Pharmacokinetics
Joel S. Mindel
DEFINITION
Pharmacokinetics refers to how the patient’s tissues affect the therapeutic response. Factors such as drug penetration, drug metabolism, and drug excretion are considered.
TOPICAL OCULAR MEDICATIONS
EYELIDS
The eyelids can be a very effective barrier, as can be seen in uncooperative children who squeeze their lids tightly. One technique for delivering topical medications to these eyes is to place the drops on the medial canthus with the patient lying supine.1 Eventually, the child will open the lids and the drops will run in. One study compared this technique with instilling eyedrops with the lids open, in the usual manner.2 Eighty adult volunteers received two 30-μl drops of cyclopentolate 1% to one eye, once with the eyes open and once with the eyes closed. There was no significant difference between the two methods for the following parameters: time to reach peak mydriasis, peak cycloplegia, and peak cyclopentolate blood level and amount of peak mydriasis, peak cycloplegia and peak cyclopentolate blood level.
LACRIMAL VOLUME AND TURNOVER
The lacrimal volume is 6 to 7 μl.3,4 If the lids are permitted to blink, only an additional 3 μl of fluid can be added without overflow, giving a maximum of 10 μl.5 If the lids are prevented from blinking and the head is held upright, a maximum of 30 μl can be retained. Assume that a 50-μl eye drop is placed on the blinking eye (depending largely on the outside diameter of the eye-dropper tip, commercial eye drops can vary greatly in volume; e.g., glaucoma medications can range from 24 to 56 μl.6,7,8) The best retention possible, if the tear fluid were entirely replaced by the medication, would be 20% (i.e., 10 μl/50 μl). Eighty percent of the drug given has been lost onto the patient’s lid because of overflow. Compounding this poor retention is a tear turnover rate of approximately 16% per minute. Five minutes after the drop has been instilled, only 40% of the medication initially retained by the lids is still present.9 In the example given, this means that, at best, only 8% of the medication in the original 50 μl drop is present in the tear film 5 minutes after it is applied. The additional problem of a variable but usually significant reflex tearing after eye drop administration leads one to conclude that very little of the medication applied has the opportunity to be absorbed. This situation compares very unfavorably to that found with oral medications in which the drug is in contact with the intestine for hours to days. Reflex tearing can be minimized to some extent. Prior administration of a local anesthetic drop will reduce tear turnover, as well as increase drug absorption, by altering the corneal epithelium.10
Zimmerman and coworkers10a have provided evidence that continuous eyelid closure and/or occlusion of the nasolacrimal duct by digital compression of the nasal canthal area produces increased drug absorption. Both techniques reduce the drainage of tear fluid and prolong eye-drug contact. Digital compression obstructs tear flow. Continuous lid closure prevents blink-induced nasolacrimal pump drainage. Total punctal occlusion, with a plug, improves the efficacy of glaucoma medications.11
White and coworkers12 reported a study in which technetium pertechnetate levels in the tear film were measured twice in the same eye—once with the eye allowed to blink normally for 5 minutes and once with the eye closed for 5 minutes. The clearance rate was significantly faster in the blinking eye for 0 to 2 minutes after adding the technetium, but there was no significant difference after 2 minutes. The mean ± standard error technetium half-life for 0 to 2 minutes was 93 ± 10 seconds in the blinking eyes and 1290 ± 578 seconds in the closed eyes; for 2 to 5 minutes, it was 1080 ± 223 seconds for blinking eyes and 1040 ± 151 seconds for closed eyes.12
In another study, when nasolacrimal occlusion was performed for 3 minutes, a single drop of pilocarpine 2% gave as effective a hypotensive response for 12 hours as a single drop of 4% pilocarpine.13 The same was true for carbachol 1.5% with nasolacrimal occlusion versus carbachol 3%. Timolol maleate 0.25%, after nasolacrimal occlusion, was as effective for 24 hours as timolol maleate 0.5%. When aqueous humor samples were obtained without nasolacrimal occlusion or after 5 minutes of nasolacrimal occlusion in patients given a single dose of timolol maleate 0.5%, peak timolol levels occurred in both groups 1 hour after instillation. However, the mean level of aqueous humor timolol was significantly higher, by approximately 70%, for up to 180 minutes in those eyes with occlusion.14 Nasolacrimal occlusion achieved no additional hypotensive benefit from pilocarpine 4%, epinephrine 1%, or dipivefrin 0.1%, which indicated that these doses were already at their peak therapeutic concentrations in amount of intraocular pressure lowering and duration of effect.15
The rate of drainage through the canaliculi may be related to the volume of drug solution instilled.16 The larger the volume, the more rapid the drainage. Therefore, it would seem that smaller eye drops containing drugs in higher concentration would be more effective. However, the situation is more complex.17 In humans, drop sizes ranging from 1 to 10 μl18 and from 5 to 15 μl19 did not alter the drainage rate. When drops approached 20 μl in volume or became larger, their size may have altered lacrimal drainage, but overflow occurred, complicating the analysis. Thus, a report by Brown and coworkers20 that two 8-μl drops of 10% phenylephrine were more effective as a mydriatic than two 32-μl drops of 2.5% phenylephrine (6.7 ± 0.3 mm vs. 5.7 ± 3 mm) is difficult to interpret.
There are a number of arguments that can be made for and against the use of drug combinations. The rabbit studies of Chrai and coworkers21 support their use. These investigators found that if different drugs were given separately 1 minute apart, the second drug washed out a disproportionately greater amount of the first drug. The loss of each drug was proportional to the volumes instilled. If 0.01 M pilocarpine nitrate were given before 0.01 M epinephrine hydrochloride, the aqueous humor concentration of the former was 0.388 μg/ml and that of the latter, 0.024 μg/ml. If the order of administration was reversed, then the concentration of aqueous humor epinephrine fell to 0.016 μg/ml, whereas the pilocarpine concentration rose to 0.573 μg/ml. With regard to applying a second drop of the same medication, rabbit studies found very little benefit.21 If the drops were given less than a minute apart, the gain in tear film concentration of the drug was only 10%. If the drops were given 3 minutes apart, the increase in tear film concentration was only 20%.
The beneficial effect of a second drop in humans might be greater because the rabbit tear turnover rate of 7.1% is less than half that of humans (16%).22 In addition, the nictitating membranes of rabbits may provide prolonged drug contact time. Miller and O’Connor23 removed the nictitating membranes from a number of rabbit eyes and found that tear film fluorescein was present nearly three times as long, 8.0 ± 0.7 minutes versus 3.2 ± 0.3 minutes, in rabbits with nictitating membranes. In another study, drops of tropicamide, 0.125%, or phenylephrine, 2.5%, were instilled in two different groups of 50 volunteers.24 One eye received two drops of the same drug, one drop immediately after the other; the other eye received only one drop of that drug. Pupil measurements were made for 60 minutes after tropicamide and for 90 minutes after phenylephrine. For tropicamide the mean dilation 20 minutes after instillation was 2.2 ± 0.75 mm in eyes receiving two drops and 1.4 ± 0.72 mm in eyes receiving one drop. At maximum dilation, 60 minutes, the corresponding dilations for two drops and one drop were 3.16 ± 0.83 mm and 2.34 ± 0.75 mm. For phenylephrine, the mean dilation 30 minutes after instillation was 2.49 ± 0.75 mm in eyes receiving two drops and 1.56 ± 0.68 mm in eyes receiving one drop. At maximum dilation, 90 minutes after instillation, the corresponding increases in pupil diameter for two drops and one drop were 4.19 ± 0.83 mm and 3.21 ± 0.71 mm. These differences in dilation, greater by approximately one third in eyes receiving two drops, were highly significant.
The lacrimal fluid contains protein, which can bind drugs and diminish their therapeutic efficacy. The tears in humans have an estimated 0.7% protein content, with 0.4% albumin.9 Mikkelson and coworkers found that addition of 3% bovine serum albumin to rabbit tears lowered the miotic response to 50 μl of 0.025 M pilocarpine nitrate to a level equivalent to that of 0.00025 M pilocarpine (i.e., one hundredth as concentrated).25 They conjectured that in certain disease states, such as conjunctivitis, increased lacrimal protein might alter the therapeutic response. They have also provided proof that excipients in pharmaceutical preparations can compete for the same lacrimal and corneal protein binding sites as pilocarpine.26 Cetylpyridinium chloride 0.02% enhanced the therapeutic efficacy of pilocarpine solutions tenfold. This may, in part, have been due to a surfactant effect that promoted penetration through a partially disrupted corneal epithelium barrier. However, addition of cetylpyridinium chloride to fluorometholone solutions did not increase corneal penetration. The neutral fluorometholone molecule does not compete for the same protein binding sites as the cationic cetylpyridinium chloride. Green and Downs27 found that corneal penetration of the anion prednisolone phosphate was not improved by the presence of 0.01% benzalkonium chloride, a cationic surfactant. The explanation may be that they compete for different protein binding sites. Perrin and Nelson28 have shown that benzalkonium chloride binds strongly to albumin.
Tear pH and buffering capacity are important to corneal penetration of drugs. The manufacturers almost always make their preparations at a pH level below 7 (e.g., Propine is pH 2.5 to 3.5). This increases the shelf life of drugs, most of which have pK values above 7. The manufacturer depends on the patients’ tear pH and buffering capacity to rapidly overcome the unbuffered pH of the eye drops. Carney and coworkers29 have shown that the mean ± standard deviation (SD) pH of tears is 7.50 ± 0.16 and that the buffering capacity is most effective against an acidic challenge. The buffering system is believed to have bicarbonate and proteins as its two major components.
CORNEA
The cornea presents a physical barrier to intraocular drug penetration. Active transport has not been demonstrated. Rather, drugs appear to penetrate by diffusion. This process is characterized by the following factors: the rate of diffusion parallels drug concentration, the process is not saturable, the process is not retarded by metabolic inhibitors, and penetration will not occur against an electrochemical gradient.
Because of the limited time that drugs in the lacrimal fluid are in contact with the cornea, the first barrier to penetration, the corneal epithelium is the most important. Drugs must enter the corneal epithelium rapidly or they will be washed away.
The corneal epithelium is about five cell layers thick. Cell membrane lipids limit drug penetration. The greater the lipid solubility of a drug, the greater its diffusion into the corneal epithelium.30 Lipid solubility is expressed as the partition coefficient. It is determined by using a phase separation of an oil (e.g., olive oil) floating on water. The drug is added, and, after allowing enough time for an equilibrium to be achieved, the amounts in the oil phase and in the water phase are measured. The partition coefficient is the ratio of the amount of drug in the oil phase as numerator and the amount of drug in the water phase as denominator. The higher the partition coefficient, the greater is the lipid solubility. For example, the β-adrenergic antagonists propranolol, levobunolol, and timolol lower the intraocular pressure when applied topically and have high partition coefficients, respectively, of 1640, 249, and 82. Nadolol and sotalol are β-adrenergic antagonists that are relatively ineffective when given topically; their partition coefficients are low, 8.4 and 0.24, respectively. These latter drugs penetrate the cornea less readily.31
Leibowitz and Kupferman and their coworkers32,33,34,35,36,37,38 have written extensively about the effect of lipid solubility on glucocorticoid penetration. The lipid solubilities of the different esters of dexamethasone and prednisolone increase in the following sequence: phosphate << free alcohol that is < acetate. They found that rabbit corneal epithelial levels of corticosteroid esters, administered both as drops and ointments, followed their lipid solubilities (Table 1). Intraocular inflammation produced by intravitreal injections of clove oil enhanced epithelial penetration of the less lipid-soluble esters (i.e., the phosphate and free alcohol). However, there was little or no increase in penetration of the more highly lipid-soluble acetate (Table 2). Presumably, the inflammation disrupted the epithelial barrier and aided penetration of the less lipid-soluble esters. The increase in corneal penetration was insufficient to overcome the advantage that increased lipid solubility gave the acetate ester. As a result, in both the normal and inflamed eye, the acetate ester gave the highest corneal concentrations. These data cannot be directly translated into statements about the relative therapeutic efficacy of these corticosteroids because their intrinsic potencies may differ.
TABLE 1. Corticosteroid in Rabbit Corneas Following Topical Administration of 0.05 ml | ||||||||||||||||||||||
---|---|---|---|---|---|---|---|---|---|---|---|---|---|---|---|---|---|---|---|---|---|---|
|
(Kupferman A, Liebowitz HM: Topically applied steroids in corneal disease: IV. The role of drug concentration in stromal absorption of prednisolone acetate; V. Dexamethasone alcohol; VI. Kinetics of prednisolone phosphate. Arch Ophthalmol 91:377, 92:329, 331, 1974)
TABLE 2. Effect of Inflammation on Corticosteroid Penetration in Rabbit Corneas Following Topical Administration of 0.05 ml | ||||||||||||||||||||||
---|---|---|---|---|---|---|---|---|---|---|---|---|---|---|---|---|---|---|---|---|---|---|
|
*Compared to uninflamed corneas in Table 1.
(Kupferman A, Liebowitz HM: Topically applied steroids in corneal disease: IV. The role of drug concentration in stromal absorption of prednisolone acetate; V. Dexamethasone alcohol; VI. Kinetics of prednisolone phosphate. Arch Ophthalmol 91:377, 92:329, 331, 1974)
The corneal epithelium contains hydrophilic constituents that retard drug penetration. Cell membrane phospholipids and proteins have anionic and cationic binding sites. Smolen and coworkers39 have made the following observation: The miotic response to anterior chamber injections of carbachol was increased by placing 0.1 ml of 0.06% diethylaminoethyl dextran on rabbit corneas. The molecular weight of the dextran was approximately 2 million, which made it unlikely that it penetrated the corneal epithelium. They attributed the identical effect of 0.03% benzalkonium chloride to a corneal surface interaction: Binding of these two cationic molecules with surface anionic sites might produce a significant displacement of electrons. This would alter protein-carbachol binding in the deeper layers of the cornea. Smolen and coworkers40 showed that the fixed charge density of the corneal surface could be altered by the pH of an applied solution. This occurred in a manner similar to the pH titration curves of amphoteric macromolecules. The epithelial surface was predominantly acidic at physiologic pH 7.4. The exposed negatively charged sites could interact with positively charged molecules such as the quaternary amine carbachol.
Ramselaar and coworkers41 studied the effect of local anesthetics and preservatives on the penetration of topical fluorescein into the human cornea. Five drops of each topical anesthetic, oxybuprocaine or tetracaine, did not significantly increase penetration. The preservatives, chlorhexidine and benzalkonium, and combinations of preservatives and local anesthetics did increase penetration. However, their effectiveness seemed due to punctate breakdown of the corneal epithelium. For example, three drops of a tetracaine-benzalkonium combination failed to increase fluorescein permeability. Four drops resulted in 40% of treated eyes having punctate staining.
Largely of historic interest, iontophoresis was used to aid corneal penetration of ionizable drugs having very limited lipid solubility. One electrode was placed on the cornea in the presence of a drug solution. The other electrode could be placed anywhere else on the body. If the corneal electrode were made the anode, positively charged drugs were driven into the eye by the current. If the corneal electrode were made the cathode, negatively charged drugs were driven into the eye. The epithelial damage produced by the electrode (usually placed in a scleral contact lens), the current, the topical anesthetic, the hypertonic solution, and the nonphysiologic pH aided drug penetration. It is questionable whether the current alone was ever responsible for increased corneal epithelial penetration. Boyd,42 for example, applied hypertonic 5% sodium sulfathiazole, pH 10.5, to rabbit corneas using an iontophoresis contact lens. He observed that currents of more than 3 mA when applied for more than 5 minutes produced gross epithelial damage. Once the corneal epithelium was breached, the current would drive molecules into the stroma. Boyd found the corneal concentration of sulfathiazole three times greater if a 1-mA current were used compared with using no current. The development of topical drugs with increased lipid solubilities made iontophoresis unnecessary. Nonionizing antibiotics, such as tetracycline, chloramphenicol, and bacitracin, would not benefit from iontophoresis.43
The corneal stroma presents a barrier unlike that presented by the epithelium. The stroma is relatively acellular and made up primarily of collagen. Although it makes up approximately 90% of the cornea by weight, it contains only 1% of the lipid. As a result, drugs need not have lipid solubility to traverse the corneal stroma. Water solubility is required instead. Leibowitz and Kupferman studied the stromal absorption of different glucocorticosteroid esters after removal of the corneal epithelium.34,38 As expected, the water-soluble phosphate ester penetrated the stroma most readily. The acetate ester and free alcohol produced lower stromal drug levels (Table 3).
TABLE 3. Total Corticosteroidin Denuded Rabbit Corneas Following Topical Administration of 0.05 ml | ||||||||||||||||||||||
---|---|---|---|---|---|---|---|---|---|---|---|---|---|---|---|---|---|---|---|---|---|---|
|
(Leibowitz HM, Kupferman A: Bioavailability and therapeutic effectiveness of topically administered corticosteroids. Trans AM Acad Ophthalmol Otolaryngol 79:78, 1975)
The third component of the cornea, the endothelium, is one cell layer thick. Presumably, it acts as a lipid barrier, although its thinness makes it much less formidable than the corneal epithelium.
The need for drugs to have both lipid and water solubility if they are to penetrate the cornea is called the differential solubility concept. It has been used to explain the diffusion characteristics of many drugs. A logical application can be made for weak bases such as pilocarpine, tropicamide, epinephrine, cyclopentolate, and atropine.44 These exist in both ionized and nonionized molecular forms at physiologic pH 7.4. The nonionized form, being the more lipid soluble, penetrates the corneal epithelium and endothelium. The ionized form, being the more water soluble, penetrates the corneal stroma (Fig. 1). However, this diagram does not seem valid for all drugs. For example, the quaternary amines, such as carbachol, echothiophate, and demecarium, carry positive charges at all pH levels. Despite the low lipid solubilities of these ions, they penetrate the cornea in sufficient amounts to be therapeutically useful (Fig. 2). Other exceptions are those molecules with significant water solubility in the nonionized form and significant lipid solubility in the ionized form (Fig. 3). Pilocarpine is an important example. Ionized pilocarpine can penetrate all these corneal layers, as can nonionized pilocarpine. Increasing evidence suggests that although transcorneal penetration of the nonionized form of a drug is determined by its lipid solubility, the penetration of the ionized form of a drug into the anterior chamber is much greater than lipid solubility would allow. For example, Maren and Jankowska45,46 found that the highly ionized molecule bromacetazolamide had 20 times the permeability at pH 7.6 than could be explained by penetration of the nonionized form of the molecule. However, penetration of the drug into the posterior chamber more closely reflected the lipid solubility of the drug. The authors interpreted this latter finding as evidence that entry into the posterior chamber is achieved by limbal penetration of the drug followed by direct diffusion through iris and ciliary body. Ahmed and Patton47 also found evidence that topically applied drugs could enter the eye yet bypass the anterior chamber. In their studies, the drug was believed to enter the conjunctiva, diffuse across the sclera, and then penetrate the uveal tissues.
![]() Fig. 3. The transcorneal diffusion of a molecule with significant water solubility in the un-ionized form and significant lipid solubility in the ionized form (e.g., pilocarpine). |
In the past, the cornea has been looked on only as a barrier to drug penetration. More recently, the work of Makoid, Sieg, and Robinson48,49,50 has emphasized a different aspect: the cornea as a drug reservoir. Krohn and Breitfeller51 developed an in vitro rabbit corneal chamber with an epithelial irrigating system mimicking tear flow. When an 8% pilocarpine solution was allowed to remain in contact with the corneal epithelium for only 30 seconds, pilocarpine molecules could be detected diffusing through the cornea 90 minutes later. Van Hoose and Leaders52 hypothesized that the endothelium retarded pilocarpine diffusion into the aqueous humor, creating a stromal drug reservoir. However, Sieg and Robinson50 provided data supporting an alternative view. After applying 10-2 M pilocarpine to intact corneas, they scraped off the epithelium at various time intervals. The amounts of pilocarpine in the stroma and endothelia were assayed. These values were compared with those from corneas with intact epithelia (Table 4). The data indicated it was the epithelium that acted as the reservoir. The levels in stroma and endothelium (in micrograms per gram tissue) and in aqueous humor (in micrograms per milliliter) were nearly identical during the 2-hour period studied (i.e., they were in equilibrium and could be considered a single drug compartment). Mindel and coworkers53 found that although drugs were distributed in the cornea according to their partition coefficients, with a more lipid-soluble drug found in higher concentration (calculated as milligram of drug per milligram of tissue) in the corneal epithelium and a more hydrophilic drug found in higher concentration in the stroma, the ninefold greater mass of the stroma tended to make it the more important reservoir (calculated as milligram of drug per whole tissue).
TABLE 4. Corneal Concentration Following Topical 10-2 M Pilocarpine | |||||||||||||||||||||
---|---|---|---|---|---|---|---|---|---|---|---|---|---|---|---|---|---|---|---|---|---|
|
(Sieg JW, Robinson JR: Mechanistic studies on transcorneal permeation of pilocarpine. J Pharm Sci 65:1816, 1976)
The existence of a corneal reservoir might explain an observation by Harris and Galin,54 who found that if a single pilocarpine drop were given to patients with open-angle glaucoma, it made little difference whether a 1% or 10% solution were used. For a 2-hour postinstillation period, the decreases in intraocular pressure were indistinguishable. However, chronic administration of 4% pilocarpine drops produced a reduction in intraocular pressure that was far superior to that from chronic administration of 1% pilocarpine. This superiority could result from a greater accumulation of pilocarpine in the corneal epithelium using the 4% concentration.
Metabolizing enzymes in the cornea, especially in the corneal epithelium, can enhance or limit a drug’s effect. Dipivefrin is a prodrug of epinephrine. The conversion of dipivefrin to epinephrine is, at least in part, dependent on the activity of tissue esterases, which remove the two pivalic moieties by hydrolysis.55 These esterases do not appear to be cholinesterases because they are not inhibited by diisopropylfluorphosphate.56 Acetylating enzymes in the cornea, and especially in the iris-ciliary body, seem to explain why the topical carbonic anhydrase inhibitor aminozolamide is effective as an ocular hypotensive agent. Retention of high levels of the active metabolite, 6-acetamido-2-benzothiazolesulfonamide, has been found in these tissues.57 Degradation of pilocarpine to inactive pilocarpic acid has been demonstrated in the rabbit eye58; the corneal stroma-endothelium, as well as the corneal epithelium and iris-ciliary body, were sites of metabolism. The presence of atropinase and cocainesterase in rabbit ocular tissues and plasma has also been documented.59
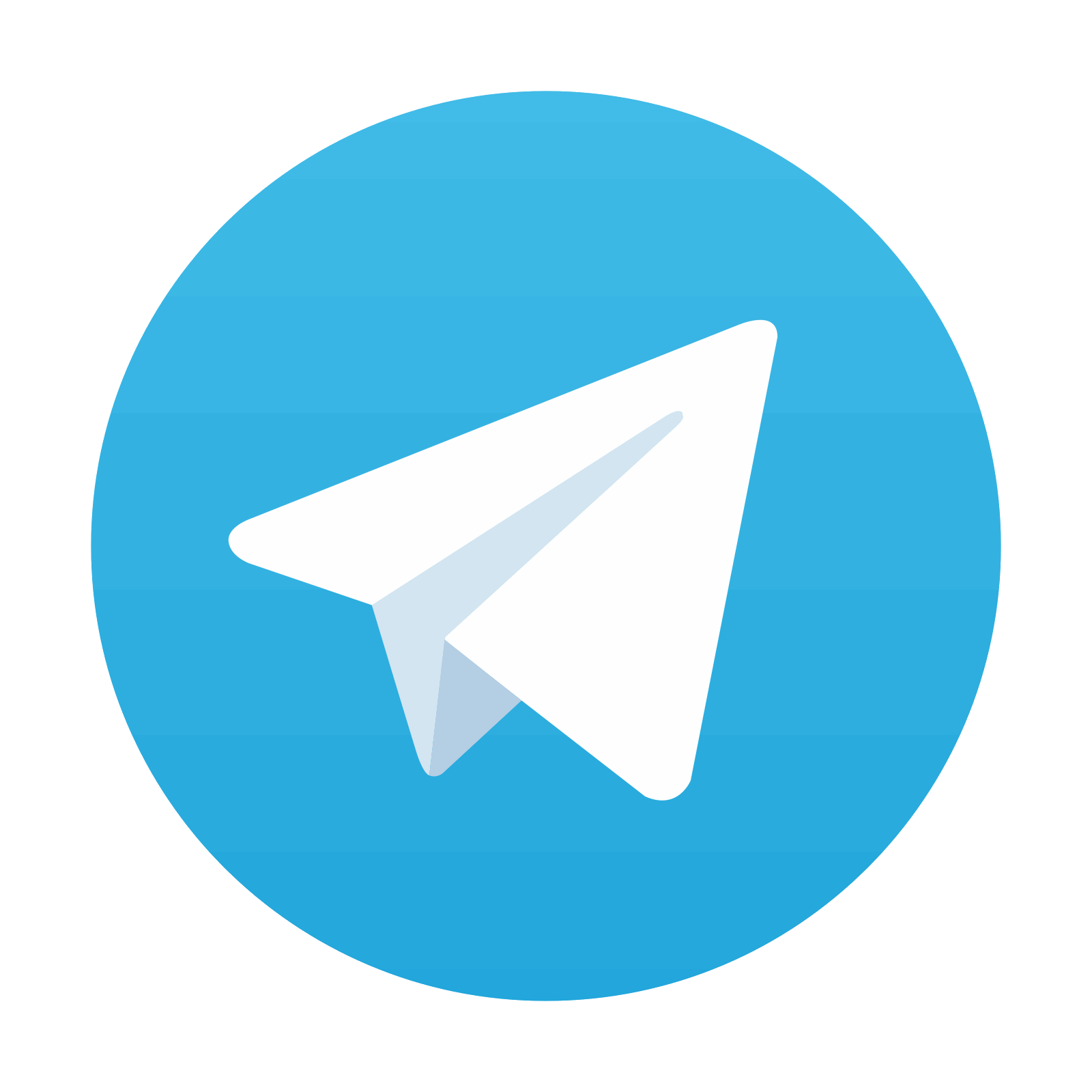
Stay updated, free articles. Join our Telegram channel

Full access? Get Clinical Tree
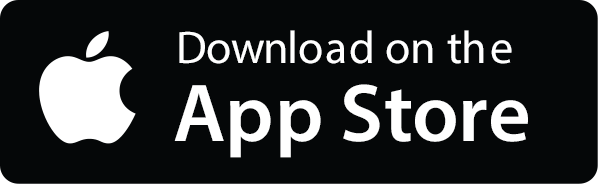
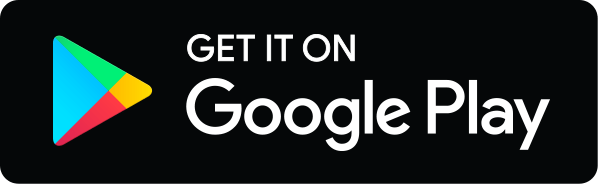