Physiology of the Lens
George Duncan
I. Michael Wormstone
Cataract has been a recognized debilitating visual condition since Roman times and has been the subject of intense investigations by lens anatomists and physiologists for centuries.1 David Brewster2 was the first to recognize the importance of investigating cell structure in the lens. He used novel optical diffraction techniques, using a candle as a light source, to calculate very accurate values not only for fiber diameters in different regions of the lens but also pointed out the presence of ball-and-socket junction decorating the lateral faces of the fibers. Brewster also was the first to suggest that osmotic swelling played a role in cataract.3 Brewster’s interests are still reflected today, although his candle has been replaced by the laser and electron microscope and his simple swelling manipulations by cataract-inducing transgenic technology. Great advances have been made in the past few years on how the cells within the lens are structured and organized, particularly in the role of the cytoskeleton and adhesion molecules in fiber cell movement4 and the role of cell-cell junctional communication in the maintenance of lens homeostasis5 and in cataract.6 Osmotic regulation of the lens also is of prime interest in cataract development, and calcium, pH, and second-messenger systems all play a role.7
It has long been appreciated that the lens continues to grow throughout life, and strenuous efforts also have been made to identify the various factors controlling this very well-regulated process (see growth factor section).8 It has become increasingly recognized in the past few years that lens cells continue to grow after modern extracapsular cataract extraction, and it is this growth that is critical to the development of posterior capsule opacification (PCO).9 Because a search for the factors modulating this type of growth can give insights into how lens growth in general might be controlled,10 this chapter deals with the congruent issues of normal lenshomeostasis, cataract, and PCO. Furthermore, because it is becoming increasingly obvious that many of the factors playing a critical role in human lens behavior do not always have a similar role in the animal lens, this chapter presents, as far as possible, data obtained from human lens investigations. Because this is not always possible, the species origin is given and the data evaluated in terms of known differences in behavior with the human lens.
LENS STRUCTURE AND FUNCTION
The lens is an asymmetric structure bounded on the anterior surface by a single layer of epithelial cells and on the posterior surface by fiber cells (Fig. 1). This apparent asymmetry initiated a number of double-chamber experiments that appeared to show a translens (posterior to anterior) flux of sodium ions correlated with a translens short circuit current.11,12 Studies such as these have given rise to the “pump-leak model” of lens transport. However, more recent studies performed using a vibrating probe to measure radiating currents13,14 have shown asymmetries that are more marked between the equator and the two poles than there are between the two poles themselves, and this has given rise to the “internal flow model” for the lens.5
There is little doubt that the membranes of the anterior epithelial cells have a very different array of channels and pumps than the fiber cell membranes. This fact, allied to an asymmetric distribution of internal gap junctions that seem to favor an internal flow of current toward the equator rather than from posterior to anterior, makes the two models difficult to reconcile.5 However, if an amalgam of the two asymmetry models is considered, then it is possible to merge the two separate ideas into one working hypothesis. The model is presented here and the evidence scrutinized later.
It is suggested that the lens consists of two symmetric and superimposed structures that are only intimately connected at the equator. In this model (see Fig. 1), the anterior epithelium and dynamic bow cells represent one system and the mature fiber cells another. The cells within each system are known to be in good electrical communication with other cells within the same system,15,16 but it is likely that good communication between the systems exists only at the bow region.17 If we also assume that the resting potential of the epithelial cells, on average, is higher than the mature fiber cell membrane potential (again on average), then current flows in at the fiber cell membranes and out through the epithelial cells. If the anterior epithelial layer is assumed to act as a leaky epithelium with little potential difference between the apical and basal surfaces, then the only net current that is observed is inward at the poles and outward at the equator. Furthermore, when the anterior and posterior surfaces are isolated, then the anterior epithelium lies in series with the rest of the lens and a small voltage difference (the net transepithelial voltage) wouldbe expected to be observed. In fact, using an oil to isolate the two surfaces of the bovine lens in a nondisruptive manner, Duncan and colleagues18 observed a maximum potential difference of + 6.5 mV (anterior positive). It should be noted that if the anterior epithelial cells were well-connected to the whole mass of underlying fibers, then a much greater asymmetry in polar currents would be expected than that actually observed as the high voltage of the anterior face membranes would give a strong outward current across the whole of the anterior surface.
INTERCELLULAR COMMUNICATION IN THE LENS
It should be noted that there are two types of intercellular communication possible: electrical and molecular (or metabolic). It has been shown in several systems, including the lens, that metabolic communication can be severed while ionic communication remains intact.16 This important point should be borne in mind when picking one’s way through the mine field of lens junction literature. It also should be remembered that there are possible species differences in the extent of communication between different regions of the lens and, furthermore, the pattern of communication can change as the lens develops.
The molecules (connexins) that form junctional complexes have been reviewed in detail recently,5,19 and only a brief summary is given here. Connexin 43 is the major junctional molecule in epithelial cells, whereas there is a much greater heterogeneity of connexins found in fiber cells, including connexins 46 and 50, although the latter is not found in mature fibers. Interestingly, connexin mutations can give rise to congenital cataracts,5 showing the importance of junction communication to the normal function of the lens. There is general agreement among lens physiologists that the fiber cells arecoupled electrically and that all epithelial cells are extremely well coupled, both electrically and metabolically.5,16,17,20 There is not the same degree of uniformity of opinion concerning the metabolic coupling between epithelial and fiber cells.17,20,21
FIBER-FIBER CELL COUPLING
Duncan15 first pointed out, from evidence obtained by inserting current-passing and voltage-measuring electrodes into the lens, that fiber cell membranes in the bulk of the lens did not present a great resistance to the passage of current within the lens. However, fiber cell membranes at the surface appeared to have a considerable resistance. This appears to be the case in every vertebrate lens so far investigated,5,15 although it should be pointed out that in the invertebrate lens, the equivalent fiber-like structures in the anterior portion of the lens are not coupled to the fiber plates in the posterior hemisphere. Interestingly, the fibers in both hemispheres are well coupled to their neighbors within the same hemisphere.22
The low internal resistance view was further refined by Eisenberg and colleagues23,24 who quantified the internal resistance and showed that it was not homogeneous, but was, in fact, lower in the equatorial regions in the cortex. This conclusion is satisfyingly in agreement with the distribution of gap-junctional complexes within the lens. They are found in much higher density in the equatorial regions.17,20
Electrical communication is so efficient within the lens that electrical signals arising from the activation of certain receptor systems can be coordinated so that the whole lens can be seen to oscillate. This pattern has been observed in the whole lens25 and in a lens clamped in a double-chamber system.26
Electrical communication also can be modulated or controlled, and lowering internal pH or increasing internal calcium has been shown to increase internal resistance.27,28,29 In the latter case, the uncoupling process is calmodulin-dependent.27 Mathias and coworkers28 pointed out that the nucleus of the amphibian lens does not uncouple even although the internal pH is reduced to very low values. This is interesting in view of the latest findings from the chick lens, in which it has been shown that the nuclear fibers are completely permeable to the passage of very large molecules.30 Duncan31 also has pointed out previously that the central regions of the lens contain relatively high levels of lysophospholipids32 and suggested that the nuclear fiber membranes were degenerate in some way. This, he argued, would explain why nuclear cataracts are homogeneous defects and involve always the whole nucleus whereas cortical cataracts that involve an increase in internal calcium can be localized and can involve only small, specific regions of the lens.33
Ionic coupling within the anterior epithelium is extremely efficient and although the calculated intercellular resistance of 25 Ωm17 is much higher than that of aqueous humor (1 Ωm), it is considerably lower than the value calculated for the fiber-fiber resistance (90 Ωm) in the bulk of the lens.24 It is interesting in this respect that ionic coupling in the bulk of the lens can be greatly reduced by conventional uncoupling agents such as octanol and low pH,27,28,29 whereas ionic coupling across the epithelium is relatively resistant to such treatment.16
There is only one report of ionic coupling between epithelial cells and fiber cells,34 but the model suggested in Figure 2 would allow for current injected into the fibers to flow into the epithelium, even if communication only existed between equatorial epithelia and underlying fibers. The space constant (λ) for the epithelium extends some 20 cell diameters,17 and so little decrement of voltage would be expected across the whole epithelium for currents injected into fiber cells anywhere in the lens.
METABOLIC COUPLING IN THE LENS
The only noncontroversial statement in this area of lens research is that there is little agreement about the extent and nature of fiber-fiber or fiber-epithelium coupling. This lack of agreement may reflect species differences or the fact that some areas of the lens are better connected than others. Often, when the same experimental protocols are performed on lens cells from different species, different results are obtained. For example, when fluorescent dye is injected into a cultured chick lentoid body (expressing MIP26), then it spreads rapidly from the injected site into neighboring cells,35 while in a rat lentoid (similarly expressing MIP26), the dye remains within the injected cell.36 In the embryonic chick lens, dye injected into the posterior lens rapidly spreads throughout the lens and passes from fibers to epithelium.37 These communicating pathways are quite sensitive to low pH at early stages, but beyond stage 23, low pH could not prevent the flow of dye between fiber cells nor from fiber cells to epithelium.37 In amphibian and rat lenses, however, even ionic coupling between fiber cells is very sensitive to low pH.28,29 Furthermore, in these latter species, heroic efforts have to be made in terms of intrusive dissection of the anterior capsule and application of very-high-sensitivity imaging techniques to observe any flow between anterior epithelial and fiber cells.21 In the end, the authors of this latter study concluded that at most, one in four anterior epithelial cells are metabolically coupled to the underlying fibers. In fact, in humans, rats, and amphibians, there appear to be few junctional complexes between epithelial cells and underlying fibers, except, as has been mentioned above, in the equatorial region.17,20,38
Just as there are regional variations in electrical coupling in the bulk of the lens, there also are variations in the extent of metabolic coupling. Epithelial cells near the bow appear to be in communication with newly formed fiber cells,21 whereas short fiber cells that are actively elongating do not appear to pass dye efficiently to their neighbors and do not possess many gap-junctional plaques.17 However, longer, more mature, fibers with interdigitating junctions did pass injected dye efficiently to their neighbors.17,21 These fibers also possess abundant junctional complexes. Fibers that are more mature still and extend from the anterior to posterior pole do not appear to be metabolically coupled to their neighbors nor were they coupled efficiently to the anterior epithelial cells.17 Again, this behavior was in agreement with the lack of typical gap-junctional arrays in the membranes of these fibers. On pro-gressing to the nucleus, the fiber cell membranes appear to degenerate so that they do not impede the diffusion of very large molecules,30 at least in the chick, and neither can nuclear cells be uncoupled in the amphibian lens by low pH.28
PERMEABILITY PROPERTIES OF THE LENS MEMBRANES
The permeability properties of the lens can be measured by a number of different methods including radiotracer techniques to assess directly the passage of ions and molecules and electrophysiologic techniques both to assess the overall electrical conductance of the lens but also to investigate the specific channel mechanisms involved. In the latter case, it is emerging from patch-clamp experiments that the lens possesses a remarkable variety of potassium, sodium, chloride, and calcium channels. The different methods used to identify the various channels have been reviewed recently in a number of publications.5,39,40 This review deals rather with the overall changes in lens conductance that occur with age and on exposure to oxidation, but it also deals with the changes that occur after the activation of certain receptors. It also should be remembered that ions may traverse membranes by a number of electrically silent systems, and they include the Na+ /H+,Na+ /Ca2+ HCO3-/Cl- exchange mechanisms as well as active transporters such as Na-K-ATPase. The movement of certain ions and even water itself also is restricted by interactions with other species within the lens, and this has to be taken into account in the overall picture of movement across the outer barriers and through the internal matrix of the lens. In the human lens, for example, although almost 100% of K+ ions are in free solution, ion-sensitive microelectrode measurements have shown that approximately 50% of internal sodium is complexed, whereas more than 99% of the total calcium is so tightly bound or sequestered that it does not contribute to the measured activity.33,41 Interestingly, a large fraction of the calcium that enters during cortical cataract also finally resides in the lens in a complexed form.42,43
It is now generally accepted that the main ionic permeability barriers lie at the outer surface of the lens, whereas the inner fiber membranes present less of a barrier to the flow of ions because adjacentfibers are coupled through gap junctions or become fused or “degenerate.”5,15,31 The relative permeability of the different ionic species can be computed by fitting the Goldman equation44 to the membrane potential and (Em) ionic data:
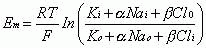
where α = PNa/PK and β = PCl/PK. In most lensesso far studied, PNa <m25> PK, while PCl ≈ PK. In the amphibian lens, the relative permeabilities obtained in this way are in good agreement with the individual permeabilities calculated from radioisotope flux data obtained for the individual ions.45 The 42K (or 86Rb) and 36Cl efflux data are easiest to interpret as the major component follows single exponential kinetics.31,45 The 22Na and 45Ca2+ data kinetics are multiexponential in form, presumably because significant proportions of both of these ions are in a bound or complexed form.45,46
ACTIVE SODIUM AND POTASSIUM CONTROL MECHANISMS
Sodium is distributed far from equilibrium in the normal lens and energy must be expended continuously to maintain the normal sodium, potassium, and water content of the lens.31 When the pump is inhibited by ouabain, then the sodium and water content increases and there is some loss of transparency due to localized refractive index changes. Several studies have shown that ouabain is only effective when applied to the anterior surface11,12,18 and, indeed, the largest fraction of Na-K-ATPase activity resides in the anterior epithelium. It should be noted, however, that ATPase activity is found associated with the membranes of all superficial lens cells.47 Interestingly, Western blot studies show that pump molecules are present on all lens membranes, even although activity is only found in those near the surface.47
The Na-K-ATPase pump consists of an α-catalytic subunit with an associated β subunit and is, in fact, a member of a multigene family of proteins.48 Three α subunits (α1, α2, α3) and three β subunits (β1, β2, β3) have been identified so far, and the expression of the three isoforms (the α form in particular) is quite tissue specific. Most kidney cell membranes express α1 and α3. Interestingly, the lens appears to express all three polypeptides in anterior epithelial cells but only the α1 form in fiber cells.49 It further appears that all three isoforms are not expressed homogeneously across the anterior epithelium, with α3 being located at the anterior pole and α1 predominating in the equatorial region.50
Sodium pump activity is under dynamic control itself and more α subunits are found in lenses stressed by exposure to amphotericin, for example. Interestingly, expression of the α2 subunit appears to be preferentially upregulated.51,52 More recently, it has been shown that Na-K-ATPase activity can be modulated by tyrosine kinase (TK) activation, which raises the very interesting possibility thatthis critical pump mechanism is under second-messenger control in the lens.53
SODIUM CONDUCTANCE
The membrane potential of the human lens depolarizes with age (see Fig. 2) and the decline becomes most significant after the age of 50 years.54 Theion concentration and electrical data indicate thatPNa/PK progressively increases and as the measured electrical conductance and free Ca2+ activity also increases, Duncan and coworkers54 have suggested that all of these age-related changes could be explained by the progressive activation of a nonselective cation channel. Several studies have shown the presence of such a channel in a number of lens species.5,55,56 Interestingly, in the amphibian lens at least, this channel can be activated by pressure.56 After the age of 50, the lens becomes increasingly unable to accommodate. This is not because the ciliary muscles cease to function, but rather the lens properties became less elastic.57 The stretching and relaxation energy of the muscles would then be dissipated in the lens, leading to an increase in pressure on the lens membranes after the age of 50. The lens nonselective cation conductance also appears to be activated by oxidation58 and a progressive decline in glutathione content of the lens with age59 would also, therefore, tend to activate such a channel. It should be noted that there is a catastrophic decline in membrane potential in human cortical cataract with a concomitant increase in membrane permeability7,42,60 (see Fig. 2).
Although tetrodotoxin-sensitive sodium-specific conductance channels have been identified in tissue-cultured cells,5 there is as yet no evidence to suggest that neuronal-like sodium channels exist in native human lens cells.
POTASSIUM CONDUCTANCE
Potassium ions are distributed near, or above, equilibrium levels in most lenses so far studied, and there is a huge diversity in the range of channelsthrough which K+ ions can diffuse. Patch-clamp and molecular cloning methods currently are in use to identify the channels that are responsible in thelens for K+ movement.5,39,40 The three types of channels identified by Mathias and his colleagues5 include inward rectifiers, large conductance calcium-activated channels (BK), and delayed rectifiers. The amphibian lens has been most extensively studied so far, and the overall conductance has beenmapped out by current-clamp techniques.61 The I-Vcurves show outward-rectifying properties and the I-V relationship in the normal lens is so steep, it cannot be predicted by Goldman theory. The overall conductance-voltage relationship is best explained by a combination of nonvoltage-sensitive and outwardly rectifying conductances. Removal of external calcium increases the former, largely by increasing Na+ conductance, while the latter is largely inactivated so that the conductance under Ca-free conditions is actually lower than the control conductance at voltages more depolarized than-70 mV. There are significant differences in the behavior of the overall potassium conductances of frog and rat lenses. In the former, the voltage-sensitive conductance is largely inactivated at the resting potential and the conductance is insensitive to blockade by TEA or quinine.62,63 However, on depolarizing by voltage or by adding external K+, the voltage-sensitive conductance is activated and can be blocked by TEA. Interestingly, the voltage-sensitive conductance in the frog can be activated by low concentrations of the nonpermeant SH complexing agent PCMPS. Under these circumstances, the lens voltage hyperpolarizes to near -100 mV, and the conductance can now be blocked by TEA.63 In the rat lens, conversely, the resting conductance is very sensitive to blockage by TEA or quinine.64 Under these circumstances, the K+ conductance also can be blocked by the addition of external Ca2+ and the voltage depolarizes. Hence, although the K+ conductance in the rat lens can be modulated by Ca2+, it is not strictly a Ca-activated conductance but rather an inactivated conductance. Significantly, 86Rb+ efflux from the human lens is reduced significantly by increasing external (so presumably internal) Ca2+ .60 At present, the only potassium channel identified by molecular cloning techniques from native human lens epithelial tissue is the inwardly rectifying channel 1RK1.65 This channel also has been identified in a number of animal lenses.5,39,40
CHLORIDE CONDUCTANCE
Chloride ions are distributed near equilibrium across the lens membranes of a variety of species,31,45,66 and in the amphibian lens, the measured electrical conductance decreases when chloride ions are removed from the external solution.67 The isotopic chloride efflux rates from both amphibian and mammalian lenses are high. In addition, it should be noted that part of this efflux arises from exchange mechanisms and these would not be expectedto contribute to the electrical conductance.66,67 Examples of such processes included the DIDS-sensitive HCO3-/Cl- and the Na+ /HCO3-/H+ /Cl- exchangers66,68 as well as the Na+ Cl-cotransporter.69 Undoubtedly, other exchange systems are likely to exist. Chloride channels have been identified by patch-clamp techniques in both epithelial and fiber membranes70 and are likely to play important roles in both volume70 and pH66 regulation.
WATER MOVEMENT
The cytoplasm of the lens, in common with most animal cells, is isosmotic with the surrounding media.71 However, this is not an equilibrium situation, because of the large concentration of fixed negative charges with the membranes of the lens, and the lens has to expend energy continuously to maintain osmotic equilibrium.72 When the sodium pump is inhibited or the sodium permeability is increased, then the lens swells.31 The water permeability of lens membranes can either be determined by creating an osmotic gradient across the lens membranes and observing the rate of change of volume of the lens, or it can be determined by measuring the efflux of radioactive water. The first measurement determines the hydraulic permeability (Pos) and the second the diffusional permeability (Pd). Both permeabilities have been determined for the amphibian lens and the values are 100 μm-1 and 0.4 μm-1, respectively. These values are remarkably close to the two permeabilities measured in a wide range of cell types,71 and the large difference between the two indicates that much of the water movement can take place through water-filled pores. Water channels are known as aquaporins and lens epithelial cells contain an abundance of these.73 Interestingly, MIP26 is believed to be a very old (and not very efficient) member of the aquaporin family and is termed aquaporin O. When MIP26 is expressed in oocytes, it does, indeed, give rise to a small increase in water permeability and, significantly, in agreement with some other members of the same family, it also acts as a glycerol transporter.74 MIP26 could be termed the Swiss army knife of lens membrane proteins as it appears to have had a very large number of functions ascribed to it.74 The one role it seems to have escaped so far is of a “membrane chaperone” and its huge abundance in the lens might, indeed, indicate such a function!
pH REGULATION
The lens must continually and actively extrude protons for two main reasons. The first reason, in common with most cells, is that the lens has a relatively high negative resting potential and thus there is an inwardly directed electrical gradient for mobile positive charge. For example, with a transmembrane potential of -60 mV and an external pH of the humors of approximately 7.3,29 the internal pH (pHi) at equilibrium would be predicted to be 6.2 from the Nernst equation. The measured pHi from a range of mammalian lens cells (including human) is close to 7,28,29,41 and so protons are not in equilibrium. The second important reason arises from the fact that because the lens is largely an anaerobic tissue, there is a continuous production of H+ from lactic acid.
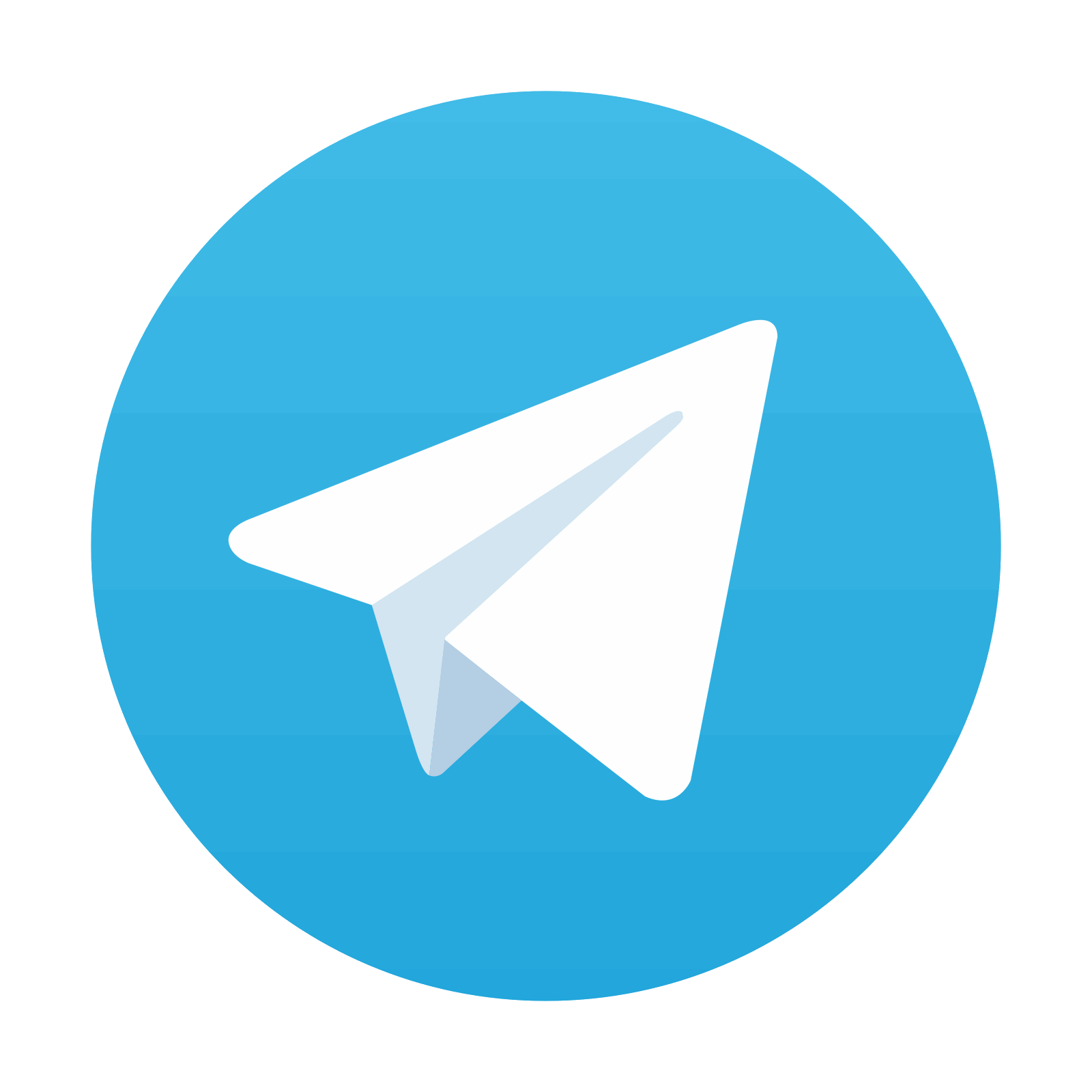
Stay updated, free articles. Join our Telegram channel

Full access? Get Clinical Tree
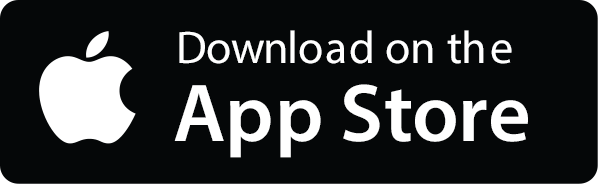
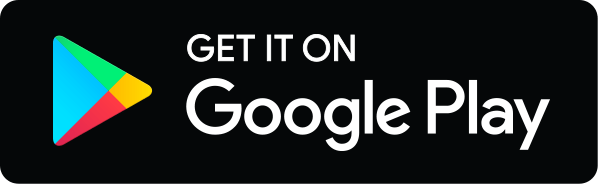