Pharmacology of Local Anesthetics
Joel S. Mindel
HISTORY
Local anesthesia became a reality with the introduction of cocaine. Cocaine is an alkaloid found in coca bush leaves and first isolated in 1862. A Viennese ophthalmologist, Karl Koller (1854–1944) discovered the anesthetic effect of cocaine in 1884. He had been encouraged by Sigmund Freud to study the effect of cocaine on muscle strength. Koller noticed that cocaine numbed his tongue and, realizing that this might make it a topical anesthetic, he placed the drug on the eye. Koller could not afford to travel so he asked Josef Brettner, who was visiting the Vienna Eye Clinic on his way to the Heidelberg Ophthalmologic Congress, to announce his discovery at that meeting on September 15, 1884.1 Subsequently, Koller submitted his paper to Vienna’s Physician Guild on October 17, 1884. He later reported the removal of corneal foreign bodies and the performance of iridectomies and cataract surgery using topical cocaine anesthesia. The 1884 Archives of Ophthalmology contained this evaluation by Knapp: “No modern remedy has been received with such general enthusiasm, none has been as rapidly popular, and scarcely any has shown so extensive a field of useful application as cocaine, the local anesthetic introduced by Karl Koller of Vienna.” Koller emigrated to the United States in 1888 and settled in New York, where he became the first Chief of Ophthalmology at The Mount Sinai Hospital in 1901. He was the first recipient of the Howe Medal of the American Ophthalmological Society in 1922.
It quickly became apparent that cocaine was not the ideal local anesthetic. It had a low therapeutic ratio, which could result in convulsions and deathwhen it was injected for regional anesthesia. It was relatively unstable when stored as a solution, and it was addicting. By the time cocaine was successfully synthesized in 1924, superior local anesthetics had already been developed based on the evolving knowledge of the structure of cocaine (Fig. 1). Cocaine was found to contain an essential ester bond. This was shown by the inactivation of its anesthetic effect when this bond was hydrolyzed. Cocaine deesterification liberated benzoic acid. In 1905 procaine (novocaine) was synthesized; procaine is a benzoic acid derivative with an ester bond (Fig. 2), that is, a paraminobenzoic acid ester of diethylaminoethanol. Other frequently used agents that followed were tetracaine (1930), lidocaine (1946), mepivacaine (1956), and bupivacaine (1963).
STRUCTURE
Local anesthetics act by blocking the conduction of impulses along the neuronal membrane. Many types of chemical structures do so to some degree, including ethanol and barbiturates. However, those agents that are clinically useful as local anesthetics are more potent in this regard and are less toxic. Structurally, their syntheses require a secondary (-NH) or tertiary (-NH2) amino group in the hydrophilic portion of the molecule. With few exceptions, they also contain a lipid-soluble group (e.g., benzoic acid), separated from the water-soluble group (containing the amide) by an intermediate chain. The intermediate chain contains an oxygen, nitrogen, or sulfur atom. This general structure has been called the anesthesiophore group (Fig. 3). It is important that the amino group in the hydrophilic portion of the molecule be a proton receptor and thus be basic, allowing it to become positively charged (e.g., a tertiary amide, -NH2, becomes quarternized, -NH3+, and thereby ionized) at physiologic pH (7.3–7.4).
MECHANISM OF ACTION AND EFFICACY
Local anesthetics block the formation or conduction of action potentials. They stabilize the neuronal membrane and prevent the generation of impulses; the standing potential remains intact.
The action potential begins with extracellular sodium flowing into the neuron, followed by intracellular potassium flowing out of the neuron. At low concentrations, local anesthetics primarily affect sodium conductance. At higher concentrations, potassium conductance may also be affected. The clinically used local anesthetics act primarily by reversibly blocking the sodium ion channel at its internal pore.
If a nerve is perfused in a local anesthetic solution at a low pH, causing all the anesthetic molecules to be ionized (quarternized), there is no effect. However, as the pH of the solution is raised toward physiologic levels, the number of ionized molecules is reduced, and a conduction block develops. The explanation is that ionized molecules cannot readily penetrate the sodium pore or the neuronal membrane. The anesthetic agent must be acting on the internal side of the sodium pore or neuronal membrane, not externally.
If the nerve is anesthetized with local anesthetic at pH 7.3 and the pH is markedly raised (e.g., to more than 9.5), the blockade will be reversed. At a high pH, the anesthetic molecules inside and outside the neurons are in the nonionized state. Lowering the pH to 7.3 will restore the conduction blockade. This is consistent with the concept that the active form of the anesthetic molecule must be ionized and positively charged if it is to block the sodium pore or neuronal membrane. Exceptions exist; benzocaine is a local anesthetic, but it is not ionized at physiologic pH.2
There are several other generalizations about the molecular structure and anesthetic activity relationship that seem to hold up fairly well. The larger the alkyl substitutions on the nitrogen atom of the hydrophilic group, the greater the anesthetic activity; lengthening the intermediate chain increases activity and toxicity; the most effective substitutions that increase or decrease local anesthetic action are those on the lipid-soluble group; and local anesthet-ics with an amide nitrogen in the intermediate chain of the anesthesiophore group tend to be more stable and to have a longer duration of action than those with an ester oxygen.
Neuronal factors that affect local anesthetic activity are axon diameter, myelinization, and rate of firing. The greater the diameter of the axon, the more internal membrane surface area and number of sodium channels there are to block and therefore, the lower the intensity and duration of anesthetic action.3 The greater the amount of myelin, the less readily the anesthetic penetrates the axon. Frequency dependency describes the observed phenomenon that the more rapidly a neuron discharges, the more rapidly the anesthetic enters the axon. Anesthetic agents whose molecules alternate between positively charged and neutral form at physiologic pH are most likely to show frequency dependency. Benzocaine, which is uncharged at physiologic pH, does not show frequency dependency.
The principal manufacturing factors that affect activity are drug concentration, pH of the solution, and the presence of a vasoconstrictor. Cocaine contains intrinsic vasoconstrictor properties. It blocks the reuptake of norepinephrine released by sympathetic nerve endings. All other local anesthetics block sympathetic neuron release of norepinephrine, resulting in vasodilation. The addition of a vasoconstrictor reduces drug absorption by the vasculature, thereby increasing the local effect of the anesthetic, reducing in theory its systemic toxicity, and reducing surgical bleeding. Norepinephrine is less stable than epinephrine, the vasoconstrictor that is usually added. Concentrations of epinephrine of approximately 1/200,000 (i.e., 0.0005%) are maximally effective.
There are many techniques for evaluating the intrinsic activity of a local anesthetic. One that was developed early on used the corneal surface of animals. In 1894 Von Frey reported using the tip of a single hair from a horse’s tail. Onset of anesthesia was defined as beginning with the loss of the blink reflex, and the duration of anesthesia ended with its return. Depth of anesthesia was determined by multiple rapid stimuli. For example, failure for a blink to occur during four stimulations at a rate of two per second might be considered complete anesthesia.
TERMINATION OF ACTIVITY
Most anesthetic molecules reaching the systemic circulation are metabolized to inactive forms before elimination from the body. Hydrolysis of the ester and amine groups and oxidation and dealkylation of amino groups can occur.4 For the amide anesthetic, lidocaine, dealkylation is the major, but not only, metabolic pathway.5 However, the role of local (e.g., orbital) tissue enzymes in terminating the anesthetic effect is limited.6 Passive diffusion of an anesthetic away from its site of action seems much more important in terminating action. Because motor axons tend to be of larger diameter than sensory axons, the former require a larger quantity of drug to maintain anesthesia. Therefore, as the tissue level decreases, motor activity recovers before sensory.7
ANESTHESIA OF THE EYE AND ORBIT
Topical, corneal, and injected orbital anesthesia are relatively safe ways to perform surgery. In 195 patients with known previous myocardial infarctions who underwent 288 ophthalmologic procedures under local anesthesia, there were no postoperative myocardial infarctions or deaths during, or in the week after, surgery. In 255 patients with previous angina pectoris but no documented myocardial infarctions who underwent 373 ophthalmologic procedures under local anesthesia, four patients had myocardial infarctions, two of whom died. In 9,617ophthalmologic procedures performed on patients with a history of neither myocardial infarction nor angina, there was one myocardial infarction.8
TOPICAL ANESTHESIA
The cornea and conjunctiva can be anesthetized by drops of any of the local anesthetics.
Bupivacaine, for example, is effective as an eyedrop.9 It is perplexing to see the surgeon stand over the patient with a syringe full of local anesthetic and call impatiently for a vial of topical anesthetic. Generally, those agents chosen to be topical anesthetics differ only in penetrating the sensory nerve endings more rapidly, thereby having a faster onset of action and a shorter period of producing ocular irritation.
The sympathetic β-receptor antagonist, propranolol, stabilizes neuronal membranes and produces anesthesia. For this reason, it is not used topically as an ocular hypotensive agent although it can effectively lower pressure. Propranolol is not a typical local anesthetic because it does not contain an anesthesiophore group; the aromatic hydrophobic group is not joined to the hydrophilic tertiary amine by an ester or amide group. The local anesthetic potency of propranolol is approximately equal to that of lidocaine. Several other β-blockers also have local anesthetic properties. For example, oxprenolol, acebutolol, and alprenolol have approximately half the anesthetic potency of propranolol. Timolol has no significant anesthetic properties.10
The depth and duration of topical anesthesia are dose-dependent.11,12 Return to full recovery after one drop of proparacaine 0.5% may take more than 1 hour and the maximal effect may last 5 to 20 minutes. Pathologic tissues (e.g., the hypesthetic corneas associated with previous herpes simplex or herpes zoster infections) may take longer to recover.13
In general, the ester topical agents, because of their relatively rapid tissue penetration, have a lower margin of safety and are often not marketed in an injectable form. Tetracaine is such an example. Procaine is an exception; it is an ester-containing local anesthetic available as an injectable agent. When used as a topical agent, procaine has the dual advantages of a rapid onset and a shorter duration of action.
Specific Uses
SKIN SURGERY.
Topical anesthesia of the eyelid skin, sufficient to perform surgery, has been achieved with creams and patches containing lidocaine, applied 60 to 90 minutes before the first incision.14
DIAGNOSTIC TESTS.
Topical anesthetics are routinely used when performing applanation tonometry. However, small but statistically significant reductions in intraocular pressure have been reported, lasting for 1 to at least 15 minutes, after their application. In healthy patients measured 1, 5, 10, and 15 minutes after one drop of 0.4% benoxinate, the intraocular pressure decreased from a baseline of 14.80 ± 3.28 mm Hg to 14.01 ± 3.33 mm Hg, 13.56 ± 3.12 mm Hg, 13.17 ± 1.12 mm Hg, and 13.98 ± 3.45 mm Hg, respectively.15 One drop of tetracaine 2% produced a small but significant reduction in intraocular pressure from baseline at 10 minutes (10.67 ± 2.55 mm Hg to 9.94 ± 2.17 mm Hg).16 The mechanism of this reduction may be an increased aqueous humor flow rate leaving the eye, but the evidence is not strong.17
Topical anesthesia has been used when trying to differentiate reflex from nonreflex tear production. The anesthetic drop is given before the test to prevent reflex tearing from corneal or conjunctival irritation during tear collection (e.g., by a paper strip [Schirmer test] or by a pipette). However, it was found that tearing remained nonlinear, with an initial rapid phase of tear secretion, long after the anesthetic drop was administered.18 Reflex tearing was not completely inhibited even by two drops of proparacaine 0.5% applied 5 minutes before the insertion of paper strips.
OCULAR SURGERY.
Topical anesthesia for adult cataract surgery has seen a rebirth. Regional retrobulbar injections of local anesthetics had replaced the topical cocaine introduced by Koller. The reintroduction of topical anesthesia began with its use in patients with coagulopathies in whom the threat of needle-induced retrobulbar hemorrhages existed.19 Topical anesthesia has subsequently had widespread use and is only slightly less effective than intracameral, sub-Tenon’s, peribulbar, or retrobulbar injections in preventing intraoperative pain.20,21,22,23,24,25,26 In general, the effectiveness of topical anesthesia appears uninfluenced by the use or absence of systemic analgesics preoperatively or intraoperatively.
POST-OPERATIVE ANALGESIA.
Post-operative pain after strabismus surgery performed on children, 1 to 12 years old, was found to be reduced for up to 8 hours by applying one drop of a topical anesthe-tic after intubation and a second drop immediatelybefore tubation.27 Those receiving placebo dropsrequired more analgesic drugs during the post-operative period. The pain after laser (photorefractive) keratectomy was reduced during the first 24 hours after surgery by using local anesthetic drops every 30 minutes; there was no impairment of corneal epithelial healing or visual acuity as measured 1, 3, and 6 months after the procedure.28
THERAPEUTIC USE.
Most ophthalmologists reject the thought of using long-term topical corneal anesthetic drops because of their potential side effects and toxicities. However, as treatment for trigeminal neuralgia, a small amount of literature indicates that topical anesthetics are efficacious and advocates their use.29,30
Topical lidocaine gel 5% has been applied to the face without occlusion, for 8 hours to relieve pain from herpes zoster infection.31 Significant pain relief begins by 30 minutes and lasts 24 hours. Blood levels are less than 0.3 μg/mL blood, well below the minimum of 0.6 μg/mL blood needed for any cardiac effect and the minimum of 2 μg/mL blood needed for a therapeutic antiarrhythmic effect. Long-term use for more than 2 months does not produce any systemic or topical ill effects other than some minor skin redness and irritation. A commercial preparation of lidocaine 5% is available as a patch.
Toxicity
Topical anesthetic agents can cause a decrease in corneal epithelial cell glycolysis, respiration, and healing. Microscopically, reduction in the numbers of corneal epithelial microvilli, disruption of the intercellular spaces, damage to the cell membranes and cytoplasm, and desquamation can be identified. Penetration of other topically applied agents, simultaneously or subsequently applied, is enhanced. The intraocular penetration of topical fluorescein is increased, aiding fluorophotometry. Mydriasis and cycloplegia are more rapid and profound when a topical anesthetic precedes the parasympatholytic eyedrop. However, at least one study has found evidence that the preservatives chlorhexidine and especially benzalkonium chloride, which are usedin commercial preparations of local anesthetics, are primarily the cause of this increased corneal epithelial permeability.32 It is, therefore, difficult to evaluate the role of the anesthetic agent itself when commercial preparations are reported to produce increased corneal thickness33 or epithelial permeability.34
Topical anesthetics retard healing of corneal abrasions by interfering with epithelial cell mitosis and migration.35 However, it is accepted practice for the physician to administer a topical anesthetic to assess the acutely traumatized eye. The pain, blepharospasm, photophobia, and epiphora after a corneal abrasion can prevent an accurate assessment of visual acuity and the structural integrity of the anterior segment. A drop or two of topical anesthetic will produce a dramatic, if only temporary, improvement in these symptoms.
Prolonged administration of topical anesthetics can produce a toxic keratopathy consisting of corneal epithelial defects, stromal edema and opacification, and anterior chamber inflammation. Corneal scarring and endothelial damage may require a penetrating keratopathy for visual rehabilitation.36 A sterile corneal ring infiltrate may mimic an infectious process, such as Acanthamoeba keratitis.37 The relative proportions of the keratopathy attributable to the anesthetic agent and the preservative are not clear. Healthcare workers, especially physicians,38 and the psychiatrically disturbed are the two groups most likely to abuse corneal anesthetics. If the cause of the keratopathy is not recognized, corticosteroid and antibiotic eyedrops may be given, increasing the chances of secondary fungal superinfections and resistant bacterial infections (e.g., Candida species, staphylococci, streptococci, and Proteus species).39
Topical anesthetics are routinely used when obtaining scrapings from a presumably infected cornea. A portion of the scrapings can be used to culture the causative microorganism. However, the anesthetic preparation may be toxic to the organism and impair its growth. It would seem unlikely that, in the clinical situation, a drop or two of an anesthetic agent applied to the cornea would have any significant antibacterial effect. However, when a 50-μL drop of a commercial topical anesthetic preparation was placed on an infected mouse cornea, there was a reduction in the number of microorganisms recovered; this reduction correlated with the presence, type, and concentration of preservative.40 The preservative chlorobutanol 0.2% or 0.4% was much less effective than benzalkonium 0.01% or chlorhexidine 0.001% in inhibiting growth; benzalkonium 0.004% had little inhibitory effect, as did unpreserved anesthetic agents. The earliest in vitro studies showing microorganism growth inhibition added the local anesthetic directly to the incubation medium, exposing the organisms to it continuously. Under these conditions, the anesthetic agent can retard microbiological growth even if the preservative is not present.41 The local anesthetics tetracaine, benoxinate, and cocaine and the preservatives chlorobutanol and butyl parahydroxybenzoate could each alone inhibit growth. This effect depended on the drug concentration and the organism. Unpreserved proparacaine in concentrations of up to 0.5% did not show significant growth inhibition of the strains of staphylococci, Pseudomonas and Candida that were tested.
Whereas suppression of organism growth is detrimental if the goal is to obtain a culture, this same suppression is of value if the goal is to prevent contamination of a reusable anesthetic preparation. Three commercial fluorescein 0.25%-anesthetic combinations were evaluated for their ability to regain sterility after contamination with Pseudomonas or staphylococci.42 The preparation containing the anesthetic agent benoxinate hydrochloride 0.4% with chlorobutanol 1% as preservative was more effective in regaining sterility than were the two different commercial preparations containing proparacaine hydrochloride 0.5% with thiomerosol 0.01%.
INJECTION ANESTHESIA
Subcutaneous injections of local anesthetics numb the skin of the lids, brows, and temporal areas served by the distal branches of the trigeminal nerve. Facial nerve motor fibers to the muscles in these structures run more deeply than the sensory fibers and are more variably affected by subcutaneous injections. In 1914 Auguste Van Lint43 described a technique for deliberately blocking motor conduction to the lids by injecting the anesthetic solution deeply, along the bones of the outer margins of the lateral and inferior orbital rim. By paralyzing the orbicularis muscle, lid closure during cataract surgery was prevented.
Intraorbital anesthetic injections include subconjunctival,44 sub-Tenon’s,45 epibulbar or peribulbar,46 and retrobulbar techniques. Not only is the ophthalmic branch of the trigeminal nerve anesthetized by injections into the posterior orbit, but so is the maxillary branch, which is exposed after it enters the orbit through the inferior orbital fissure. Because the needle tip cannot be visualized and the limits of anesthetic diffusion cannot be determined, there may be considerable overlap in the structures affected by these seemingly different techniques. Tracking of anesthetic solution along the needle pathway may also alter the tissues affected. When local anesthetic containing contrast material was injected behind the eye, either inside or outside the muscle cone, computerized tomography showed that the dye had diffused to both sides of the muscle cone within 3 minutes.47
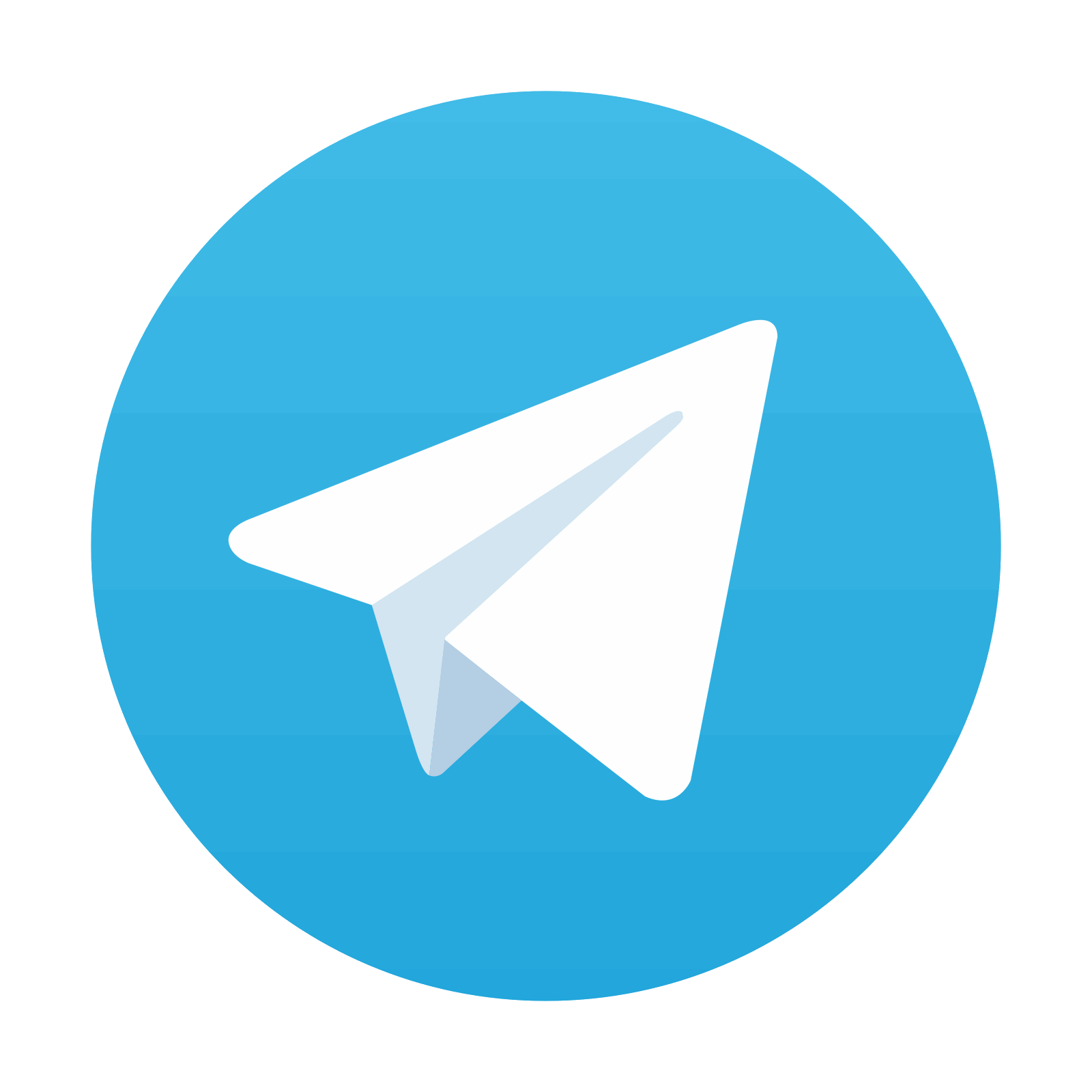
Stay updated, free articles. Join our Telegram channel

Full access? Get Clinical Tree
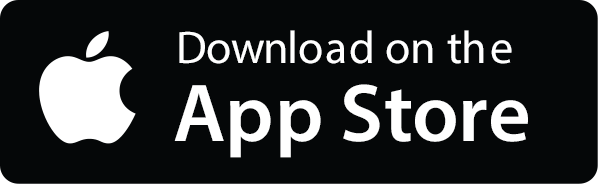
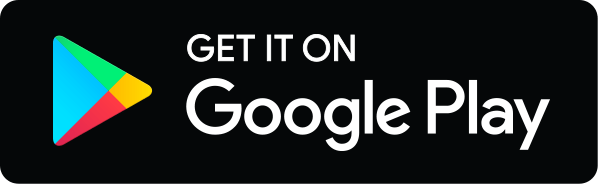