Chapter 101 Principles and Techniques of Vitreoretinal Surgery
Vitreoretinal surgical anatomy
The vitreous can be considered as a three-dimensional matrix of collagen fibers and hyaluronic acid gel (Fig. 101.1). In the normal state, the outer surface of the vitreous is in contact with the retina, pars plana, and ciliary body in a roughly spherical shape with an anterior facet for the lens. Disease-induced RPE and glial cell migration, attachment of cells to the extracellular matrix and hypocellular contraction of the collagen matrix take place with the majority of the relevant changes occurring at the cortex surface. The anterior vitreous cortex (AVC) is contiguous with the posterior vitreous cortex (PVC) and, for the most part, is nonfenestrated.
Mechanics of vitreoretinal surgery
Peeling
Force along the axis of a collagen fiber bundle causes non-elastic collagen fibers to slightly stretch and ultimately to fail. Damage to attached structures is a function of the number of fibers and the strength of the attachment and the substrate. Membrane peeling requires force perpendicular and tangential to the retina, which causes failure of the attachment at the vitreoretinal interface by elongation. Because dense, highly adherent membranes such as those associated with diabetic traction retinal detachments are approximately 100 times stronger than the retina, retina surface defects or retinal breaks usually occur before removal of the membrane occurs (Fig. 101.2). For this reason, membrane peeling is inappropriate in diabetic traction retinal detachment cases.
Shear
Shear cutting occurs when force is applied along two opposing parallel edges moving past each other. Vitreous cutters and scissors use shearing to cut tissue. Inclusive shears such as a vitreous cutter prevent the push-out force that occurs as exclusive shears (scissors) close (Fig. 101.3), pushing the tissue away from the fulcrum, but require elastic deformation of tissue into the port caused by transport pressure gradient (Fig. 101.4).
Infusion system management
Vitrectomy surgeons have occasionally experienced excessively low intraocular pressure (IOP) during vitreoretinal surgery throughout the history of vitreoretinal surgery. Gravity-fed infusion systems were simplistic and could only cause low intraocular pressure if the bottle was too low or the infusion fluid was depleted. Sutured 20-gauge (G) vitrectomy resulted in low intraoperative IOP if the cannula was initially positioned into the suprachoroidal space without surgeon recognition or displaced intraoperatively thereby causing suprachoroidal infusion. Digital display of infusion driven by an air pressure source in the machine created the false impression that IOP was controlled when in fact as much as 25 mmHg of difference existed between infusion pressure and IOP with typical flow rates during core vitrectomy and fragmenter use. The fragmenter lumen, unlike the vitreous cutter is not obstructed by an inner needle or port opening and closing. Low intraoperative IOP is prevented by using a sufficient infusion pressure to compensate for infusion line and cannula resistance-based losses. Ohm’s law of fluidic resistance; pressure = flow × resistance, explains pressure drop in the infusion system during flow. There are many causes of excessively low intraocular pressure during vitrectomy; each of these will be discussed. Inadvertent suprachoroidal infusion is a relatively common cause of low pressure as well as other more serious complications during vitrectomy with 23G and 25G surgery as well as the 20G sutured systems used for over three decades. Sutureless 25G vitrectomy initially utilized straight-in trocar cannula trajectories to produce sclerotomies perpendicular to the sclera. When 23G, sutureless surgery was introduced subsequently, oblique trocar-cannula entry was utilized in order to construct a scleral tunnel to reduce wound leakage. Initially, surgeons used a two-plane approach; the initial trocar-cannula insertion segment was approximately 30° relative to the sclera and the second segment trajectory perpendicular to the sclera. Some surgeons even believed that a biplanar incision was constructed, although this is not true because the scleral tunnel was created before changing the trajectory. More recently, surgeons using both 23G and 25G systems have switched to oblique entry in order to create a long scleral tunnel1–3; unfortunately some surgeons use excessively steep angles (5–10°). Although near tangential entry creates a long scleral tunnel; it increases the chances of infusing into the suprachoroidal or subretinal space (Fig. 101.5). If the cannula is in the suprachoroidal space, the peripheral choroid, which is not observed by the surgeon, expands early in the case allowing infusion without hypotony, later the choroid can no longer expand and infusion becomes limited, alerting the surgeon to the problem (Fig. 101.6). Thinking that hypotony caused a choroidal effusion leads to incorrect management. A single plane, 20–30° trajectory is better compromise between the benefits of a long scleral tunnel and the catastrophe of suprachoroidal infusion. Inspecting the infusion cannula with the operating microscope after insertion and before initiating infusion was standard practice with sutured 20G vitrectomy. Many surgeons have discontinued this practice since 23/25G sutureless vitrectomy began; clearly the crucial step of observing the tip of the infusion cannula must not be omitted. It is best practice to insert the infusion port in the cannula with the infusion running to prevent bubbles followed by immediate inspection of the tip of the infusion cannula. The naked eye and endoilluminator provide insufficient magnification to make the determination that the cannula has penetrated the choroid and non-pigmented pars plana epithelium; microscope visualization is essential. Adhesively fastening the infusion cannula tubing and associated stopcock(s) and connectors to the drape is imperative to prevent traction on the infusion cannula and the eye. Unrecognized pulling on the tubing by the assistant or surgeon can easily cause the cannula to partially pull out causing a suprachoroidal infusion. Adhesively fastening the infusion cannula tubing to the drape with eye in the primary position with a short tubing loop can result in a suprachoroidal infusion when the eye is rotated to view the periphery creating tension on the cannula. Scleral depression is another cause of inadvertent suprachoroidal infusion by causing torque on the cannula as the eye is rotated by the depressor. In addition, scleral depression can force blood clots, dense scar tissue, peripheral vitreous, or silicone oil into the infusion cannula and tubing, effectively plugging it, giving the false impression of infusion system failure. Placing the infusion cannula too close to the lower lid rather than just inferior to the horizontal meridian is a common cause of suprachoroidal infusion created when the eye is rotated down to visualize the inferior periphery and the cannula is rotated into the suprachoroidal space. Kinking of the more flexible silicone tubing terminal segment of the infusion cannula can be caused by the surgeon or assistant accidentally pulling on the tubing. This problem is exacerbated by using excessively low infusion pressure settings (10–25 mmHg) insufficient to straighten out the tubing kink. The author has always used 45 mmHg except when operating on children or patients with very low systemic blood pressure, typically under general anesthesia. Some surgeons have recently advocated using infusion settings of 10–20 mmHg because of a completely unfounded belief that occult ischemia is common during vitrectomy. Using infusion settings of 10–20 mmHg causes miosis, bleeding, and corneal astigmatism from contact lens pressure on the cornea and instrument forces on the sclerotomies as well as scleral infolding often mistakenly thought to be choroidals. Kinking as well as multiple bubbles in the infusion line increase resistance to flow and cause pressure drop ultimately resulting in excessively low IOP. Surgeon remediation of intraoperative low IOP should be systematic; the first step is to inspect the cannula with the microscope to make sure it extends all the way through the choroid and non-pigmented ciliary epithelium. If not, a 25G MVR blade can be used to incise the tissue covering the cannula while pressing the cannula into the eye with smooth forceps (Fig. 101.7). Another option is to move the infusion system port to the supranasal cannula. The infusion tubing should be examined for kinking or inadvertent disconnection. Kinking is most common when excessively low infusion pressure settings are used and the tubing bends at the fluid–air stopcock/valve. If a suprachoroidal infusion occurs, infusion through a cannula with a 25G needle (if 25G surgery) into the middle of the eye will compress the choroid against the sclera and cause the suprachoroidal fluid to disappear often via egress around the cannulas (Fig. 101.8). Cut down drainage is never necessary. If choroidals are present at the inception of surgery, a 6 mm instead of 4 mm cannula can be used and/or infusion initiated with a 25G needle as described above.
Vitreous cutter considerations
All current vitreous cutters utilize suction and inclusive shearing. Ideal tissue cutting is defined as that producing zero displacement of the tissue to be removed and no vitreoretinal traction. It is safest to use the lowest suction force sufficient to imbricate tissue or vitreous into the cutter port. High cutting rates (≥5000 cuts/minute) increase port-based flow limiting and thereby decrease pulsatile fluid flow and pulsatile vitreoretinal traction (Fig. 101.9). Port-based flow limiting via high cutting rates also limits fluid surge after sudden elastic deformation of dense ERM, scar tissue or lens material through the port. Dual actuation eliminates the spring, which facilitates higher cutting rates and enables duty cycle control (Fig. 101.10). In summary, using the highest available cutting rate is the best approach for all tasks and all cases unless all the vitreous has been removed first.
Microscope requirements
All power and control sources for surgical tools should be integrated into a single system for greater efficiency. Advanced vitreoretinal surgery systems have a unified human–machine interface combining all surgical functions into an integrated system (Fig. 101.11). Illumination, diathermy, and infusion are referred to as global functions and are always available. Infusion is best controlled by digital, sensor-based, pressurized infusion systems.
Surgical steps
Transconjunctival, small gauge vitrectomy
Transconjunctival, sutureless 23/25/27G vitrectomy systems have improved to the point that in general, there is no longer a need for 20G vitrectomy; referring to 20G vitrectomy as the “gold standard” is, in the author’s opinion, now arcane. The advantages of 23/25/27G are improved safety because of fewer iatrogenic retinal breaks, reduced operating times, less patient discomfort, and faster visual improvement.4–6 A single 20G sclerotomy is required to remove intraocular foreign bodies and for a fragmenter to remove dense lens material (Fig. 101.12).
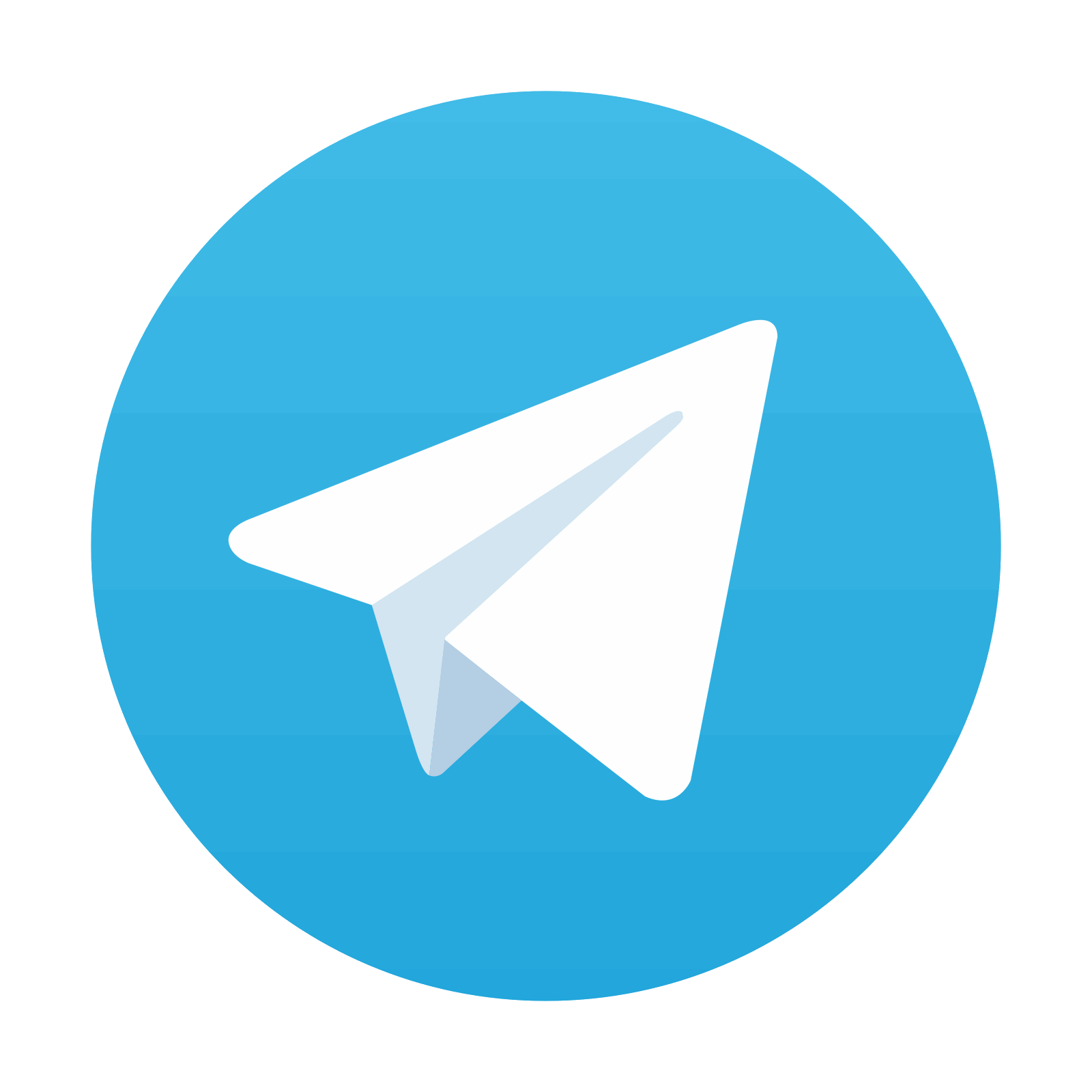
Stay updated, free articles. Join our Telegram channel

Full access? Get Clinical Tree
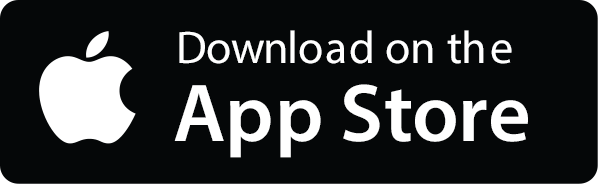
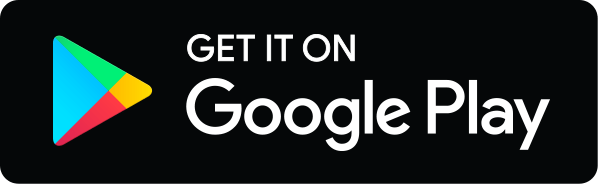