineage was retained in the primary sphere and could be expanded to form additional spheres. With this method, it was possible to routinely culture primary spheres through at least 10 passages, indicating the substantial capacity of adult mouse utricular stem cells to self-renew in culture. The observation that adult mouse utricular stem cells grow as clonal, free-floating colonies that express the intermediate filament nestin suggested that the stem cell-derived spheres contained a significant population of progenitor cells.34,35 To test the pluripotency of stem cell-derived spheres in vitro, Li and colleagues33 challenged sphere-derived cells with several growth environments and then determined which cell types were formed. In vitro differentiation of stem cell-derived spheres gave rise to neurite-bearing cells expressing neuron-specific β-III tubulin, as well as cells expressing glial fibrillary acidic protein, an intermediate filament characteristic of astrocytes in the central nervous system as well as inner ear supporting cells.14,36 Coculture of sphere-derived cells with C2C12 myogenic cells resulted in spindle-shaped, multinucleated cells positive for the muscle marker fast myosin heavy chain. These data indicate that representative cell types of the ectodermal (i.e., neuronal) and mesodermal (i.e., myocyte) lineages form in vitro from adult inner ear stem cell-derived spheres. Heterotypic grafts involve the transplantation of cells or tissue from one species into another. The developing embryo is an attractive model system for evaluating pluripotency because of the extraordinary diversity of inductive signals present. To address the plasticity of adult mouse inner ear stem cells in vivo, Li and colleagues33 transplanted adult mouse inner ear stem cell-derived spheres into the amniotic cavity of early-stage chick embryos growing in ovo and determined what cell types the progenitors generated. Genetically labeled mouse grafts differentiated into cell types from each of the primary germ layers. Graft-derived Pax2 positive cells in the liver expressed transferrin, suggesting that they are endodermally derived.37 Sphere-derived, cytokeratin-positive cells in the skin indicated differentiation along the ectodermal lineage, consistent with the observation of neuron-like cells generated in the in vitro assay. Finally, transplant-derived Pax2 positive cells in the heart coexpressed desmin,38 indicating differentiation along the mesodermal lineage, which is again consistent with myocyte formation in the in vitro assay. Adult mouse inner ear stem cells are therefore pluri-potent, capable of giving rise to cell types from all three embryonic germ layers. Can hair cells be generated from adult mouse inner ear stem cells? Li and colleagues33 showed that a subpopulation of sphere-derived progenitors differentiated into cells expressing the hair cell markers myosin VIIA and espin in vitro. In addition, myosin VIIA- and espin-positive cells presented filamentous actin-bearing epithelial protrusions on their apical surfaces reminiscent of hair cell stereociliary bundles.39 Furthermore, the supporting cell markers pan-cytokeratin17 and p27kip140 never colocalized with cells expressing hair cell–specific markers. These data indicate that a distinct subpopulation of stem cell-derived progenitors differentiated into hair cell-like cells in vitro. Importantly, neurosphere-forming cells41 cultured in precisely the same way as the utricular sphere-derived cells did not generate myosin VIIA- and espin-positive cells, suggesting that inner ear-derived stem cell spheres may be predisposed to form hair cells. The determination that ES and adult inner ear stem cell-derived progenitors generate hair cell-like cells in vitro raised hopes that they may do so in vivo. The emergent principle from the in vivo work discussed thus far is that stem cell-derived progenitors respond to local cues present in their immediate environment (i.e., the developing liver, heart, and skin) to generate tissue appropriate cell types. The essential experiment then is to evaluate stem cell plasticity in vivo by transplanting progenitors into the developing vertebrate inner ear, and then assay for the cell types generated by the grafts. To appreciate this experimental approach, a brief discussion of inner ear morphogenesis follows. In-depth discussions of the genetic basis of inner ear development are presented in a series of recent reviews.42,43 The inner ear is perhaps the most structurally and functionally complex sensory organ in higher vertebrates and it consists of two parts: the membranous and bony labyrinths. The membranous labyrinth originates from the otic placode (Fig. 4–1), a thickened sheet of epithelial cells lying adjacent to rhombomeres 5 and 6 of the developing hindbrain.44,45 Otic epithelial cells in the pla-code are called progenitor cells and they give rise to all of the constituent cell types of the inner ear, as well as the neurons that permit the inner ear to communicate with the brain. Over 2 days in birds and mice, the otic placode invaginates to form a cup whose edges cinch together, forming a fluid-filled vesicle.46
Stem Cell Characteristics
Embryonic Stem Cells
Embryonic Stem Cells Originate from the Preimplantation Mammalian Embryo
Embryonic Stem Cells Self-Renew and Are Pluripotent
Sensory Hair Cells Derived from Embryonic Stem Cells
Adult Stem Cells
Adult Stem Cells Produce Tissue-Specific Cell Types
Stem Cells Are Present in the Adult Mouse Utricle
Utricular Stem Cells Demonstrate Pluripotency in Culture
Utricular Stem Cells Are Pluripotent In Vivo
Utricular Stem Cells Generate Hair Cells in Culture
The Chick Model System for Assaying Stem Cell Plasticity In Vivo
Inner Ear Morphogenesis
The Inner Ear Is Structurally Complex
Stay updated, free articles. Join our Telegram channel

Full access? Get Clinical Tree
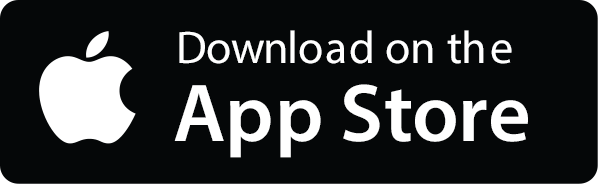
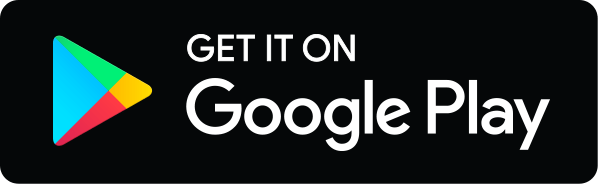