where
P g − P e = the pressure gradient between stomach and lower esophagus
R = the radius of the esophagus
L = the length of the LES (essentially, the distance from crura to GE junction)
η = the fluid viscosity
As a model of flow through the LES, this equation is wrong, but useful. The flow through the LES is not laminar, the tube is not rigid, and none of the parameters is constant. Still, the relationship given in the equation does reveal what constrains the mechanics of the LES as a valve. Several elements of the LES work together to create its asymmetric resistance [8]. All of these physiological elements (and all successful treatments for GERD) work through at least one element of this equation. The functional elements of the LES include:
1.
Crus muscle: When contracted, the diaphragmatic crura shorten and pinch the esophagus. This decreases the radius R, blocking reflux. It also contributes to the pressure gradient ∆P blocking GER by increasing P e.
2.
Intrinsic tone (or elastance) of the distal esophagus: most reflux occurs during “transient lower esophageal relaxations” (TLESRs), or brief episodes when the LES relaxes [6]. In contrast, the elastance of the LES inhibits reflux by reducing R and by creating higher pressure P e in the LES than in the stomach.
3.
Intra-abdominal esophageal length: the length of the esophagus inferior to the diaphragmatic crus (L). A “negative” length here would be a hiatal hernia, where the gastroesophageal junction (GEJ) sits superior to the crura. In the newborn, the intra-abdominal esophagus may be less than 1 cm, but is reported to be 3 cm at 3 months of age [8]. Perhaps this anatomical development (as well as other effects) explains in part how infants tend to “grow out of” reflux problems.
4.
The cardiac angle: Also known as the “Angle of His.” It is believed that an acute angle protects against reflux because a filling fundus will tend to close the LES if the angle is acute, but tends to open the LES if the angle is obtuse. When functioning properly, this mechanism should both reduce R and produce a restrictive pressure gradient between stomach and LES.
All of these are weaker in small children, increasing the propensity to have GER events. But even if the LES was functioning well, it bears repeating that it is not a perfect one-way valve. As most pregnant women can attest, a strong enough pressure gradient from abdominal to throracic cavities can overcome the resistance in the LES. Infants have extra mechanisms that similarly increase the tendency to overcome the LES:
Scaling: Mismatch between gastric scaling and energy scaling means that the stomach of an infant carries a larger burden than an adult’s. While the capacity of the stomach scales with body mass linearly (a reasonable heuristic is around 22 mL/kg), energy scales nonlinearly, roughly according to an inverse power law [9, 10]. For example, a 3 kg infant will require around 128 kcal/kg/day but his 80 kg father uses just 34 kcal/kg/day. However, both have similar gastric capacity relative to body mass. It is this mismatch that explains why infants need to eat every 3 h, and why they are so “spitty”: To ingest 128 kcal/kg/day of breast milk (~20 kcal/30 mL) requires drinking 576 mL/day, or 72 mL every 3 h, or 24 mL/kg/feed—right at the limit of gastric capacity. A constantly “loaded” stomach increases opportunities for reflux events.
Relatively slower gastric emptying: It appears that infants (especially premature infants) have relatively slow emptying of gastric volumes compared to adults, a problem easily made worse by various medications administered to neonates [11, 12]. This longer dwell time probably contributes to GER by increasing the probability of refluxate with a given TLESR.
Work of breathing: Breathing requires energy, and is associated with cyclical pressure gradients between abdominal cavity and chest cavity. Here again we see a mismatch between linear and nonlinear physiological scaling [13]. The disproportionally greater energy requirements (
) and carbon dioxide production (
) plus the linear tidal volume (~7–8 mL/kg/breath) translate necessarily into a higher respiratory rate. For a given airway impedance, work is a function of this rate. Of course, several diseases greatly increase impedance per breath, compounding increases of WOB in sick infants.


These mechanisms explain why babies universally have some GER (Fig. 1). Moreover, “some GER” may be quite a lot, with around 70 GER events/day recorded in normal newborns [14, 15]. But nothing here explains why some infants cross a threshold into GERD. It is reasonable to assert that GERD appears whenever the amount of airway soiling exceeds some capacity of the airways to recover. It follows that GERD will become manifest whenever the amount of refluxate in GER is very high (e.g., hiatal hernia, increased frequency of TLESRs, etc), the ability to protect the airway from refluxate is inhibited (e.g., neurological degradation, tracheomalacia, etc.), pulmonary repair mechanisms are degraded (e.g., bronchopulmonary dysplasia (BPD), pulmonary hypertension, cystic fibrosis, etc.), or the character of the refluxate is particularly toxic (e.g., acid, bile acids, pepsins, bacteria).
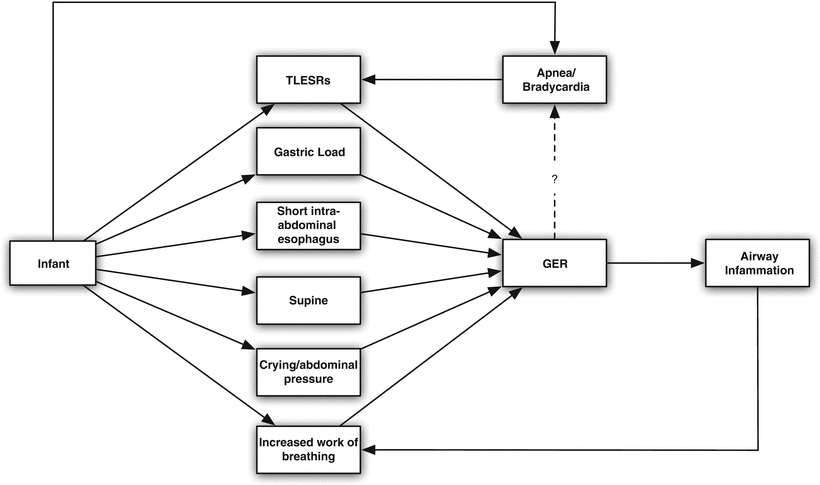
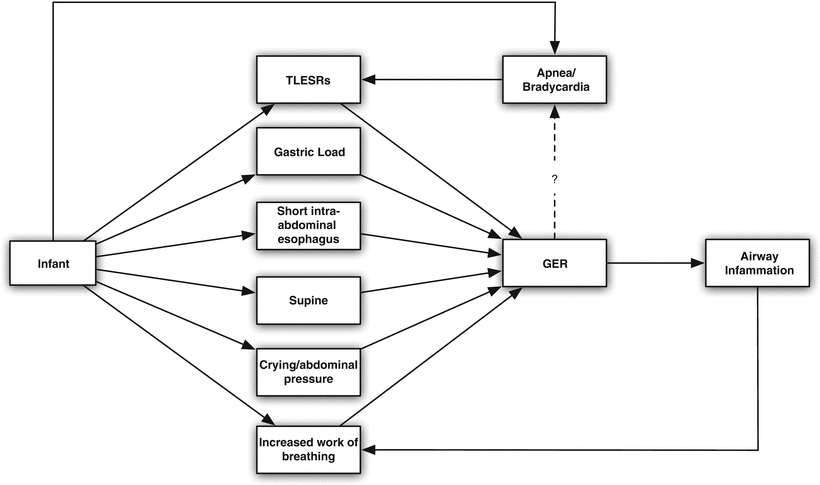
Fig. 1
Multiple mechanisms are likely to contribute to GER in infants, but GER only becomes GERD when the airway and lungs become damaged. The connection between GER and apnea is less clear. At least two positive feedback loops may exist here, where the effects of reflux potentiate factors that contribute to reflux
Several surgical diseases in the neonate also come accompanied by GERD. Patients with diaphragmatic hernia, tracheoesophageal fistula, and gastroschisis commonly exhibit difficulties with enteric feeds, and resort to surgical control of reflux is common here. In all of these, a specific failure of the LES can be posited. For instance, in CDH, the diaphragmatic crus is typically dysfunctional and GERD is common [16–18]. In TEF, the distal esophagus has decreased tone and motility [19]. In gastroschisis, hiatal hernia is common [20], and intra-abdominal pressure can be elevated for weeks while downstream small bowel motility [21] (and therefore gastric emptying) can be slowed for months (or longer).
Regardless of etiology, lungs are damaged by large amounts of debris (milk proteins and fats); acid, bile acids, bacteria, and digestive enzymes. Of these, it seems that acid, while plainly damaging to the respiratory epithelium, is the least problematic in infants. Certainly acid in the esophagus produces a noxious feeling that can lead to arching, pain, and reduced oral intake (“food fear”). But babies tend to have weakly acidic refluxate [15]. As a result, in neonates there may not be enough acid reaching the airway to provoke the intense inflammatory reaction seen in GERD both clinically and experimentally.
The same cannot be said about the effects of digestive enzymes, particularly the pepsins. This family of proteases (particularly Pepsin 3) is manufactured only in the stomach. While pH-dependent, the enzyme remains active at relatively high pH (i.e., pH 5) and does not denature until pH > 7.2. Once aspirated into the airways, these enzymes spark intense inflammation [22]. These inflammatory changes degrade the ability of pulmonary epithelium to clear debris and aspirated organisms. Probably, the lungs become more vulnerable as their “clearance capacity” is overwhelmed by large amounts of aspirate, and later even relatively small amounts of aspirated refluxate may provoke inflammation, wheeze, cough and elevated work of breathing. These inflammatory changes are posited to exacerbate BPD, pulmonary hypertension, and prolonged oxygen requirements in infants. However, despite a great deal of opinion and investigation, this causal chain has not been established. Still, in documented refluxers, damage to the airway may sometimes be demonstrated on inspection (see Fig. 2).
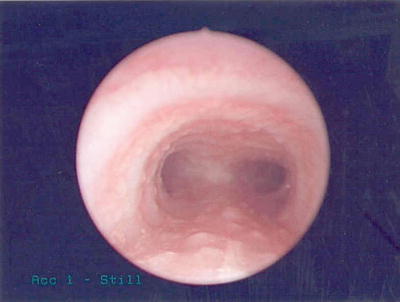
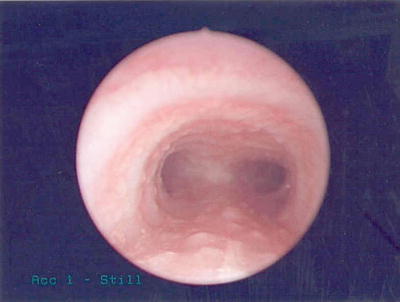
Fig. 2
A bronchoscopic view of the trachea and carina in an infant with GERD. Observe the “cobblestone” eruptions along the epithelium attributed to constant microaspiration of gastric contents
At this point, one may notice that the elements of this list could comprise a harmful positive feedback loop between lung damage and reflux. As reflux damages the lung parenchyma, work of breathing and inspiratory pressure may rise. As these rise, energy needs for growth increase, further increasing the filling pressure on the stomach while the inspiratory pressure steepens the pressure gradient between abdominal and thoracic cavities. Meanwhile, apneic spells may not always be caused by GER as commonly believed, but may not be effective but cause, contributing to TLESRs [23]. All of these effects must increase refluxate, leading to further lung damage. Treatment, then, should be aimed at interrupting this positive feedback loop.
Diagnosis
Diagnostic interventions seek to determine whether GER may be the cause of pulmonary manifestations or failure to thrive. There is no single best test for “GERD” in infants. Instead, the clinician must combine specific testing with clinical context of a given patient. Several diagnostic tests are available.
pH-probe/impedance probe: probes introduce an esophageal tube with multiple side ports each able to detect pH or electrical impedance or both. For pH probes, a falling pH at a certain detector site in the esophagus is a proxy for gastric contents, presumably with low pH. Similarly, a decline in impedance suggests fluid at the detector. With multiple channels, these devices reliably detect GER (and its character—acidic, weakly acidic, or alkaline), and give an indication of its severity: how far up the esophagus refluxate travels, how often refluxate appears, and how fast it is cleared [24]. pH/impedance is often referred to as the “gold standard” for diagnosis of GER. It cannot however provide sole evidence that GER is actually GERD, nor can it reveal problems with gastric emptying or altered anatomy (like malrotation or hiatal hernia).
Fluoroscopy: To reveal anatomical problems that may present as GER, esophagosocopy and upper gastrointestinal (UGI) series are helpful. While insensitive to GER (reported sensitivity is under 50 %) [25], the images are far more specific in revealing hiatal hernia, H-type tracheoesophageal fistula, microgastria, esophageal stricture, and malrotation. Some surgeons insist on UGI before fundoplasty in order to plan the operation, for example to allow for the need to perform a Ladd’s procedure concomittantly [26] or even instead of fundoplication [27]. Data suggests that at least 4 % of patients being considered for fundoplasty will be found to have a surgically important abnormality [28]. But this value alone does not give the value of information from the UGI. For example it is reasonable to place premium value on decreased uncertainty about malrotation; that is, to assert that avoiding one missed malrotation is worth more than the cost of 24 negative studies. Value judgments of this kind resist standard calculus.
Manometry: this method uses a series of pressure sensors in the esophagus to describe esophageal pressure waves. Critical for diagnosis of motility disorders like achalasia in older children and adults, manometry contributes little to the workup of GERD in infants.
Esophagoscopy: Regarded as a mandatory diagnostic step by some, esophagoscopy is critical for diagnosis of esophageal metaplasia, ulcers, eosiniphilic esophagitis, conditions rarely encountered in newborns. Like manometry, esophagoscopy is not routinely used in newborns for workup of GERD.
Bronchoscopy: Bronchoscopy may provide compelling evidence of reflux with aspiration (see Fig. 2) but is not a front line tool in initial workup. On the other hand, patients who appear to have severe “reflux” may require interrogation of the airway to identify other structural problems that can mimic GERD, e.g., laryngeal cleft, H-type tracheoesophageal fistula, or tracheomalacia.
Nuclear scintigraphy: Radionuclide scintigraphy is attractive because it is noninvasive, carries no X-ray exposure, and can purportedly detect GER and microaspiration while quantifying gastric emptying. When compared to pH monitoring, the sensitivity of radionuclide scintigraphy is reported to be between 75 and 100 %, and specificity between 81.2 and 100 % [29]. But the usefulness of these “milk scans” in practice is less clear. Milk scans confirm that reflux is common in preterm infants, but it turns out that “positive scintigraphy has no correlation with symptoms” [30]. In that study, the authors found no discrimination at all in detection of GER between symptomatic babies and asymptomatic babies. Others report that the scans do not offer any information that guides whether pyloroplasty is indicated along with fundoplasty (Pyloroplasty is very rarely indicated in any case, and its routine use with fundoplication is condemned) [31]. Most likely, these scans are of little value in infants in the NICU: virtually always “positive” (even in children who have intact and working fundoplasty), their specificity appears considerably lower than reported for other populations. At the same time, the sensitivity to detect aspiration also appears in daily practice to be far below reported levels. In other words, in infants, they merely confirm reflux that is nearly universal, while failing to detect the “disease” in GERD.
Biomarkers: In patients undergoing bronchoscopy, who are intubated, or have a tracheostomy, bronchoalveolar lavage allows examination of the fluid in the airways. While traditional diagnosis relied on the presence of “lipid-laden macrophages” as a proxy for lung soilage, this finding appears to lack specificity [32]. Others have used the presence of pepsin [22, 33], measured according to various assays, to indicate GERD. This method seems to have promise, but lack of guidelines regarding interpretation of results, lack of standard methods for measurement, limited availability of the tests and restriction to patients with some tube in the airway all prevent widespread use for now.
N-of-one trials: While this method has the least published evidence, it is arguably the method to which experienced neonatal clinicians resort the most. N-of-1 trials are single-patient trials with “multiple-period crossover experiments comparing two or more treatments within individual patients” [34]. In detecting GERD, the clinician will observe the patient while being fed normally (orally or by tube) into the stomach. Frequency of apneas, oxygen requirement, discomfort, and other signs of symptomatic reflux are noted. Then feeds are withheld, with nutrition supported either by nasojejunal enteral feedings or parenteral nutrition. If GER is really GERD, improvement in respiratory and other symptoms will manifest within 2 or 3 days (sometimes less). Return to gastric feeds will reproduce the symptoms, and a second period of non-gastric nutrition relieves them. Each “block” of these trials is about 1 week long. While this method does not rule out laryngeal cleft or other anomalies in orally fed children, these confounders can be controlled by restricting enteral nutrition to tube feeding. While evidence is scant regarding this method, and criticisms are plain (jejunal feeds are imperfect protection against reflux; trials take a long time; etc.), this pragmatic method may yield the highest individual validity.
Medical Treatment
Traditionally, the mainstay of control of GERD has been drugs which fall into two categories: motility agents and acid blockers.
From the discussion above on pathophysiology, the usefulness of acid blockade on the control of GERD manifestations should seem suspect. Nothing in the function of the LES depends on pH; acid is weak in infants already; and decreased pH creates an important “deflector screen” against enteric organisms migrating from the stomach back into the airway. It is plausible that reliable acid control could act to deactivate pepsin and other digestive enzymes, but this has not been shown. At the same time, while improvements in pediatric asthma have been shown in the past, more recent trials show no benefit, and even harm in asthmatic children [35]. Meanwhile, there is increasing skepticism about acid blockade in infants. Proton-pump inhibitors (PPIs) carry serious risks and little effect on GERD symptoms [36], and even H2 blockers were recently associated with serious, even fatal, problems in infants [37]. While these medications have their place in gastritis with bleeding, or GERD manifesting largely as oral resistance and discomfort, it is plain that they are over-prescribed [1] and undereffective.
Motility agents fare little better. Metoclopramide (Reglan), bethanechol, cisapride, and erythromycin have all been tried as frontline treatments for GERD. In theory, increasing “motility” (i.e., decreasing gastric emptying time, decreasing intestinal transit time) should decrease GER events associated with TLESRs by reducing the dwell time of feeds in the stomach.
These posited effects have not been demonstrated practice. Reglan has no measurable effect on GERD in infants but carries a high risk of dystonic reactions [38]. Cisapride was shown to be effective, but was removed from the US market after several patients developed cardiac dysrythmias attributed to it [39]. Bethanechol, a parasympathomimetic, was posited to aid GERD by increasing intestinal motility, but trials have failed to demonstrated effectiveness (for example [40]). Erythromycin, a motilin agonist, certainly increases gastric emptying, but has not been shown to be an effective treatment for GERD, perhaps because of its strong tendency toward tachyphylaxis [39, 41]. At one time, it was common to place almost every baby in the NICU on “R&R” (reglan and ranitidine), a practice now, unsurprisingly, out of favor.
The reflux equation described above illustrates why other therapies that seem reasonable have unclear power to attenuate GER. Chief among these is the practice of “thickening” feeds. In this practice, a thickening agent (rice cereal, guar gum, cornstarch) is added to milk or infant formula. This “thickening” is actually a change in viscosity, η. From the equation, it is apparent that in order to halve GER, one would need to double the viscosity. The viscosity of human breast milk is around 3 cP (pumped, untreated), but addition of a commercial thickener (Nestle ThickenUp®, a preparation of cornstarch) demonstrated a large increase in measured viscosity: “In relation to untreated pumped human milk without thickener, we observed that the addition of 7 % of the thickener increased the viscosity up to ninefold” [42]. So far so good. But this improvement comes at a cost—delayed gastric emptying. There are other costs: infants have diminished alpha-amylase and thus diminished ability to digest cornstarch which can produce diarrhea [43] Increasing viscosity delays gastric emptying [44]. With contrary effects and varied effects of different thickeners plus the confounder of varied viscosity of human infant milk formulas, mixed results with use of thickeners is unsurprising. Pectin, another thickening agent, appears to delay gastric emptying [45] but a trial in neurologically injured children showed improvement in GERD [46]. In sum, no reliable evidence exists to support or decry use of thickeners as GER treatment in infants. A Cochrane review in 2002 concluded “There is no evidence from randomised controlled trials to support or refute the efficacy of feed thickeners in newborn infants with [GER]” [47]. In a more recent study, the results are, again, mixed: “A formula thickened with amylopectin did not reduce the number of apnea of prematurity or GER-related apneas. It reduced acid GER features but had no effect on non-acid GER indexes” [48].
Others have turned to “elemental” formulas in order to treat GERD. There is nothing in these formulas that can be expected to improve the function of the LES or to increase gastric emptying. Rather the opposite is true—all of these formulas share one counterproductive characteristic, relatively high osmolarity. While breast milk has an osmolarity similar to serum (~290 mOsm/L), elemental formulas are much higher (e.g., Alimentum™, 370 mOsm/L when prepared at 20 kcal/30 mL). The stomach responds to elevated osmolarity by holding the fluid longer and diluting, since the small bowel does not tolerate high osmolarity fluid well (producing cramping, flushing, and diarrhea recognized as dumping syndrome). Changing a baby to an elemental formula may certainly be the right intervention for many diseases (protein hypersensitivity, malabsorption, etc.). But pathophysiologically, if a change to an elemental formula does ameliorate some observed manifestation of “feeding intolerance,” it is unlikely that the cause was GERD.
Surgical Treatment
Surgical control of GER aims to restore normal functioning of the LES. “Normal” function does not include the inability to vomit, the inability to burp, or total elimination of reflux. Instead, surgery aims to re-establish or reinforce the mechanical functions that the LES normally employs [8]. To review:
1.
The “pinch cock” effect of the crus muscle.
2.
Adequate intra-abdominal esophageal length.
3.
An acute angle at the cardia (Angle of His).
4.
Decreased compliance (increased elastance) at the lowest portion of the esophagus, i.e., “tone”.
An additional surgical objective is removal of barriers to gastric emptying (e.g., malrotation, overdistended stomach, etc.). The underlying objective is to attain these results without creating unwanted problems like dysphagia.
All surgical fundoplasties achieve these mechanical objectives. It may surprise readers familiar only with the “Nissen” fundoplication, but surgical control of GERD does not begin and end with the Nissen. Instead, there are several approaches (Table 1).
Table 1
Common types of fundoplasty for reflux control
Complete wrap (360°) | – | Nissen |
Nissen–Rossetti | ||
Collis–Nissen | ||
Floppy Nissen | ||
Partial wrap | Anterior | Dor (180°) |
Thal (240°) | ||
Boix Ochoa (240°) | ||
Belsey Mark IV (240°) | ||
Posterior | Toupet (270°) | |
No wrap | – | Hill gastropexy |
While all these procedures have an eponym, rarely does the modern application of various procedures conform to the original description. This chapter confines discussion to the three most common fundoplasty types performed in North America:
Nissen: the modern Nissen is sometimes called a “floppy Nissen” to distinguish it from its original namesake, or even its modified form the “Nissen–Rossetti.” The modern Nissen creates as 360° wrap looser and shorter (e.g., 2 cm) than originally described, and involves division of the short-gastric vessels between spleen and the greater curvature. Unlike the variant called a “Collis-Nissen,” the modern Nissen places the wrap entirely above the GEJ (Fig. 3).
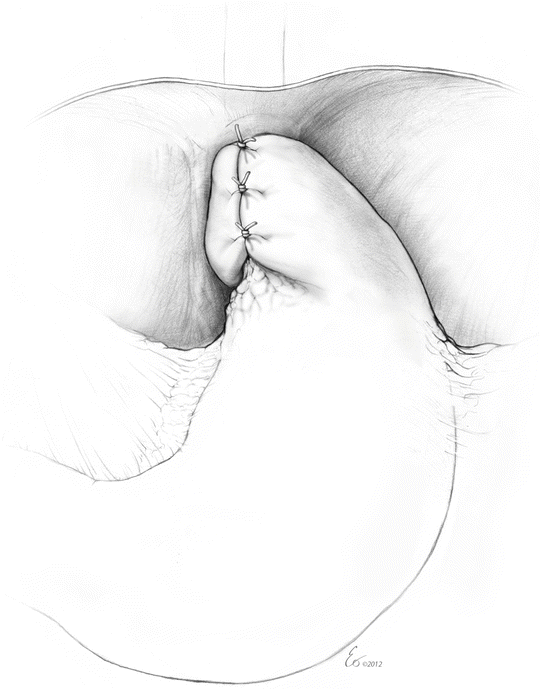
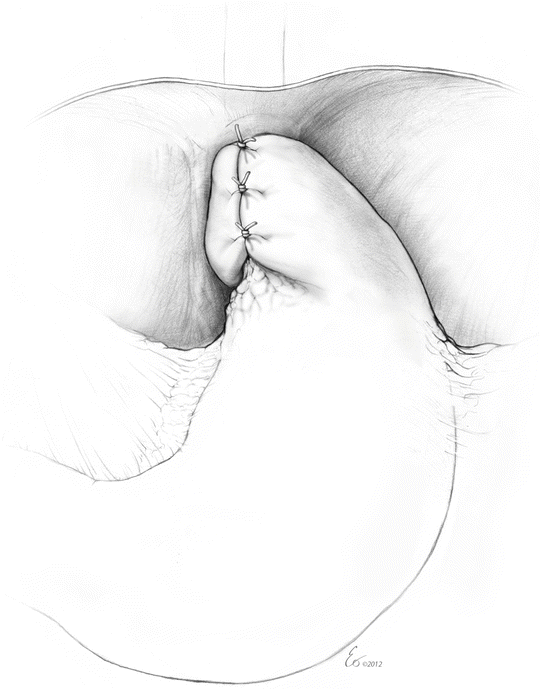
Fig. 3
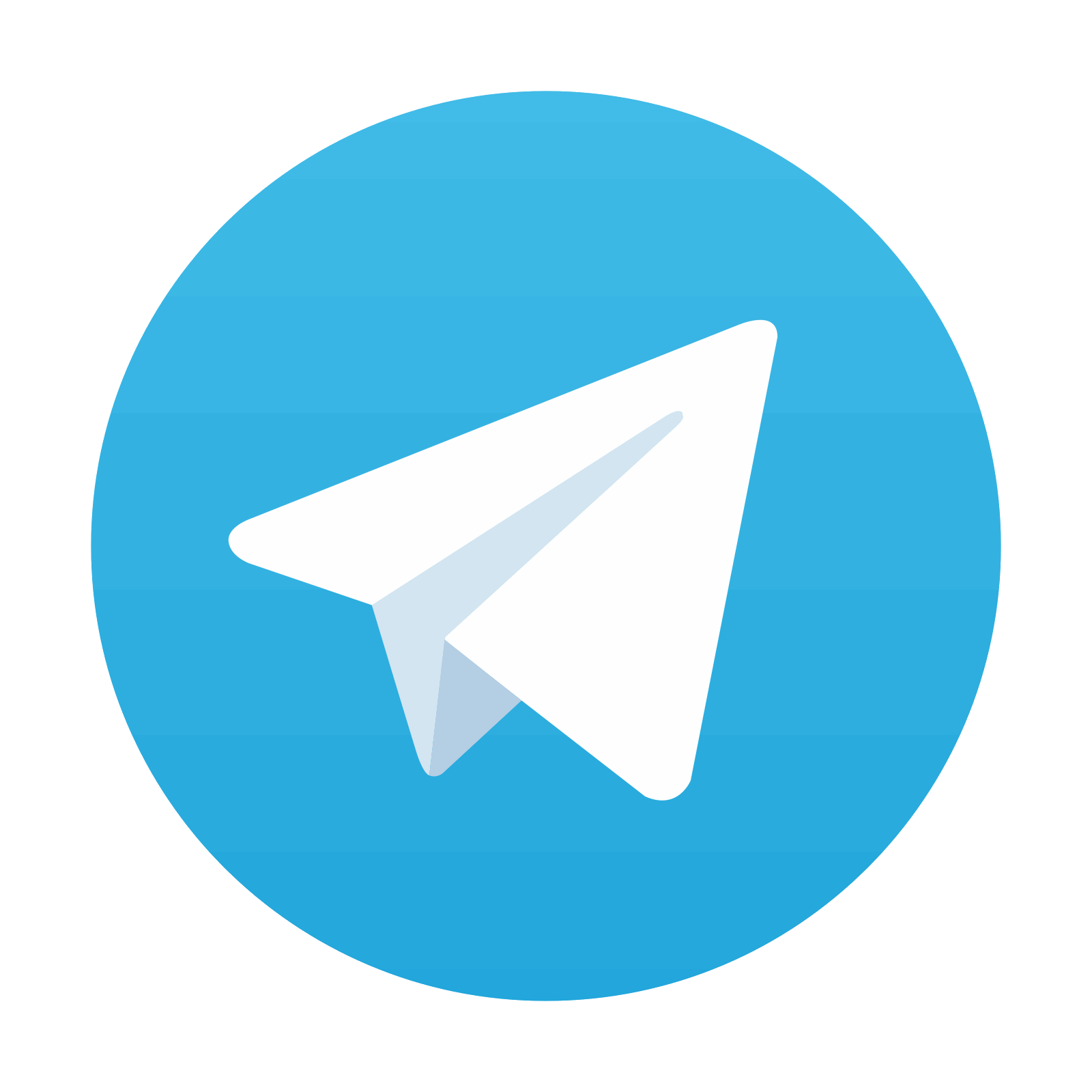
The modern Nissen fundoplication is a 360°, loose wrap located between the diaphragm and gastroesophageal junction (GEJ)
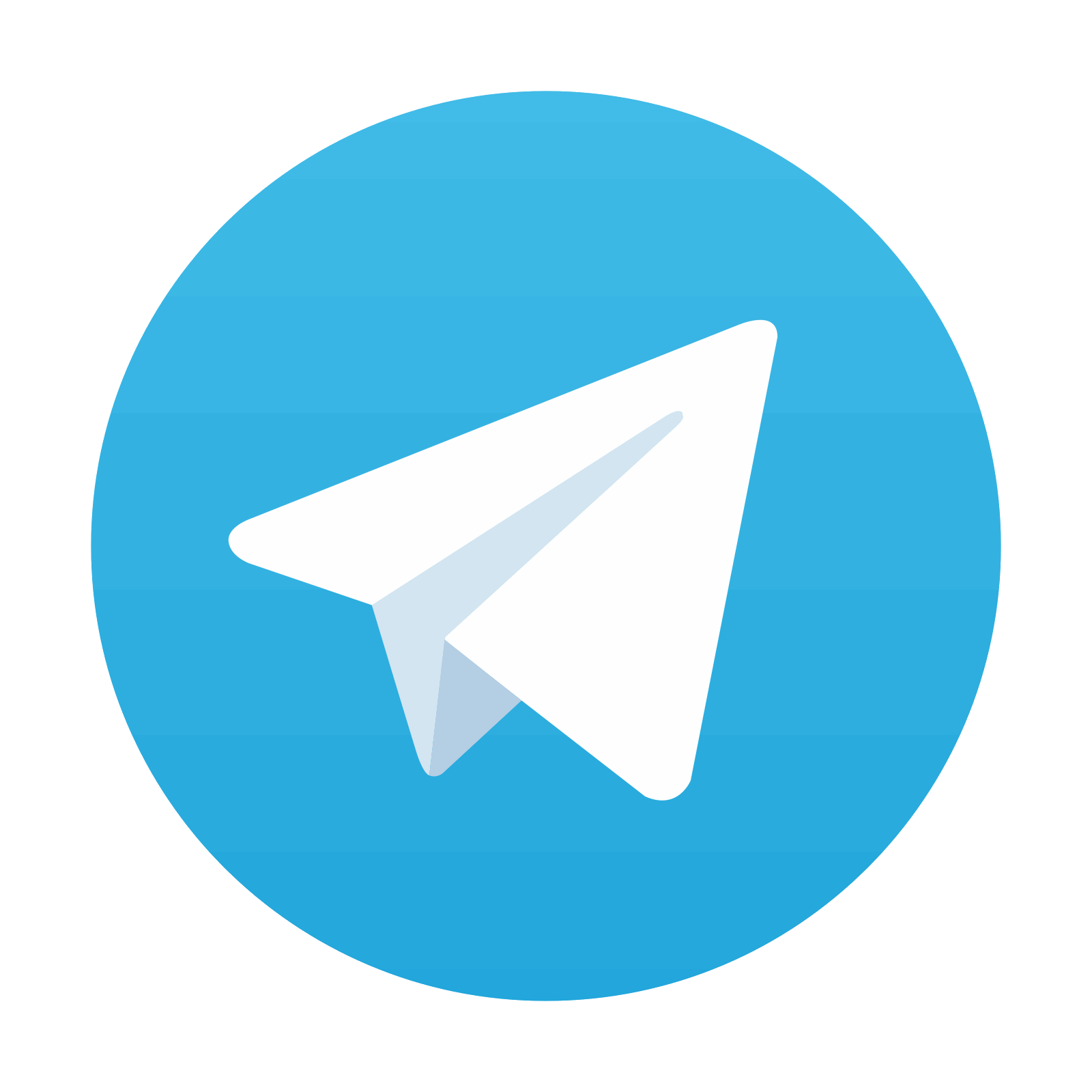
Stay updated, free articles. Join our Telegram channel

Full access? Get Clinical Tree
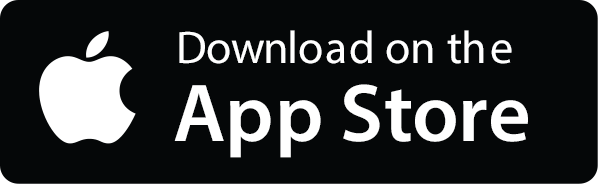
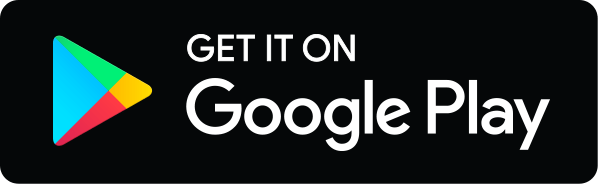
