Introduction
Radiation oncology is the discipline of medicine involving the use of ionizing radiation to treat malignant neoplasias. The radiation oncologist aims to deliver a precise dose of ionizing radiation to a defined tumor volume while minimizing damage to the surrounding normal structures. Due to the increasingly multidisciplinary nature of oncology, an understanding of radiation therapy is crucial to the surgeon involved in the combined-modality treatment of the patient with head and neck cancer.
Therapeutic ionizing radiation can be divided into two categories: high-frequency electromagnetic radiation (X-rays and γ-rays) and particulate radiation (electrons, neutrons, protons). The amount of radiation absorbed per unit mass of tissue is known as the absorbed dose. The most commonly used unit for absorbed dose is the gray (Gy), which is equivalent to one joule of energy absorbed per kilogram of tissue. One Gy is also equal to 100 cGy, or 100 rads (the previously used unit of absorbed dose).
In the head and neck, primary radiotherapy is most frequently delivered via a linear accelerator with 6-megavolt (MV) photons. Anatomic location and desired depth of penetration are the main criteria used in choosing which type and energy of external beam to employ. Less commonly employed forms of external beam radiotherapy (EBRT) include 6–20 MeV electron beams, 60Co γ-rays, and superficial (40–100 kV) or orthovoltage (250 kV) X-rays.
The past several decades have seen great advances in external beam treatment delivery schemes. Traditionally, radiotherapy was often delivered with a single field using superficially penetrating X-rays. The development of machines capable of deeper delivery of radiation (ie, linear accelerators) allowed centrally located tumors to be treated with parallel opposed radiation portals. During the early 1990s, with advances in computer and imaging technology, 3D conformal radiotherapy was introduced. This allowed for noncoplanar beam arrangements that conform to the target in three dimensions. The more recent development of intensity-modulated radiotherapy (IMRT) permitted the intensity of each beam to fluctuate in complex ways across the field. This further improved the ability of the radiation oncologist to cover irregularly shaped tumor volumes, facilitating a higher degree of dose conformality and minimization of damage to surrounding normal tissues, most importantly the parotid glands and spinal cord. Recently, image-guided radiotherapy (IGRT) has emerged as a way to ensure accurate daily tumor localization during the delivery of IMRT treatment plans.
Brachytherapy is a form of radiotherapy in which a radioactive source is placed inside or adjacent to the area requiring treatment. Selected radioisotopes contained within specialized instruments deliver radiation to the tumor or tumor bed at a short distance. The treatment may involve permanent implantation of the radiation source, or a temporary placement after which the source is withdrawn. Brachytherapy treatment can be delivered via interstitial implants (eg, base of tongue, neck, or tumor bed), intracavitary applicators (eg, recurrent nasopharyngeal cancer), or molds (eg, skin, hard palate). Treatments are also defined by the dose rate, and can be divided into low/medium-dose rate (LDR), high-dose rate (HDR), and pulsed-dose rate (PDR) techniques.
The advantage to brachytherapy is the short distance between radiation source and desired target volume. The radiation dose decreases with the inverse square of the distance from the source, and exposure of normal tissues to radiation is therefore reduced. Brachytherapy is frequently combined with EBRT. This allows the areas at risk for subclinical disease to be irradiated with external beams to a dose sufficient to sterilize microscopic metastases, while brachytherapy is reserved as a boost for the gross tumor or high-risk tumor bed.
Radiobiology
Ionizing radiation deposits energy at a constant rate as it travels through matter. This rate is defined as the linear energy transfer (LET). X-rays and γ rays are considered low LET or sparsely ionizing radiation and deposit their energy less densely per unit length of tissue. Energetic neutrons, protons, and heavy charged particles are high LET (densely ionizing) radiations. Equal doses of radiation of differing LET produce distinct biological responses. The relative biological effectiveness (RBE) is a measure of the ability of radiation with differing LET to produce the same biological effect under the same conditions. Radiation with higher RBE causes greater biological damage for equivalent radiation exposure. For example, if 800 cGy of 250 kV X-rays and 200 cGy of neutrons result in the same surviving fraction of cells under the same conditions, the RBE of the neutrons would be 4.
The fraction of cells surviving a given dose of radiation is dependent on many factors, including: the radiation type (high LET vs. low LET), dose size, cell type, oxygenation, and cell cycle phase. Some cells, and by extension, some tumors are more sensitive to radiation than others. The fraction of cells able to survive a low LET dose of 200 cGy varies between 20% and 80% depending on histology.
The presence of molecular oxygen in the tissue environment also influences the biological effect of ionizing radiation. Cells in a 100% oxygen environment are approximately three times more radiosensitive than completely anoxic cells. Malignant cells in the relatively hypoxic center of a bulky tumor therefore tend to be relatively radioresistant. Nevertheless, as the better oxygenated cells are preferentially killed and removed, relatively hypoxic cells are brought in closer proximity to blood vessels and reoxygenated.
In general, cells in the M and G2 phases are the most radiosensitive, and those in late S phase are the most resistant. Prior to irradiation, malignant cells are present in all phases of the cell cycle. Following exposure to radiation many cells are arrested in late G2 phase, thereby synchronizing cells in a more radiosensitive phase.
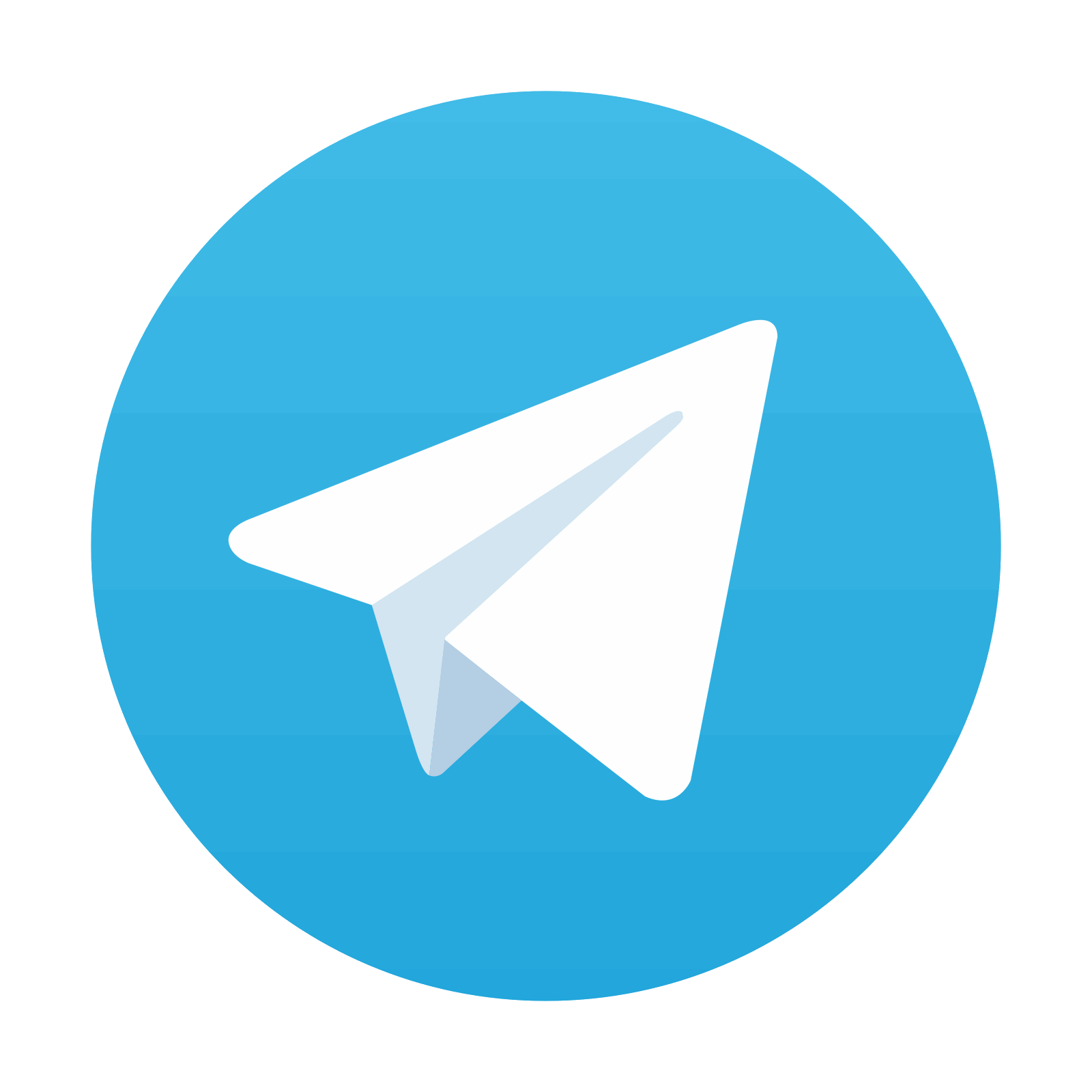
Stay updated, free articles. Join our Telegram channel

Full access? Get Clinical Tree
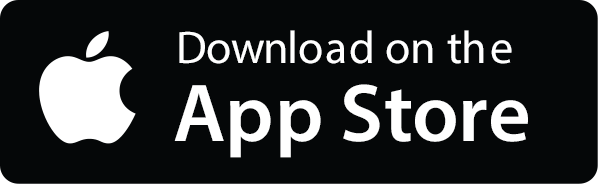
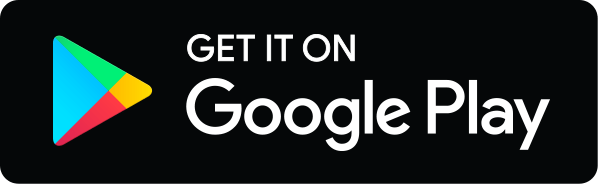