Phototherapeutic Keratectomy
Sumit (Sam) Garg
Ann Z. McColgin
Roger F. Steinert
The argon fluoride excimer laser, emitting ultrashort pulses at 193 nm, precisely etches the cornea with only submicron damage to the adjacent nonablated stroma. The freshly ablated surface supports rapid and stable re-epithelialization in advance of reformation of normal basal lamina complexes. Therefore, the cornea can be recontoured by the excimer laser in vivo. The dominant clinical interest in this laser has been for modification of the corneal optics, particularly for reshaping the cornea for the correction of refractive error. Superficial corneal opacities and irregularities also are treated by the laser as a therapeutic intervention termed phototherapeutic keratectomy (PTK). This chapter reviews the general principles, indications for, and clinical results of PTK.
PRINCIPLES OF THE EXCIMER LASER
The term excimer is a contraction of excited dimer. Excited dimers are molecules with little or no binding in the electron ground state but a more closely bound upper energy state. Rare gas atoms interact with a halogen molecule when stimulated to the upper state by electrical discharge within the laser cavity. High-power ultraviolet (UV) radiation is emitted as the bound upper state rapidly dissociates to the ground state.1,2,3 First developed in 1975,4 the excimer laser is used scientifically to perform research in physical chemistry and pump dye lasers,5 and industrially to etch various materials.6 Table 47-1 lists the UV wavelengths obtained from some common gas mixtures. Excimer laser emission is inherently in short pulses, typically around 10 nanoseconds (ns), with a repetition rate of 1 to 50 Hz.
Table 47-1 Excimer Laser Emission | ||||||||||||||||
---|---|---|---|---|---|---|---|---|---|---|---|---|---|---|---|---|
|
PHOTOBIOLOGY OF EXCIMER LASER CORNEAL ABLATION
Research in the early 1980s showed that excimer laser-generated UV light can precisely etch various polymers.7,8,9 Srinivasan and Leigh6 observed that the irradiated molecules are broken into small fragments that are ejected into the surrounding atmosphere, a process called ablative photodecomposition. Ablative photodecomposition of organic polymers is attributed to the high absorption by the polymer of short UV radiation, confining the effect to near the surface, and the high energy of each UV photon. At 193 nm, a single UV photon has an energy of 6.4 eV, which exceeds the covalent bond strength of many molecules. After bond breakage occurs, intense local pressure in a confined volume ejects the molecular fragments into the surrounding atmosphere.6,9,10,11,12,13,14
Direct bond breakage by a high-energy photon is a photochemical laser–material interaction. The relative contribution of photochemical and thermal mechanisms to the UV ablation of organic polymers is regarded as negligible.15 At the shortest laser wavelengths, such as 193 nm, the high photon energy may result in a purely photochemical process of ablative photodecomposition. At longer wavelengths, absorbed photon energy leads to a local rise in temperature that causes etching through a photothermal process. At longer wavelengths, coagulation of protein may be expected adjacent to the ablation zone. Short laser pulses delivered at a low repetition rate help to limit local heating.
Photochemical and photothermal effects of excimer laser wavelengths on the cornea result from absorption by solid elements. Water poorly absorbs wavelengths between 193 and 293 nm.16 The carbon–nitrogen peptide bond is believed to be the source of a strong protein absorption peak at approximately 190 nm.17 Most of the corneal amino acids are nonaromatic, and they account for collagen absorption that begins to rise at wavelengths of less than 260 nm and particularly less than 240 nm.18,19 Aromatic amino acids absorb more strongly at wavelengths of greater than 240 nm. A collagen absorption peak around 250 nm is attributed to a carbon–nitrogen enolized peptide linkage.17 The corneal glycosaminoglycans have similar absorption spectra, with peaks around 190 nm and minimal absorption at 248 nm.20 Nucleic acids are limited to the occasional keratocyte in the stroma but are more important chromophores in the epithelium, with strong absorption at both 248 and 193 nm.21,22 Ascorbic acid, particularly concentrated in epithelial cells, has more absorption at 248 nm than at 193 nm.23
Mutagenesis and carcinogenesis are concerns with UV radiation. Almost all carcinogens have been shown to be mutagens.24 UV radiation-induced mutation parallels absorption by DNA.19 The low density of stromal keratocytes offers some protection against carcinogenesis resulting from stromal photoablation. In several studies, 193-nm irradiation did not cause mutagenic or carcinogenic cellular events. Nuss et al25 examined unscheduled DNA synthesis, a measure of repair of pyrimidine dimers. Compared with a control incision made with a diamond knife, unscheduled DNA synthesis did not increase after 193-nm linear ablation; in contrast, a statistically significant increase occurred after 248-nm irradiation. Possible mechanisms for the decreased toxicity at 193 nm include absorption of that wavelength by protein surrounding the nucleus (a protein shield), lack of cytotoxicity of DNA photoproducts produced by 193-nm light, DNA damage that can readily be repaired by the cells, or such lethal damage that potentially mutagenic repair processes are not possible.26 In a skin model, DNA damage and subsequent cytotoxicity were least at 193 nm, intermediate at 308 nm, and greatest at 248 nm.
Corneal irradiation at 193 nm also results in fluorescence between 295 and 425 nm.27 These emissions could be both mutagenic and cataractogenic; however, the highly attenuated energy of the fluorescence may not reach toxic levels.
LASER–CORNEA INTERACTION
In 1983, Trokel et al28 first reported the precise and controlled etching of the cornea by an argon–fluorine (ArF) excimer laser. Puliafito et al29 compared the histopathologic effects of linear cornea ablation at 193 and 248 nm. Both studies found excellent preservation of normal corneal stromal microstructure adjacent to the ablation zone at 193 nm (Fig. 47-1). The adjacent cornea remained optically clear. High-power transmission electron microscopy showed a submicron zone of electron density immediately adjacent to the ablation only. Kerr-Muir et al30 first described a pseudomembrane that appears to seal cells and cellular nuclei transected by the laser beam. In contrast, at 248 nm, disorganization of the collagen microstructure extended into the adjacent stroma for more than 10 νm. The cornea immediately adjacent to the ablation showed a loss of transparency, which was indicative of thermal injury. Furthermore, a study by Peyman et al31 showed a significant coagulative effect from 308-nm excimer laser radiation with induced corneal necrosis, stromal opacification, and endothelial cell damage.
![]() Figure 47-1. Light micrograph of a 193-nm, slitlike ablation in a bovine cornea. Dosage parameters: 20,000 pulses, 50 Hz, 125 mJ/cm2 per pulse, with a 10-νm mask (original magnification ×214). (Reproduced with permission from Puliafito CA, Steinert RF, Deutsch TF et al: Excimer laser ablation of the cornea and lens: Experimental studies. Ophthalmology 92:741, 1985.) |
Krueger et al32,33 quantitated ablation rates. Etch depth per pulse plotted against radiant energy density generated sigmoid curves. The steep portion of the curve is approximately logarithmic; this finding corresponds to observations in simpler organic polymers. The inflection point of the curve is approximately 200 mJ/cm2 for 193 nm, which represents the energy density yielding the most efficient ablation. Puliafito et al34 postulated that ablation at the inflection points may be clinically undesirable; at the inflection point small changes in laser output could alter the ablation rate dramatically. Ablation at higher energy densities, such as 400 to 600 mJ/cm2 per pulse, where the ablation rate has the flattest slope, might be preferable in situations where ablation rate is critical. Krueger et al33 further observed that for wavelengths higher than 193 nm, the threshold for corneal ablation increases as the laser repetition rate decreases. At 193 nm, the threshold was constant despite varying repetition rates. This observation is consistent with the photochemical theory of excimer corneal ablation, in which the buildup of heat does not play an important role at 193 nm and thermal mechanisms of ablation are important for ablation at longer wavelengths.
The effect of radiation density on corneal smoothness remains controversial. Fantes and Waring35 showed improved corneal surface uniformity with their scanning system at higher laser energy density, especially with 500 mJ/cm2 per pulse. In contrast, Berns et al36 observed that such high levels of energy can be associated with ridges on the corneal surface, damage to the endothelium, and the formation of small pits on the stromal surface inside the linear ablation zones.
Endothelial toxicity probably is minimal, although endothelial effects can be seen despite the nearly complete absorption of 193-nm photons within 1 microns of tissue. Dehm et al37 found that in linear ablations, 193-nm incisions to 90% of corneal depth produced endothelial membrane disruption similar to that seen underlying diamond knife incisions at the same depth. Frank loss of endothelial cells underlying the incision was observed at 248 nm. Hanna et al38 observed the appearance of electron-dense granules in the endothelium after surface ablation of rabbit corneas at 193 nm. These electron-dense granules migrated through the Descemet membrane over several weeks and then dissipated. The clinical significance of this observation is unknown. This phenomenon may be related to transient pressure injury to the endothelium. Zabel et al39 measured pressures as high as 100 atm at the endothelium during ablation of the superficial stroma, but no frank disruption of the corneal endothelium occurred.
The fragments ejected from the corneal surface are visible several hundred nanoseconds after the laser exposure. For 193-nm irradiation, the particles travel at an initial velocity of approximately 400 m/s for the first 500 ns but then rapidly decelerate. The ejection of the particles ends within 5 to 15 νs as the decelerating particles form a mushroom-shaped cloud (Fig. 47-2).40 Increased exposure energy leads to increases in both the plume size and initial velocity of the ejected fragments. Analysis of the plume has identified numerous molecules that contain between 10 and 20 carbon atoms.41
![]() Figure 47-2. Ablation plume created by 193-nm excimer pulse at 900 mJ/cm2 per pulse. High-speed photograph obtained by illumination with Nd:YAG laser at 532 nm 50 ms after the excimer pulse struck the corneal surface (original magnification ×4.5). (Reproduced with permission from Puliafito CA, Stern D, Krueger RR et al: High-speed photography of the excimer laser ablation of the cornea. Arch Ophthalmol 105:1255, 1987.) |
THERAPEUTIC APPLICATIONS OF THE EXCIMER LASER
The excimer laser has received much attention for its ability to reshape the corneal surface to correct ametropia. Although correction of postoperative refractive errors and anisometropia has been called therapeutic, PTK typically refers to the use of the excimer laser for the treatment of other corneal disease. This section discusses the application of excimer laser ablation for recurrent erosions, treatment of surface irregularities, removal of superficial opacities, and treatment of complications after photorefractive keratectomy (PRK) and laser in situ keratomileusis (LASIK).42 PTK has been used successfully in pediatric as well as adult diseases.43,44
RECURRENT CORNEAL EROSIONS
Painful recurrent erosion syndrome, whether resulting from trauma or anterior membrane dystrophy (Cogan dystrophy or map-dot-fingerprint dystrophy), results from abnormalities in the epithelial basement membrane complex. Most of these patients respond to topical lubrication therapy, hyperosmotics, bandage soft contact lenses, debridement of the epithelium and basement membrane, or anterior stromal micropuncture. An occasional patient continues to have painful recurrent erosions despite all these measures. Superficial PTK can be curative in that setting. The ablated anterior corneal surface appears to be highly supportive of stable re-epithelialization. Although completely normal reformation of the basal lamina complex, including normal density of hemidesmosomes and anchoring fibrils, may take months to years, most investigators have been impressed with the rapid and stable re-epithelialization that occurs after ablation, with absence of punctate keratitis, staining defects, or symptoms of recurrent erosion.
The objective of PTK in the treatment of recurrent erosions is simply to remove enough of the superficial Bowman layer to permit formation of a new basement membrane with adhesion structures; therefore, it is the most superficial of the PTK procedures. The usual technique is to debride the loose epithelium manually. In most cases, even if the area of clinical recurrent erosion is localized, the surgeon finds that the epithelium is poorly adherent over most or all of the corneal surface. With the use of a large spot size, such as 6.5 mm, the surgeon ablates the central superficial corneal surface.
Depending on the laser, varying techniques are used to expose the peripheral cornea outside the central zone. For example, with the VISX laser, a smaller spot is manually scanned around the periphery by moving the patient’s head. With some smaller spot scanning lasers, the periphery can be treated by programming the scan to include these areas. van Westenbrugge45 showed small-spot PTK using the NIDEK EC-5000 excimer laser to have an 80% success rate in a retrospective analysis of ten consecutive eyes.
The amount of tissue to be ablated to maximize the effect with minimal refractive change has not been elucidated. Some investigators remove only 2 to 6 microns,46,47,48,49 but others have found that removal of 10 microns results in fewer recurrences.50 After the laser ablation, a drop of topical antibiotic, steroid, and sometimes nonsteroidal agent is applied, followed by placement of a bandage contact lens. Close follow-up is required until epithelialization is complete, at which time the contact lens is removed. Patients typically are instructed to continue aggressive lubrication, including nightly application of lubricating ointment, for several months after the procedure.
The results of clinical studies of PTK for recurrent erosions have been encouraging. Morad et al46 treated 23 eyes for recurrent or persistent corneal erosion with PTK by ablating 2 to 6 microns of tissue after epithelial removal. Eighty-three percent of patients remained free of symptoms during the 12- to 60-month follow-up, and none experienced a significant refractive change. Cavanaugh et al51 treated 36 eyes for recurrent erosion in the setting of anterior basement membrane dystrophy and found that 86.6% had no recurrence of symptoms after 12 months of follow-up. The average change in refraction was +0.72 ± 1.26 diopters (D), and a positive correlation was found between the number of pulses applied and induced refractive change. Jain and Austin47 reported that of 77 eyes treated with PTK for recurrent erosions, 69% were free of all symptoms after 6 to 55 months of follow-up and 100% were free of acute episodes after one or two treatments. It also was found that patients treated for recurrent erosions resulting from trauma had a better outcome than those whose symptoms were idiopathic or caused by a basement membrane dystrophy. Other studies have also shown higher rates of success with PTK in recurrent erosion syndrome due to trauma compared to corneal dystrophies.52,53 Several other studies have shown a 60% to 100%46,47,54,55,56,57,58,59,60 cure rate with one or more PTK treatments for recurrent erosions. Quantitative studies indicate that the fundamental health of the corneal epithelium may be improved after PTK.61
More studies are necessary to determine the optimal amount of tissue to be removed to minimize both recurrences and significant refractive changes. Sridhar et al62 found that diamond burr polishing was at least as effective although simpler and less costly than PTK for recurrent erosions. Likewise, a study from Germany concluded that intraepithelial PTK and alcohol delamination of the epithelium were both effective for trauma=related recurrent erosions where ablation of the Bowman layer or anterior stroma does not appear to be necessary.63
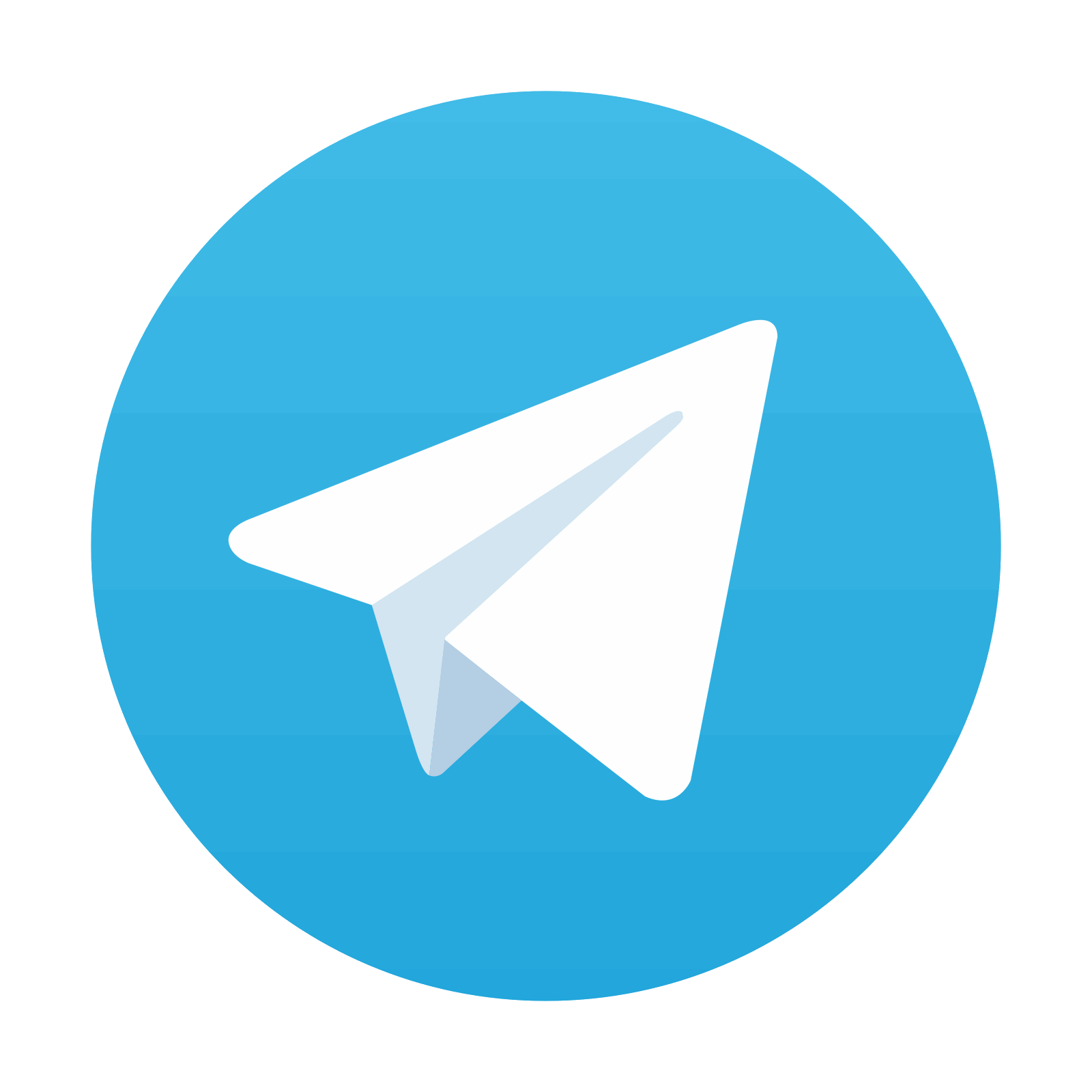
Stay updated, free articles. Join our Telegram channel

Full access? Get Clinical Tree
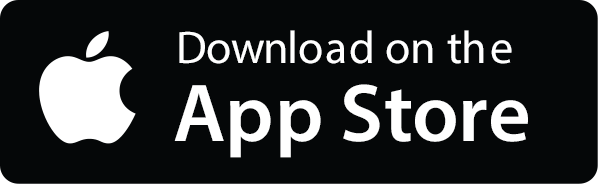
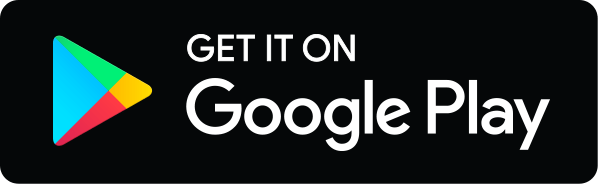