Radiation for the Treatment of Wet Age-Related Macular Degeneration
Robert Petrarca
Timothy L. Jackson
INTRODUCTION
At present, most patients with wet age-related macular degeneration (AMD) are treated with regular, intravitreal injections of drugs directed against vascular endothelial growth factor (VEGF), namely ranibizumab, bevacizumab, and aflibercept (1,2,3,4,5). While these drugs offer visual outcomes far better than the natural history, all require an expensive and burdensome course of treatment. The large phase III studies of ranibizumab used monthly dosing. For pragmatic and cost reasons, most clinicians use an as-required (PRN) (6) or “treat and extend” dosing regimen (7) that entails regular hospital visits and usually several injections per year. Further, not all patients respond fully, and many of those who do respond initially, subsequently lose vision (8). There is therefore a need for a more durable treatment modality that can reduce the cost and burden of treatment, while maintaining or improving vision. This chapter explores the early attempts to use radiation as a treatment for wet AMD, and more recent attempts to revisit this treatment modality.
BACKGROUND TO RADIATION THERAPIES
Radiation is energy emitted from a source and can be classified into nonionizing radiation (visible light, infrared, and radio waves), and ionizing radiation (alpha, beta, gamma, and x-rays). Radiation is ionizing if it creates an ion (a charged particle) when it interacts with an atom. This will only occur if the particle or photon of radiation has sufficient energy to detach electrons from another atom or molecule (9).
Ionizing radiation creates free radicals, which are highly reactive charged molecules or atoms. In the context of radiation therapy, these free radicals are usually generated from the interaction of radiation and water molecules. If this occurs within a living organism, then the free radicals can damage DNA. In addition, radiation can ionize DNA itself, breaking its molecular bonds. Alpha and beta particles are directly ionizing, in that they carry a charge and directly interact (via coulombic forces) with atomic electrons, causing excitation. Gamma and x-rays are indirectly ionizing, because they are electrically neutral and do not directly interact with atomic electrons but instead transfer energy to charged particles (via electromagnetic or nuclear interaction) (9).
The Gray (Gy) is the basic unit of measurement for the “absorbed dose,” which refers to the total energy absorbed per unit mass (kg), and has replaced the previous unit of measurement, the rad (1 Gy = 100 rad) (9,10).
Radiation used for medical therapies can be divided into two main categories, depending on its method of delivery to the tissue. Brachytherapy from the Greek word brachy, meaning “short distance,” places the source of radiation close to the target lesion. The source is usually an isotope that produces ionizing radiation as it decays and emits energy. Teletherapy (external beam therapy) from the Greek word tele, meaning “from afar,” uses radiation formed into a beam that can be projected at an internal body tissue from an external source. The source can also be an isotope, but more recently, electronically produced ionizing radiation has been used (11).
RADIATION AND NEOVASCULAR AMD
There are biologic principles suggesting radiation may have potential as a treatment for wet AMD. Neovascular AMD is similar to a proliferative wound healing process, and proliferating cells are known to be sensitive to the effects of ionizing radiation. Localized radiotherapy causes irreparable damage to DNA and protein synthesis, preventing further replication, while still maintaining cellular integrity (12,13,14). All cells in the treatment zone are affected; however, radiation has a selective effect. This is because nondividing cells are able to repair the damage to their DNA, while the rapidly proliferating cells discontinue the cell division cycle and undergo apoptosis (15).
The retina and optic nerve are relatively resistant to the effects of radiation, as they consist of highly stable cells with minimal mitotic activity (16,17). By contrast, choroidal neovascularization (CNV) is propagated by mitotic activity, with rapidly replicating endothelial cells that are more sensitive to radiation than are the nonproliferating capillary endothelial cells seen in mature retinal vessels (18,19). There is a potential therapeutic range where the radiation can be safely administered and yet inhibit the CNV vascular endothelium proliferation, causing regression or selective cell death. Fibroblast proliferation is also inhibited (20). Therefore, radiation has the potential to prevent both the vascular leakage and scarring associated with neovascular AMD and vision loss (21).
In addition to its selective action, radiation may also have synergy with anti-VEGF therapy. Anti-VEGF agents have a rapid onset of action, but only limited durability in many patients. In general, disease activity tends to recur as they are eliminated from the eye. By contrast, radiotherapy produces a delayed response but has a much longer duration of action. Therefore, there is rationale for a synergistic response when a combination approach is utilized as both therapies target the disease in different ways (22). The anti-VEGF therapy inhibits growth factors in the local area, while the radiotherapy disables the local inflammatory cell population and induces an apoptotic effect on the vascular endothelium. The overall result from these two approaches has the potential to bring a faster and more complete recovery of functional vision. This hypothesis is supported by clinical evidence from oncology, in which bevacizumab and radiotherapy were combined to treat colon cancer (23,24).
EARLY CLINICAL TRIALS
Radiotherapy as a treatment for neovascular AMD was first proposed in 1993, after initial animal studies and a phase 1 study that demonstrated significant regression of subfoveal CNV lesions (20,25). Initial ophthalmology case series investigated fractionated external beam radiotherapy, dividing the dose into smaller treatments (typically 2-3 Gy) over several days, to reduce damage to off-target tissue, with a total dose of 10 to 50 Gy (26,27,28,29,30,31,32). The results of these early, small, nonrandomized studies were somewhat inconclusive, especially given the variable natural course of AMD. Therefore, multiple randomized controlled trials were undertaken, using various fractionated radiotherapy doses from 7.5 to 24 Gy (33,34,35,36,37,38,39,40,41). Overall, a small but statistically significant reduction in the risk of vision loss was found (42). Although most studies reported that radiotherapy had a favorable effect, the difference was not always significant, and there was considerable inconsistency between the trials. Dose fractionation was not proven to offer an advantage over single-dose treatment (43).
Although the efficacy data were sometimes inconclusive, the majority of studies observed a reduction in scarring compared with the natural history of neovascular AMD. One study comparing bilateral disease showed an improvement in vision compared to the untreated fellow eyes for as long as 40 months after treatment (44).
The other technique investigated during these early studies was brachytherapy plaque irradiation. Various doses were used, with beta radiation sources such as strontium-90, in a single fraction dose of 12.6, 29, or 32.4 Gy (45,46) (doses differ from those published, based on recent recalibration measurements) (47). A radioactive source was introduced using an applicator positioned on the episcleral surface under the macula and held in place for up to 54 minutes. Favorable efficacy results were reported with the 29 Gy and 32.4 Gy doses, but not with 12.6 Gy.
Palladium-103 was another brachytherapy isotope investigated, with doses in the range of 12.5 to 24 Gy, delivered over 18 to 65 hours. The authors reported that 62% of patients had regression or stabilization of their exudative disease after 7 years (48,49,50).
A Cochrane review of radiation for the treatment of wet AMD was conducted in 2010. It did not include the recent epimacular brachytherapy and stereotactic radiotherapy studies but instead analyzed 14 early external beam radiotherapy trials and one plaque brachytherapy trial. It identified that the incidence of adverse events was low and that there was some evidence for an effect of radiotherapy in cases of severe visual acuity loss (6+ lines lost) over 12 months; however, this was not seen in the moderate visual loss groups (3+ lines lost) and was not maintained at 24 months’ follow-up. With only a small number of trials included in the meta-analysis, with different investigational dosages and clinical and statistical inconsistency between trials, the analyses were limited and firm conclusions could not be made. It recommended that further trials be conducted with sufficient sample sizes to detect any moderate effects and that the results of all the visual acuity outcomes were reported to allow further meta-analysis review (42).
While some radiotherapy studies showed results better than the natural history, the results do not compare favorably to those in the anti-VEGF era (42,46,51). This may be because of collateral damage to ocular tissue and difficulty targeting macular lesions using technology that was designed for cancerous lesions that are usually several orders of magnitude larger than AMD lesions. Another factor may have been the time delay before radiation has an effect. This meant the disease progressed before the benefit of radiation occurred.
Another disadvantage of early external beam therapy relates to the linear accelerators and nuclear laboratory facilities required to generate the radiation. These required large power supplies and cooling measures and produced extremely high levels of energy, which are tightly regulated and require special precautions to prevent the escape of radiation, including lead-lined concrete walls (52).
OCULAR ADVERSE EVENTS OF RADIATION THERAPY
Despite attempts to minimize collateral damage, radiation therapy can cause damage to normal, healthy cells within, or neighboring, the treatment area. The susceptibility to the effect of ionizing radiation varies widely depending on the sensitivity of a particular tissue, in relation to the total dose delivered (Table 20.1).
|
Tear Film Disruption
Studies have described epiphora or transient conjunctival irritation and dry eye syndrome following radiotherapy (35,49). The symptoms may result from radiation damage to the lacrimal and meibomian glands, the conjunctiva itself, or its goblet cells (53,54). Deficiencies in any of the tear-film components (lipid layer, aqueous layer, mucinous layer) can result in unstable tear film, leading to dry eye symptoms, with secondary changes of the corneal and conjunctival surface. These effects are usually transient, typically lasting for a few months (55).
Cataract
Radiation Optic Neuropathy
The threshold for clinically observable radiation damage to the optic nerve is reported to be greater than 55 Gy (19,57), with patients typically presenting after 8 to 16 months and very occasionally after 3 years (58). It is believed to be caused by damage to the vascular endothelial cells at the level of the choriocapillaris. This leads to vascular occlusions and vascular insufficiency of the optic nerve, which is then unable to meet the metabolic demands of the damaged tissue (59). Patients present with an anterior optic neuropathy that may manifest as papillitis and optic atrophy, characterized by initial disk swelling, hemorrhage, and cotton-wool spots (60). The prognosis is poor, often with a final visual acuity of less than 20/200, and many eyes become completely blind (61).
Radiation Retinopathy
Radiation retinopathy is an occlusive microangiopathy, secondary to endothelial cell loss and capillary closure. It leads to the formation of small, dilated collateral channels that bypass the area of ischemia and assume a telangiectatic-like form (62). It was first described in 1933 following the use of radon seeds to treat retinal tumors (63). Patients developed retinal inflammation and degeneration, with exudates, hemorrhages, retinal pigment epithelium changes, and optic disk edema. The retinopathy was slowly progressive with a delayed onset, typically 6 months to 3 years after radiation treatment, with the severity dependent on the total dose of radiation administered (64). It is believed that the retina can usually tolerate doses up to 35 to 50 Gy, beyond which radiation retinopathy may occur (57,65,66).
Clinically, radiation retinopathy has an appearance similar to that of diabetic retinopathy, with the development of microaneurysms, followed by retinal hemorrhages, telangiectatic vessels, capillary nonperfusion, infarcts of the nerve fiber layer, and vascular obliterations at the level of the choriocapillaris (67,68,69). The earliest clinical features are discrete foci of occluded capillaries and irregular dilatation of the neighboring microvasculature. Fundus fluorescein angiography can confirm the extent of capillary dropout and the general capillary competence at this early stage (70). Indocyanine green angiography at an early stage shows atrophy of the retinal pigment epithelium and choriocapillaris, and eventually, the larger choroidal vessels became nonperfused (71). Vision loss is caused by macular edema, exudation, and retinal ischemia. In the late stages, secondary ischemic complications may lead to proliferative retinopathy, neovascularization, and subsequent vitreous hemorrhages, rubeosis iridis, and tractional retinal detachments (72,73,74). Patients who develop proliferative radiation retinopathy have a poor prognosis, with most achieving only 20/200 vision or worse after 6 years (75).
Studies using newer treatment modalities have described a non-vision-threatening form of radiation retinopathy (76,77
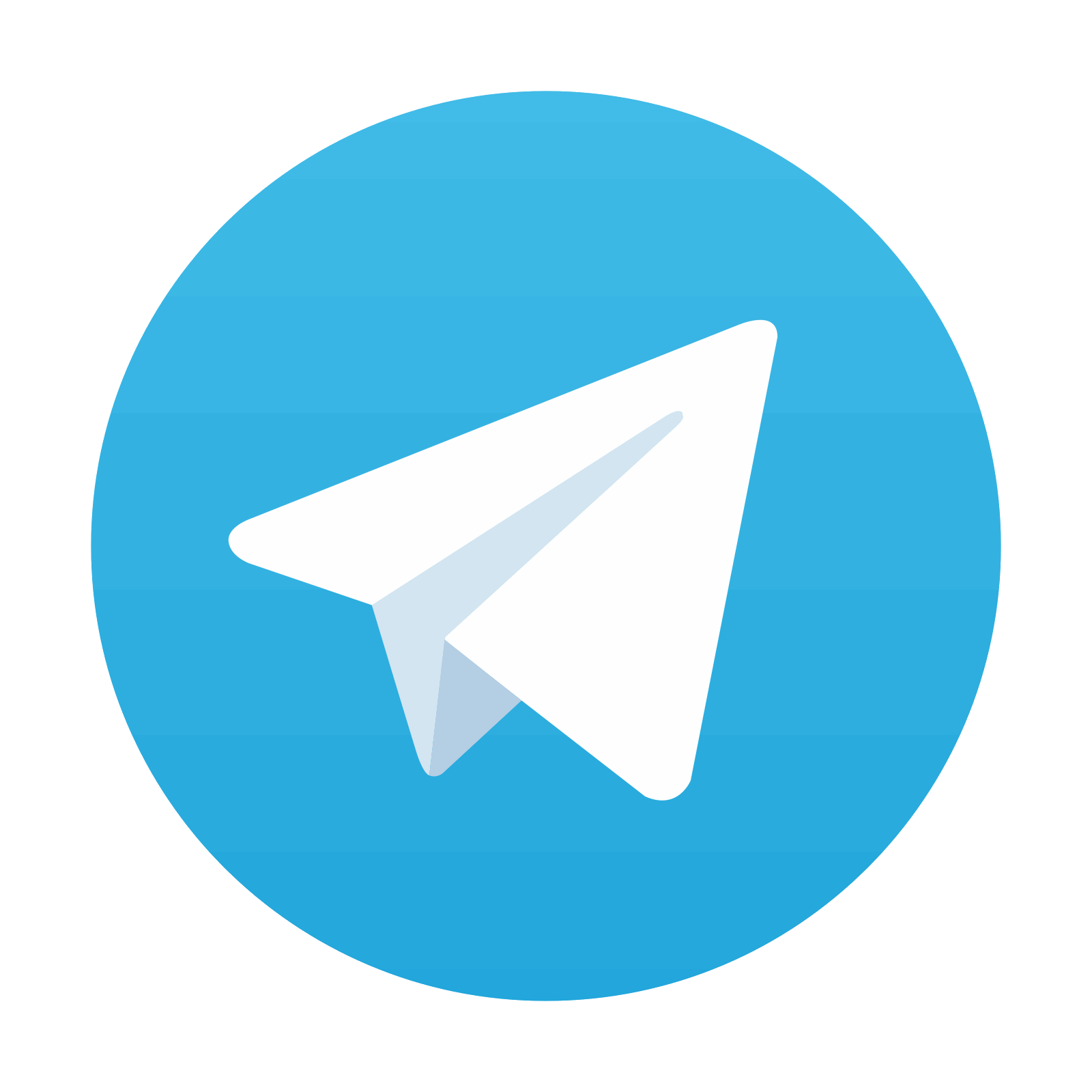
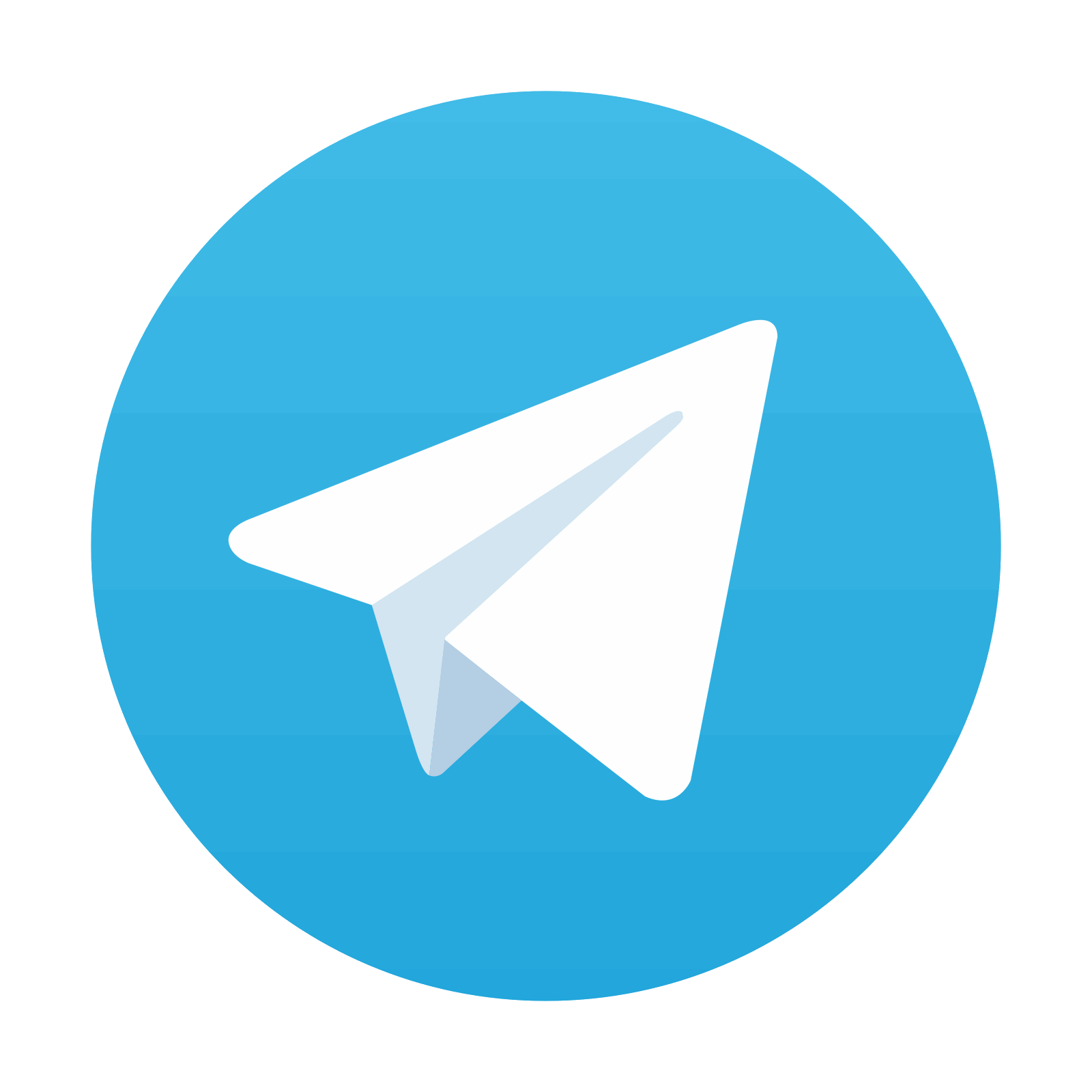
Stay updated, free articles. Join our Telegram channel

Full access? Get Clinical Tree
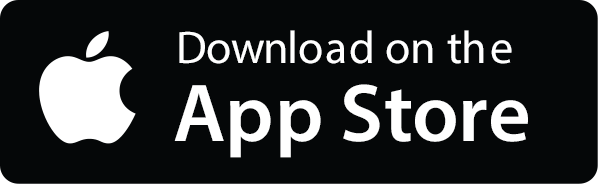
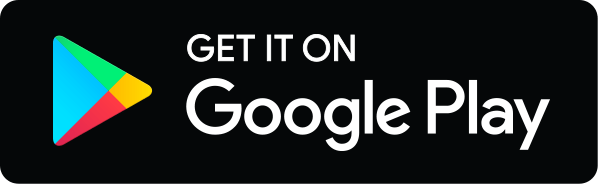