Fig. 8.1
Diagram shows normal ocular and vitreous landmarks. Cloquet’s canal is located centrally, with the annular attachment of vitreous to the lens represented by the hyalocapsular ligament (HC). The vitreous base (VB) straddles the junction of ora serrata and pars plana; the ciliary body (CB) is just external and anterior to the face of anterior hyaloid (arrow). A firm attachment of vitreous surrounds the optic nerve head (wavy arrow)
The vitreous is 98 % water and contains a network of long, unbranched collagen fibrils (90 % type II collagen) that share chemical similarities to the fibrils of articular cartilage [1]. Unlike opaque articular cartilage with its densely packed collagen, the fibrils of vitreous are widely separated. The optical transparency of vitreous is attributed to three factors: the thinness of the fibrils (8–16 nm), their wide separation, and random orientation. Given the near absence of cellular elements in the normal vitreous, light scatter is minimal, although some structural organization is perceptible with good quality slit lamp biomicroscopy.
A third critical constituent of vitreous is hyaluronic acid. As the major glycosaminoglycan of the vitreous, hyaluronic acid is a macromolecule whose flexible coiled configuration and anionic charges are ideally designed to interact with other molecules [2]. The most important interaction hyaluronic acid has is with collagen fibrils. It is through this interaction that collagen traps water and maintains proper spacing between neighboring fibrils.
Soluble proteins, low molecular weight organic and inorganic salts, and glycoproteins are also found in the vitreous [3]. Their concentrations usually mirror those in serum. The vitreous contains high levels of ascorbic acid and lactic acid. The concentration of the latter metabolite is highest in vitreous cortex presumably due to aerobic metabolism of glucose in the retina. After death, the osmolality of human vitreous is slightly greater than that of serum [4].
Notwithstanding its near optical transparency, the vitreous has a deceptively elusive architecture, best seen with magnification and special methods of illumination. Investigators attempting to characterize the gross assembly of the vitreous body have yet to arrive at any consensus [5]. Most descriptions appreciate concentric funnels each delineated as a diaphanous chamber whose apex converges on the optic disc. The most central of these funnels is Cloquet’s canal, the vestigial remnants of the hyaloid vascular system. The vitreous cortex refers to the outer shell of vitreous approximately 100 μm in thickness. It corresponds anatomically to more densely packed collagen fibrils [6]. Immediately internal to the optic disc and macula, the vitreous lacks the same density of collagen fibrils found in other regions of the posterior cortex. The surface of the anterior hyaloid separates the vitreous from the posterior chamber.
The normal vitreous cortex contains cells known as hyalocytes, which by light and electron microscopy resemble macrophages. The density of hyalocytes in the cortex is low. Although they can function as phagocytic cells when appropriately stimulated, their role in normal vitreous development and homeostasis remains unclear.
The internal limiting membrane of the retina interfaces the vitreous. It is a composite structure made up of the basement membrane of Mueller cells and an inner portion of vitreous fibrils [5]. The vitreous contributions to the internal limiting membrane are anchoring fibrils, whose strength of attachment likely varies due to their density and lengths of insertion. The strongest attachments are found at the vitreous base (an approximately 3 mm zone that straddles the ora serrata), along major retinal vessels, and at the margins of the optic disc. Notable attachments are also present in the fovea and parafoveal areas. The vitreous also adheres to the posterior surface of the lens. This so-called hyaloideocapsular ligament represents an annular adhesion in the outer third of the lens capsule.
8.3 Embryology
Not long after conception, the crescent-shaped cavity between the posterior surface of the lens vesicle and inner wall of the optic cup fills with a primordial jelly known as the primary vitreous. This primitive mesenchyme is identifiable after the 3rd week of gestation; by 8 weeks, the development of primary vitreous is nearly complete. Other than minor modifications, it will remain sandwiched between lens and developing retina until completely replaced with tissue resembling adult vitreous in the last trimester.
Between the 5th and 6th weeks, the primary vitreous is invaded by vasoformative cells originating near the lens (capsula perilenticularis) and at the optic nerve head. These sprouting vessels grow towards each other to form the hyaloid vascular system, a transient vascular supply that begins to regress during the 7th month and fully involutes before birth.
The primary vitreous is replaced by the secondary vitreous, a process that starts at the inner surface of the retina and then progresses centrally (Fig. 8.2). As the secondary vitreous increases in mass, it compresses the vascularized primary vitreous, eventually leaving a thin layer of connective tissue surrounding the major hyaloid vessels. After the largest of these vessels regress, diaphanous sheets of connective tissue (known as Cloquet’s canal) are all that remain of the embryonic hyaloid vascular system. The cell responsible for the coordinated synthesis of secondary vitreous is subject to debate.
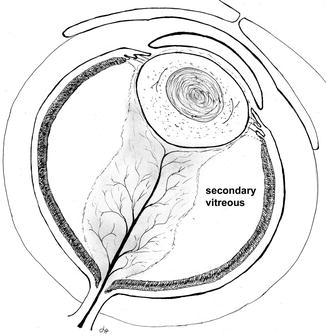
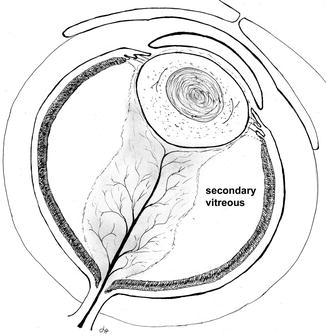
Fig. 8.2
The illustration shows the relation of the vascularized primary vitreous when it is surrounded by secondary vitreous at roughly the second month of gestation
According to the terminology just cited (originated by Ida Mann), the concept of vitreous development is biphasic. The tertiary vitreous, which refers to the suspensory ligament of the lens, is not the subject of this chapter.
8.4 Congenital Abnormalities
8.4.1 Persistent Hyperplastic Primary Vitreous
Failure of the primary vitreous to completely regress is the most common developmental anomaly of the vitreous, although in absolute terms it is a relatively rare condition. Historically, this congenital anomaly has been called persistent hyperplastic primary vitreous (PHPV), but some argue the term persistent fetal vasculature (PFV) is more appropriately descriptive [7]. Traditionally, PHPV was divided into anterior and posterior forms. Ninety percent of cases are unilateral, and most occur as sporadic anomalies. The genetic locus of a familial non-syndromic form of PHPV has been found on chromosome 10q11-q21 [8]. This inherited variety is transmitted as an autosomal recessive trait. Anterior PHPV presents clinically with leukocoria, as the white persistent primary vitreous is observed through a clear lens. Typically, the eye is small and has elongated ciliary processes visible around the lens when the pupil is dilated.
Histologically, anterior PHPV is characterized by a vascularized mass of loose connective tissue behind the lens (Fig. 8.3a, b). The persistent primary vitreous appears to drag ciliary processes inward. The contractile nature of this tissue is inferred by the wrinkling it causes in the posterior lens capsule (Fig. 8.3b). The posterior lens capsule may eventually tear under this stress, resulting in a granulomatous inflammatory reaction to exposed lens protein, a condition referred to as phacoantigenic uveitis (also incorrectly called phacoanaphylactic uveitis in the literature).
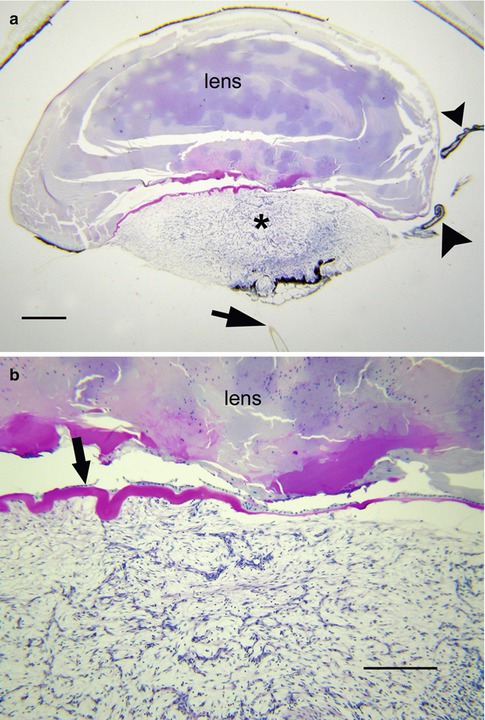
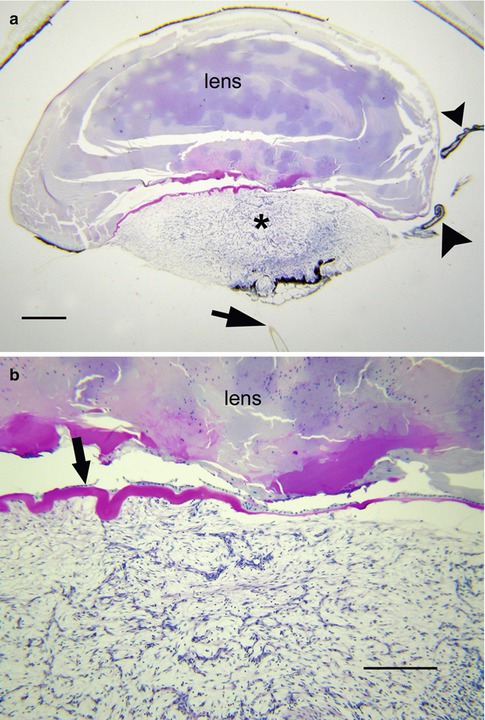
Fig. 8.3
(a) Persistent hyperplastic primary vitreous (PHPV) seen with residual hyaloid artery (arrow) and elongated ciliary processes (arrowheads) (bar = 800 μ). (b) Higher magnification of a shows undulating posterior lens capsule (arrow) of widely varying thickness. The persistent primary vitreous is composed of bland, widely separated spindle cells (bar = 160 μ)
Posterior PHPV can be seen as an isolated malformation or associated with anterior PHPV. Failure of the hyaloid vascular system to regress behind the equator of the eye defines posterior PHPV. The posterior variant of PHPV can range from a small tuft of fibroglial tissue on the optic nerve (Bergmeister’s papillae) to a patent hyaloid artery coursing to the lens. These stalks of vascular tissue can be associated with retinal folds. Small amounts of persistent primary vitreous can be associated with other developmental anomalies of the eye and should not be confused with classic sporadic PHPV [9].
8.4.2 Congenital Vitreous Cyst
Congenital vitreous cyst is a rare malformation usually detected in childhood but occasionally delayed until adulthood when the cyst causes symptoms [10–12]. The origin of vitreous cyst is speculative, with some observations supporting a choristomatous malformation related to failed regression of the hyaloid vascular system. Other evidence suggests these cysts arise from developmental defects of the retinal pigment or ciliary epithelia. Most vitreous cysts reported in the literature have been free-floating, but some are tethered to the optic nerve head. It is the latter group that implicates defective regression of the fetal hyaloid vascular system.
Clinically, vitreous cysts are nearly spherical and vary in color from gray to brown. On B-scan they appear as hypoechogenic masses. Their edges are hyperreflective. Those cysts studied histologically consist of a monolayer of cuboidal cells, either nonpigmented or partially pigmented with melanin. The cells are polarized with basement membrane on the internal surface [10]. The monolayer of cuboidal cells resembles retinal pigment or ciliary epithelia.
Congenital vitreous cysts need to be distinguished from acquired traumatic cysts, which also occur free-floating. Their morphology, down to the ultrastructural level, can be identical to congenital vitreous cyst [13]. The critical differentiating features of this form of acquired cyst are documented absence prior to trauma and clear evidence of traumatic injury.
8.4.3 Congenital Vitreous Liquefaction
Congenital liquefaction, or syneresis of the vitreous, is a manifestation of several inherited disorders including Stickler syndrome types 1 and 2, Wagner syndrome type 1, Goldmann-Favre syndrome (enhanced S-cone syndrome), and snowflake vitreoretinal degeneration. Wagner syndrome has been associated with a mutation in the gene encoding for chondroitin sulfate, one of the minor proteoglycans of the vitreous [14]. Although early onset syneresis is a consistent feature of these vitreoretinal syndromes, histological examination of the vitreous has no routine role in clinical evaluation. Each of the above named syndromes has prominent retinal findings and, in the case of Stickler syndrome, characteristic systemic abnormalities as well [15].
8.5 Inflammation
Vitreous inflammation is virtually always a manifestation of disturbances originating outside the vitreous cavity, such as trauma, primary disorders of neighboring tissues (e.g., uveitis, scleritis, etc.), or systemic disease (e.g., sepsis, vasculitis, etc.). While the potential causes of vitreous inflammation are vast, the mechanisms of vitreous injury share common pathways. Regardless of underlying type of injury, plasma proteins and polymorphonuclear leukocytes (neutrophils) enter the vitreous, followed by macrophages. Enzymatic breakdown of the collagen latticework begins almost immediately. The result is destruction of glycosaminoglycans, collapse of the fibrillar cytoarchitecture, and liquefaction of the gel. Aggregates of insoluble proteins and clumps of inflammatory cells cause varying degrees of opacification. In terms of cellular infiltrate, vitritis traverses the spectrum of acute to chronic inflammation, including granulomatous. Since exclusion of infectious disease is a priority when confronted with vitritis, biopsy for culture and other ancillary studies play a key role in clinical evaluation.
8.5.1 Infectious Endophthalmitis
8.5.1.1 Purulent Endophthalmitis
Infectious endophthalmitis following surgery, accidental trauma, or sepsis is characterized by acute inflammation of the vitreous or, less commonly, a mixture of acute and granulomatous inflammation. How the infectious organism gained entrance to the eye (e.g., cornea, surgical wound, blood-borne, etc.) determines the general pattern of ocular inflammation. Regardless whether the trauma is surgical or accidental, the earliest and often the most intense inflammation is associated with the location of microbial contamination (Fig. 8.4). Unlike externally acquired infections, blood-borne pathogens most commonly seed the posterior segment of the eye, often in multiple locations (Fig. 8.5a, b). Even low-virulence microorganisms like Staphylococcus epidermidis can result in highly destructive infections inside the eye given the vulnerability of intraocular tissues and the lack of local defense mechanisms [16]. In end-stage endophthalmitis, inflammation may be so widespread that determining the anatomic starting point may no longer be possible. Tissue Gram stain, while critical for rapid identification of putative organisms, is relatively insensitive and nonspecific compared to culture or new molecular microbial technologies. Despite the limitation of tissue Gram stain, large colonies of bacteria can often be found in purulent endophthalmitis (Fig. 8.6). Since melanin granules approximate the size and shape of bacterial cocci, microscopic screening for microorganisms is tedious.
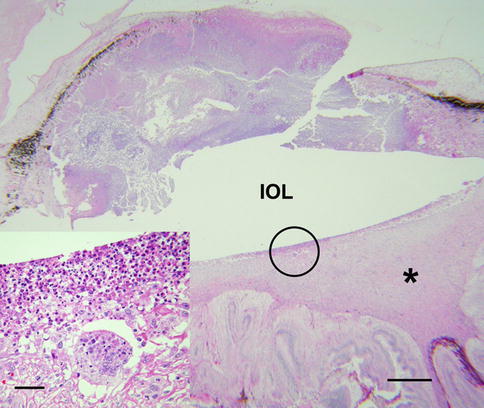
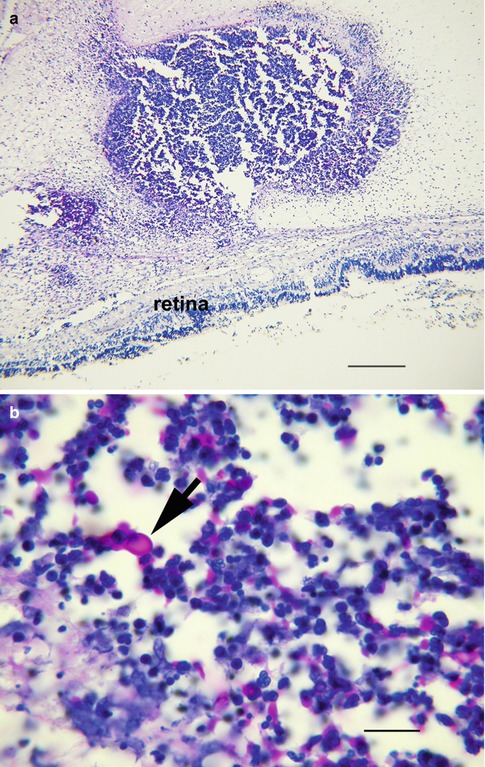
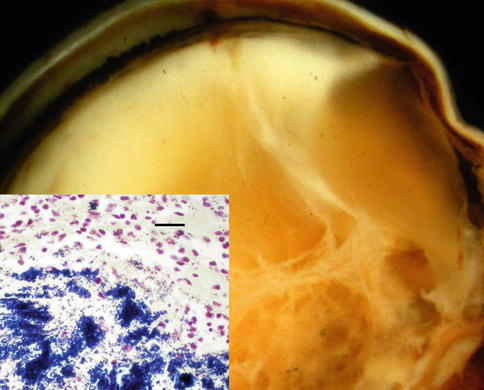
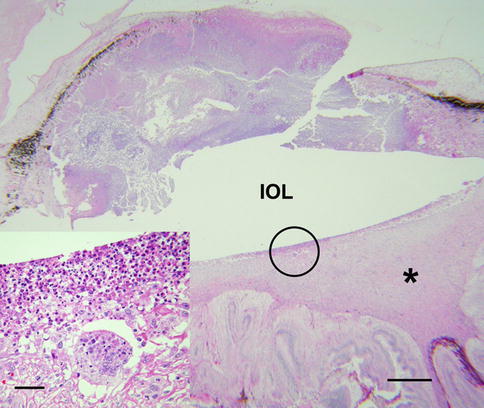
Fig. 8.4
Fungal endophthalmitis following cataract extraction shows purulent exudate anterior to the intraocular lens (IOL) implant. The vitreous is organized and chronically inflamed. The retina is detached (bar = 800 μ). Inset shows the field within circle. There is suppurative inflammation immediately behind the IOL with necrosis, acute inflammation, and giant cells (bar = 20 μ)
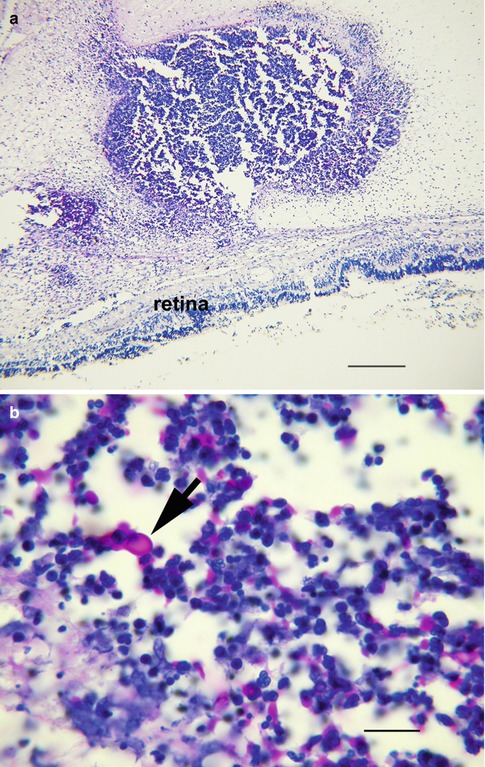
Fig. 8.5
(a) Endophthalmitis due to blood-borne infection with Candida albicans. A vitreous abscess is present immediately anterior to the retina (bar = 250 μ). (b) High magnification of a shows yeasts within the abscess (periodic acid-Schiff stain; bar = 35 μ)
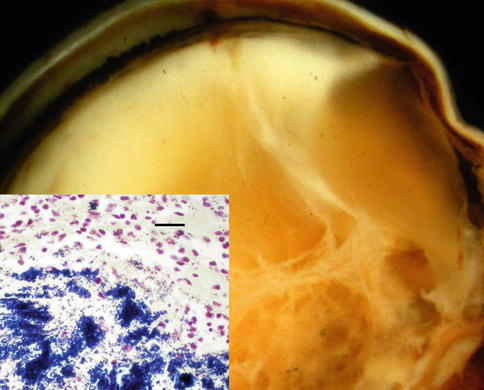
Fig. 8.6
Suppurative endophthalmitis due to a viridans streptococcus. The internal structures of the eye are effaced by purulent exudate. The vitreous has been transformed into loculated pus. Inset shows myriad bacteria with tissue Gram stain (Gram stain; bar = 30 μ)
Essentially, any infectious organism or parasite can result in vitritis. Although fungi may not have as explosive a clinical onset as bacterial infection, most cause suppurative inflammation within the eye, with a substantial minority inducing a mixed purulent-granulomatous pattern of inflammation. Special stains for fungus (periodic acid-Schiff or Gomori methenamine silver) are invaluable diagnostic aids (Fig. 8.5b).
8.5.1.2 Viral Endophthalmitis
Viral endophthalmitis is an elusive nosological entity, overlapping conceptually with viral uveitis, a term that implies the uveal tract as the target tissue, and viral retinitis, a relatively specific clinical condition. Since viruses require living cells to replicate, the hypocellular vitreous is an unlikely host tissue for viral infection. This is not to say that viral particles and viral DNA cannot be found in the vitreous because they are, but usually when the retina or retina-choroidal complex is the primary site of infection. This spillover phenomenon is usually accompanied by varying degrees of retinal inflammation. Spillover vitritis has been documented for retinitis due to herpes simplex type 1, herpes simplex type 2, varicella-zoster virus, cytomegalovirus (CMV), West Nile virus, and Rift Valley virus [17–20].
The clinical diagnosis of a spillover vitritis due to virus is often based on the funduscopic appearance of the retina. The clinical pattern described as acute retinal necrosis (ARN), for instance, may not be pathognomonic of a single virus, but it restricts the differential diagnosis to several principle viruses [17]. Systemic evaluation and ancillary studies enhance diagnostic certainty, often obviating the need of biopsy for culture or polymerase chain reaction (PCR). Studies of spillover vitritis have shown that PCR assays performed on aqueous humors have comparable sensitivity and specificity as samples harvested from vitreous [21].
Retinitis due to coxsackievirus, Epstein-Barr virus, rubeola virus (both congenital and acquired), and human immunodeficiency virus usually does not result in clinically significant vitreous inflammation [22].
Presumed herpetic vitritis, also referred to as herpetic uveitis, can occur in the absence of retinal inflammation [17]. These cases present as so-called idiopathic vitritis, though most patients have a history of past herpetic eye disease. The diagnosis is confirmed by harvesting vitreous for viral culture or PCR [17].
Is it possible that other viruses might be responsible for idiopathic vitritis (or uveitis)? One study examined vitreous samples from over 100 patients with retinitis or vitritis for DNA from human herpesvirus 6A, 6B, and 7. Using PCR, they reported detectable viral DNA from less than 2 % of samples from patients with inflammation [23]. The search for putative viral pathogens is ongoing.
Histopathology: The histopathology of viral vitritis is documented haphazardly in the literature in studies dealing with idiopathic uveitis and posterior segment inflammation of unknown cause [18, 22, 24, 25]. Vitreous samples reveal nonspecific chronic inflammation. Unless an inadvertent piece of retina is mixed in the sample, cytopathic viral changes are usually not observed.
8.5.1.3 Low-Virulence Bacterial Endophthalmitis
Propionibacterium acnes endophthalmitis is a delayed-onset infection following cataract surgery with implantation of a posterior chamber lens. Clinical manifestations begin several weeks or months after the procedure. Its pathogenesis and histological findings are distinctive enough to deserve separate discussion. A commensal microbe of skin and lid margin, P. acnes is a low-virulence Gram-positive coccus. The bacterium is introduced into the capsular bag at the time of surgery when the artificial lens is inserted into the eye. Bacteria dragged into the eye are wedged between lens capsule and lens implant, where they grow relatively unimpeded. A chronic low-grade uveitis weeks or months after cataract surgery is often the first sign of intraocular infection [26]. White plaques within the capsular bag, often near the equator, are seen in some cases. These plaques correlate with myriad bacteria sequestered between folds of lens capsule or between lens capsule and lens implant. Anterior chamber inflammation may demonstrate large or mutton-fat keratic precipitates. Neodymium-yttrium-aluminum-garnet (YAG) laser capsulotomy can release bacteria into anterior vitreous, provoking more intense inflammation. Vitrectomy and posterior capsulotomy are necessary when uveitis fails to respond antibiotic therapy.
Vitreous harvested at surgery yields P. acnes, although the organism may take weeks to grow in culture. Histologically, the Gram-positive organism is usually found next to lens capsule, often without significant contiguous inflammation (Fig. 8.7)
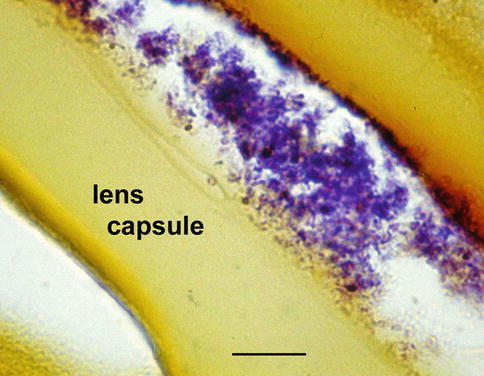
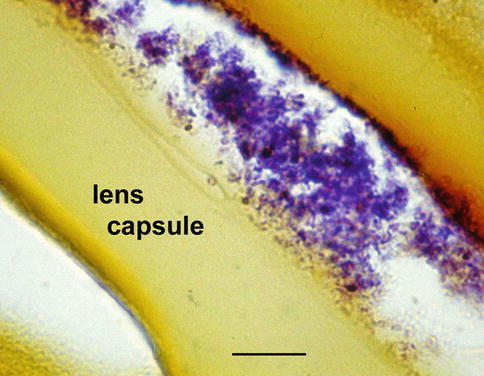
Fig. 8.7
Chronic vitritis after cataract surgery due to P. acne infection. Lens capsule was removed during therapeutic vitrectomy. Markedly degenerating, Gram-positive organisms are present without inflammation (tissue Gram stain; bar = 6 μ)
Similar clinical scenarios have been reported with other low-virulence organisms, such as S. epidermidis, Corynebacterium, Achromobacter, and several types of fungi (Fig. 8.8).
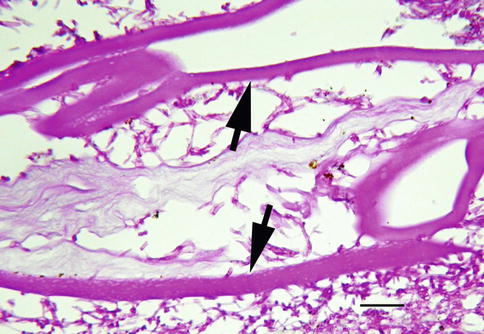
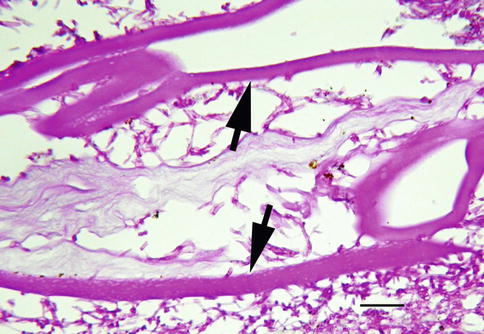
Fig. 8.8
Surgically removed lens capsule for low-grade uveitis following cataract surgery shows folded lens capsule (arrows). The hematoxylin-eosin stain reveals numerous fungal hyphae in the absence of inflammatory cells (bar = 15 μ)
8.5.2 Whipple’s Disease
Whipple’s disease is a chronic infection of Tropheryma whipplei, a fastidious intracellular bacillus that cannot be routinely cultured [27]. The epidemiology of Whipple’s disease is incompletely understood and had been considered for many years an intestinal dystrophy characterized by infiltration of the small bowel with PAS-positive macrophages. It is now known that those PAS-positive cells contain the causative organism and that the clinical spectrum of the disease is much wider than originally believed. The organism is known to involve the central nervous system, joints, lymph nodes, and the eye, where it most commonly presents as vitritis (uveitis) [28]. The diagnosis of ocular Whipple’s disease is elusive and might not even come to mind in the absence of malabsorption syndrome.
Vitreous biopsy of Whipple’s disease shows atypical macrophages with partially vacuolated cytoplasm (Fig. 8.9). The cells may appear less “foamy” than those seen in the small bowel and can resemble macrophages infected with M. avium-intracellulare. Cells similar to Whipple’s cells have been described in treated endophthalmitis from a coryneform bacterium (Fig. 8.10) [29]. Even though Tropheryma whipplei cannot be routinely cultured, vitreous fluid can be collected for polymerase chain reaction, which is highly sensitive and specific in identifying the organism [30].
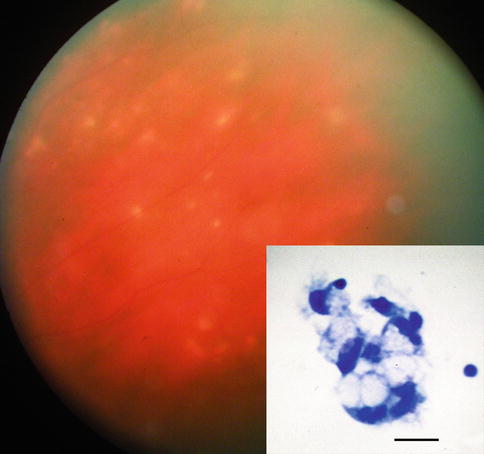
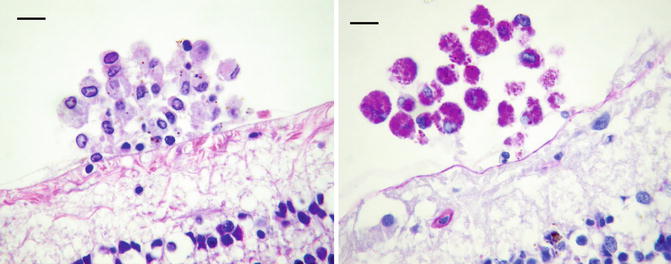
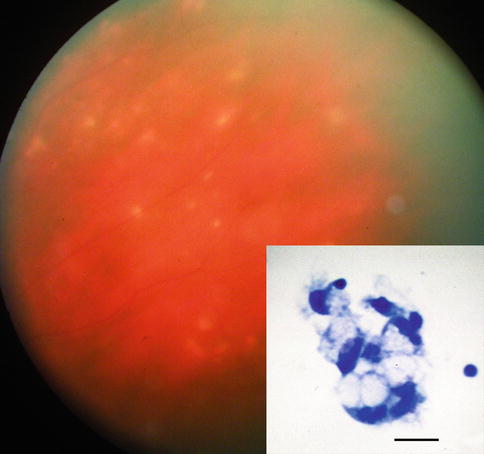
Fig. 8.9
Fundus photograph of 68-year-old woman with bilateral uveitis due to Whipple’s disease. Small hypopigmented lesions of the retina are noted. Inset shows clump of macrophages with vacuolated cytoplasm from vitreous biopsy, which leads to confirmatory jejunal biopsy (bar = 20 μ) (Courtesy of Alan Friedman, MD. Presented at 1986 Eastern Ophthalmology Pathology Society Meeting)
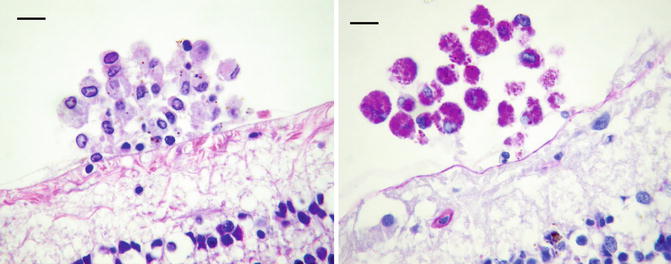
Fig. 8.10
Treated coryneform bacterium endophthalmitis shows a clump of macrophages with pale cytoplasm resting near the retina on left. With PAS (on the right) the cells are filled with bright magenta granules. The eye was obtained at autopsy (death unrelated to surgery). The macrophages are virtually indistinguishable from cells in Whipple’s disease (bars = 20 μ)
8.5.3 Vitreous Inflammation from Parasites
8.5.3.1 Toxocara Endophthalmitis
Human infection with Toxocara canis is acquired through the ingestion of soil contaminated with encysted larva. This intestinal roundworm is a cosmopolitan parasite ubiquitous in the dog family, but its life cycle cannot be completed in humans. It is the second or third larval stage of the parasite that gives rise to the human disorder known as visceral larval migrans (VLM), a disease seen primarily in children. The average age at diagnosis is 7 years, but in some regions the peak incidence is between 1 and 3 years. The majority of those infected present before the age of 16 [31]. Not all children infected with T. canis are symptomatic with VLM, which usually causes low-grade fever, coughing or wheezing, lassitude, and weight loss. Some children escape the systemic illness related to generalized migration of the larvae, only later to present with isolated end organ disease. Ocular infection is almost always due to a single larva. Though theoretically the larva could affect any portion of the eye, many appear to enter the globe at or near the optic disc before dying in the vitreous.
Clinical Features: Ocular toxocariasis usually presents as a single mass lesion either in or beneath the retina or in the vitreous, associated with inflammation [32]. Often a vitreoretinal membrane connecting the optic disc to the inflammatory mass can be seen ophthalmoscopically. This membrane represents the presumed larval path prior to death. More advanced cases are characterized by either heavy vitritis, which precludes direct visualization of the retina, or a white pupil reflex (leukocoria).
The cellular responses to dead and dying larvae are similar regardless of the tissue involved, but when T. canis dies within vitreous, the reaction can be exuberant, particularly in terms of fibroplasia (Fig. 8.11a).
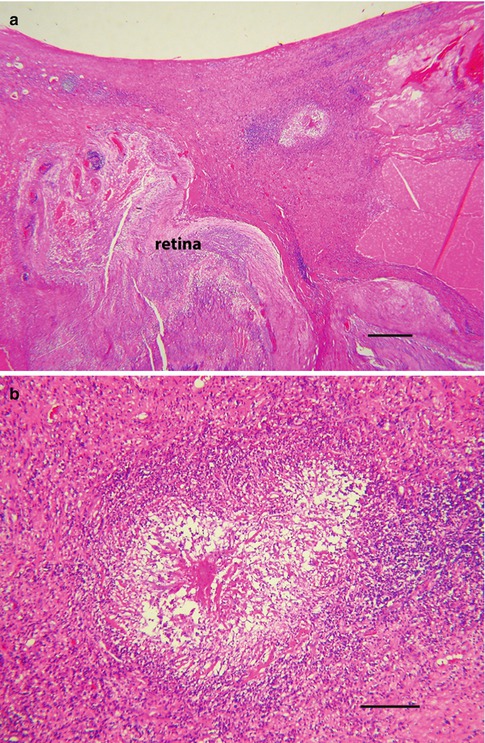
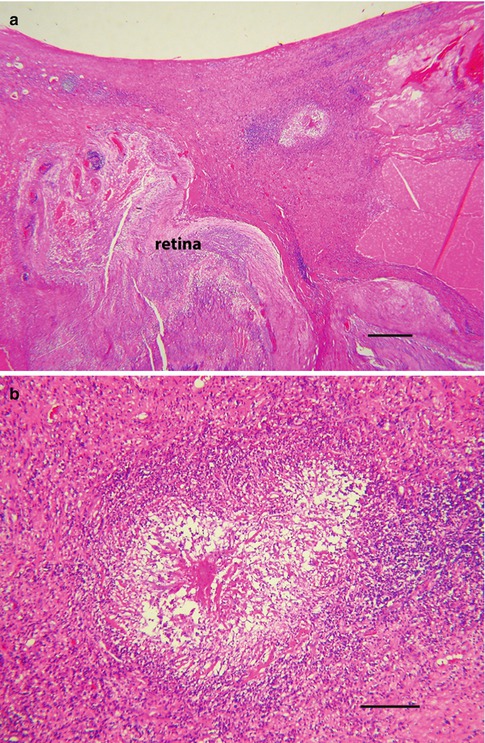
Fig. 8.11
(a) Toxocara endophthalmitis in a young boy. The retina is detached and the vitreous is replaced with inflamed fibrous tissue. Centrally within the densely collagenized vitreous is an area of necrosis (bar = 130 μ). (b) Higher magnification of a shows the area of necrosis and chronic inflammation, the presumed site of a degenerated nematode. The clinical diagnosis was supported by high ELISA titers for T. canis (bar = 35 μ)
Histopathology: The early cellular response is characterized by eosinophils and plasma cells. An eosinophilic abscess is a histological hallmark of the infection and marks the presumed location of larval death (Fig. 8.11b). Plasma proteins and degranulating eosinophils mixed with other acute inflammatory cells give the tissue a smudgy pink color with hematoxylin-eosin stain. This amorphous hyaline material is referred to as the Splendore-Hoeppli phenomenon and is associated with a variety of parasites and fungi (Fig. 8.12) [33]. Granulomatous inflammation is also a frequent finding, but is usually focal and can include multinucleated giant cells of the foreign body type (Fig. 8.13).
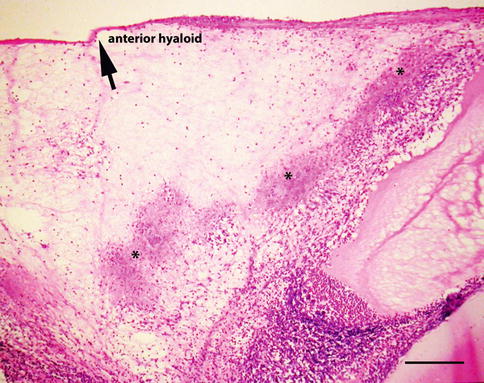
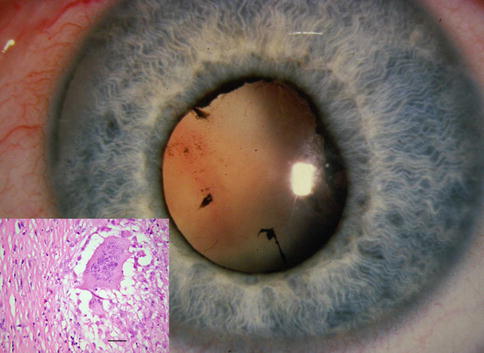
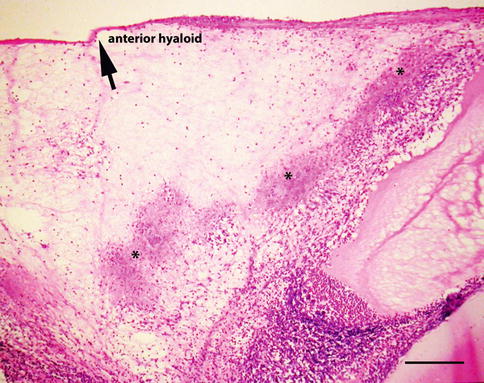
Fig. 8.12
Splendore-Hoeppli phenomenon in a case of clinically supported Toxocara endophthalmitis. The smudgy pink deposits mark the assumed path of the dying larva (bar = 95 μ)
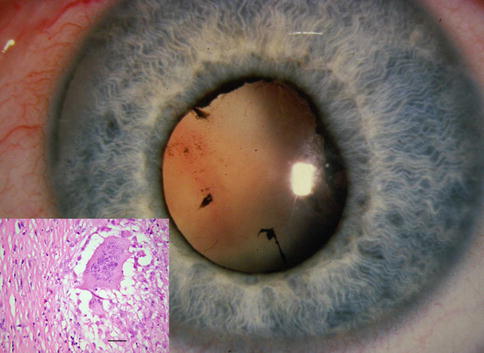
Fig. 8.13
Toxocara endophthalmitis in a child with leukocoria. Inset of the enucleated eye shows vitreous fibrosis with a single focus of granulomatous inflammation. Multinucleated giant cells were found at the presumed site of nematode death (bar = 20 μ)
T. canis is about 400 μ in length and 15–20 μ in diameter. Fragments of the dead organism can be difficult to find in tissue section, and seldom has a completely intact larva been observed. The outer cuticle of the nematode encircles its digestive tract and rarely escapes partial destruction. Larvae dying in or near the vitreous will incite a profound inflammatory reaction. The chances of sampling an organism on vitreous biopsy are remote, but the inflammatory reaction often includes eosinophils and plasma cells. Neglected cases can proceed to retinal detachment followed by chronic inflammation and scarring. Exuberant fibroplasia resulting in replacement of the vitreous with dense collagen has been referred to as sclerosing endophthalmitis. Although fibrous replacement of the vitreous is not specific for toxocariasis, few other disorders, particularly in childhood, have been associated with this robust pattern of scarring. Even at a late stage of tissue repair, eosinophilic abscesses and portions of larva can be found (Fig. 8.14).
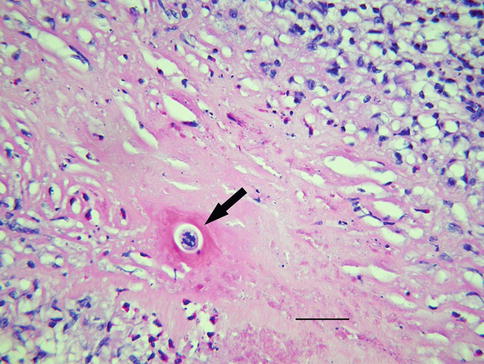
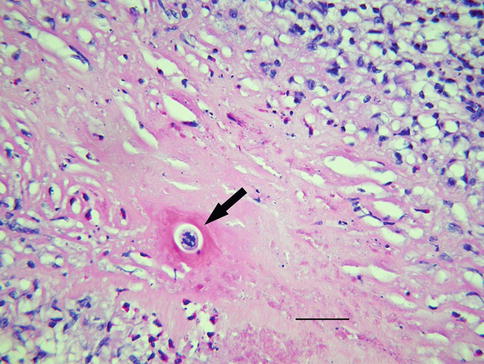
Fig. 8.14
Minute fragment of presumed helminth (arrow) embedded in dense fibrous tissue that replaced the vitreous (bar = 25 μ)
Laboratory studies play a role in the evaluation of children suspected of having ocular toxocariasis. Since ocular manifestations of T. canis typically are temporally removed from systemic dissemination of larva, eosinophilia is usually not present. The enzyme-linked immunosorbent assay (ELISA) for T. Canis has good diagnostic sensitivity, but some assays cross-react with other helminths. Strong presumptive evidence of ocular toxocariasis exists when ELISA titers from aqueous or vitreous fluids are higher than simultaneous serum samples [34].
8.5.4 Other Nematodes
Diffuse unilateral subacute neuroretinitis, or DUSN, is a generalized inflammatory disorder of the retina, choroid, and optic nerve. This unilateral inflammatory condition has been linked to infection with two related intestinal nematodes found in raccoons and squirrels. The putative worms are distinguished clinically by their size. The larger Baylisascaris procyonis measures about 2 mm, while the smaller Ancylostoma caninum is 0.4 mm [35, 36]. The clinical diagnosis depends on identifying the worm at the slit lamp. It is usually found beneath the neurosensory retina. The heat of a laser can provoke the worm to move. On occasion, worms have been found in the vitreous, where they can be extracted surgically [36].
Live nematodes have been reported in the vitreous of persons infected with the larva stage of Gnathostoma, a parasite endemic in Southeast Asia and India [37]. Humans acquire the infection by eating raw or undercooked freshwater fish. Subcutaneous infection around the eye precedes ocular involvement. The larva gains access to the conjunctiva and the deeper tunics of the globe in route to the vitreous. The larva is accompanied by vitreous inflammation and, occasionally, hemorrhage.
Microfilariae capable of invading the vitreous include the larva stage of Onchocerca volvulus, the microfilaria of Wuchereria bancrofti, and the third stage larva of Angiostrongylus cantonensis [38]. Vitreous involvement with these particular nematodes occurs with signs of widespread ocular inflammation. Vitreous involvement with Angiostrongylus cantonensis is associated with eosinophilic meningitis [38, 39].
8.5.5 Cestodes
Taenia solium, or pork tapeworm, gives rise to the human infection taeniasis or cysticercosis. The life cycle begins with the adult worm inhabiting the small intestine of humans and culminates with the larval stage and systemic dissemination. Cysticercosis occurs worldwide and is acquired by eating raw or undercooked pork. Usually, one adult worm lives in the small intestine of humans, but it can attain a length of 2–4 m. The human host must shed gravid proglottides before the pig (intermediate host) can complete the life cycle. After the pig ingest proglottides, eggs hatch inside the intestine and then disseminate through the blood and lymphatics, finally lodging in the muscle. Humans eating inadequately cooked pork that contains cysticerci (or auto-infect themselves with eggs or proglottides) are at risk of developing the disseminated larval stage of the disease.
The larvae of Taenia solium most often invade the subcutaneous tissue, muscle, brain, and eye. Those that migrate to the eye have been reported in conjunctiva and beneath the retina [38]. Cysticerci can enter the vitreous where they develop into cysts, ranging upward to 8 mm in diameter. The white scolex, which moves when irritated by the light of the ophthalmoscope, is a key diagnostic finding. Cysts can be removed surgically and the diagnosis confirmed histologically [40]. Cysts are filled with turbid fluid. Microscopically, the cyst wall is composed of an outer cuticle and inner layer of smooth muscle (Fig. 8.15). The scolex contains a sucker and hooklets.
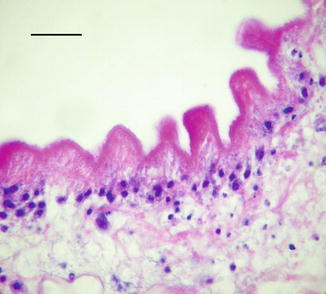
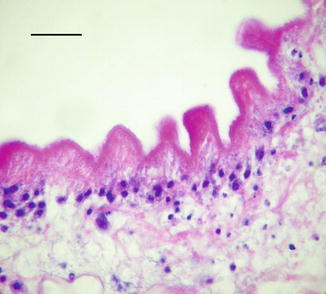
Fig. 8.15
Cysticercus removed from the eye surgically. The cyst wall is nodular. This region of the tegument lacks discernible smooth muscle; only degenerating nuclei are visible beneath the outer cuticle (bar = 25 μ)
8.5.6 Vitreous Ophthalmomyiasis
The larvae of parasite flies of humans belong to the order Diptera. Members of this order are also important vectors of bacterial, viral, and protozoan diseases. The larvae (commonly referred to as maggots) are capable of invading the eye, where they can be found in the vitreous (Fig. 8.16). Ophthalmomyiasis is the term given to maggots that burrow through ocular tissues. Most of these parasite flies belong to the genus Cuterebra [41].
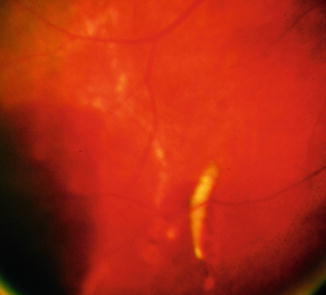
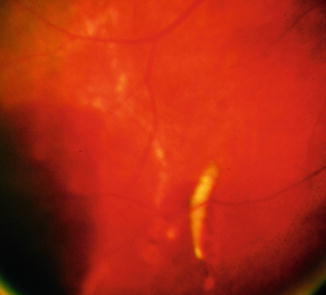
Fig. 8.16
Fundus photograph of ophthalmomyiasis and vitreous hemorrhage. A little maggot resides in the subretinal space contemplating a sojourn in vitreous
8.6 Autoimmune Vitritis and Spillover
A variety of autoimmune diseases and uveitic syndromes are associated with vitritis. This heterogeneous group of disorders includes such entities as sympathetic ophthalmia, birdshot retinochoroidopathy, and Vogt-Koyanagi-Harada syndrome to name a few. Vitreous inflammation in these conditions is considered spillover from the primary site of involvement. Clinical diagnoses usually depend on recognition of other key findings and ancillary laboratory studies. Vitreous biopsy is usually considered to have a role in this setting when clinical features are insufficient to exclude an infection or neoplastic vitritis.
8.6.1 Phacoantigenic Uveitis
Phacoantigenic (phacoanaphylactic) uveitis is a morphologically distinct autoimmune disorder of the eye. Its pathogenesis is thought to represent an immunological and cellular reaction to normal lens protein following exposure of sequestered proteins by accidental or surgical trauma. Lens proteins are isolated from the immune system throughout life by a thick basement membrane (lens capsule), making them theoretically foreign antigens to the body’s immune system. Vitritis is a consistent clinical manifestation of phacoantigenic uveitis, although other complications may dominate the clinical picture.
The inflammatory reaction directed against normal lens is usually intense, initially involving neutrophils and then lymphocytes and epithelioid histiocytes (Fig. 8.17). A mixture of both acute and chronic granulomatous inflammation is almost always seen histologically. Inflammatory cells intimately surround exposed lens, forming a mantle of neutrophils and epithelioid histiocytes. If the inciting lens protein is not removed, acute inflammatory cells are eventually replaced with histiocytes and fibrous tissue. The pattern of chronic inflammation may become that of a palisading granuloma, with large histiocytes oriented perpendicular to residual lens. Neglected phacoantigenic uveitis causes considerable ocular morbidity. Not unexpectedly, phacoantigenic uveitis is associated with sympathetic uveitis, since both conditions are causally linked to trauma. Treatment involves surgical removal of the inciting lens material, plus adjuvant medical therapy to control inflammation (see also pages 30 and 183).
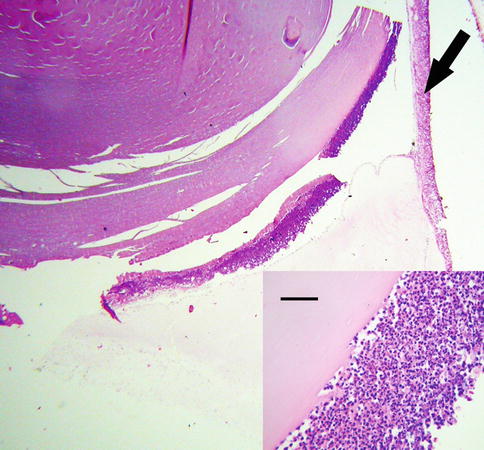
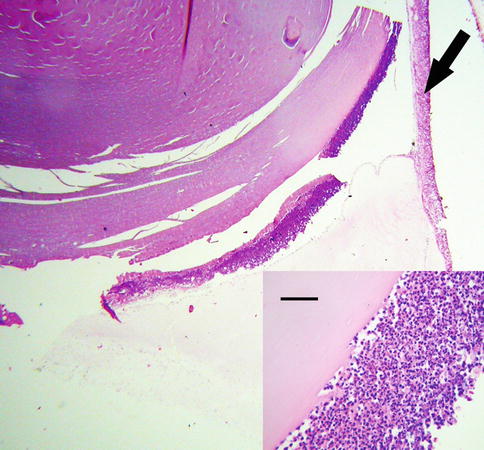
Fig. 8.17
Phacoantigenic endophthalmitis with dislocated lens in the vitreous. Arrow points to the retina. Inset shows higher magnification of the lens, which is covered posteriorly by a rim of acute inflammatory cells. There is no lens capsule (bar = 35 μ)
8.7 Hemorrhage
Since the presence of blood in the vitreous is abnormal, the determination of the underlying cause of bleeding is essential. From the pathologist’s vantage point, the diagnostic problem is twofold: first, to accurately identify red blood cells (and, if possible, estimate the stage of catabolism) and, second, to determine the source of bleeding. The second objective may not be possible if working with a limited sample such as vitreous biopsy, but may be achievable when examining the eye in its entirety [42].
The precise location of blood in the vitreous space affects its clinical features and determines the rate of cellular catabolism. Hemorrhage within the gel differs from retrohyaloid hemorrhage because blood in the later space is located outside the vitreous proper (Fig. 8.18). Blood in the retrohyaloid space sits between the posterior hyaloid surface and internal limiting membrane and tends to thrombose and lyse more rapidly than blood when confined to vitreous [43].
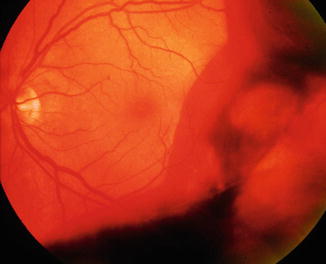
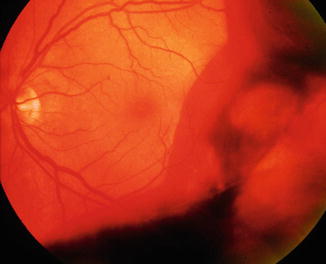
Fig. 8.18
Vitreous hemorrhage obscures visualization of the retina. Red blood cells or varying density allow partial to no view of retina
Etiology: The causes of vitreous hemorrhage are vast, from trauma and diabetic retinopathy to macular degeneration and blood dyscrasia [42]. In clinical practice, the most common causes of vitreous hemorrhage are retinal neovascularization (most often due to diabetes and retinal vascular occlusive disease) and retinal tears involving overlying (bridging) vessels, usually associated with age-related vitreous syneresis. Similarly, an acute posterior vitreous detachment can also de-roof a superficial retinal blood vessel resulting in vitreous hemorrhage. Identifying the source of vitreous hemorrhage, for which clinical algorithms exist, is beyond the scope of this chapter.
Blood that enters the vitreous degrades differently from blood in other extravascular sites, as the vitreous modulates the interaction of red blood cells with other cells and with enzymes. Red cells in the vitreous form a fibrin thrombus relatively quickly, but the cellular response of neutrophils and macrophages is delayed. Because of the lack of intrinsic vasculature and the inhibitory effects of vitreous on phagocytosis, the breakdown of red blood cells is prolonged in vitreous compared to other connective tissues [44, 45]. Erythrocytes release iron when hemolyzed, making it possible for unbound ions to induce free radical injury. The vitreous, unlike most other tissues, can have a substantial number of erythrocytes escape hemolytic destruction, giving rise to erythroclasts, or ghost cells.
Observations in experimental studies and in humans show that iron is present in macrophages within 2–4 days after intraocular hemorrhage [42, 46, 47]. Most of these iron-bearing cells are found in or near the uveal tract, but with time intracellular catabolism of erythrocytes becomes more widespread. Histological evidence of red blood cell catabolism ranges from free erythroclasts (Fig. 8.19a) and macrophages stuffed with degenerating erythrocytes (Fig. 8.19b) to extracellular clear spaces (cholesterol clefts) with foreign body granulomatous inflammation (Fig. 8.20).
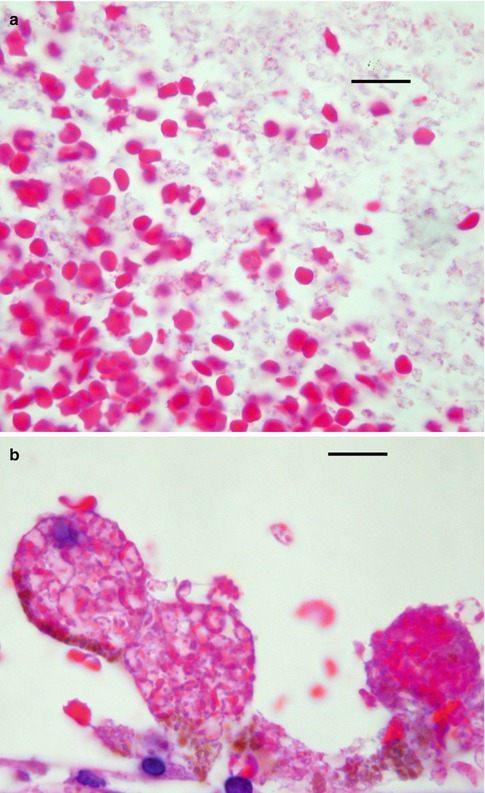
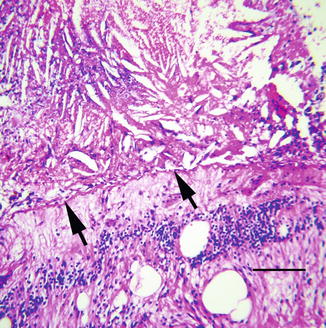
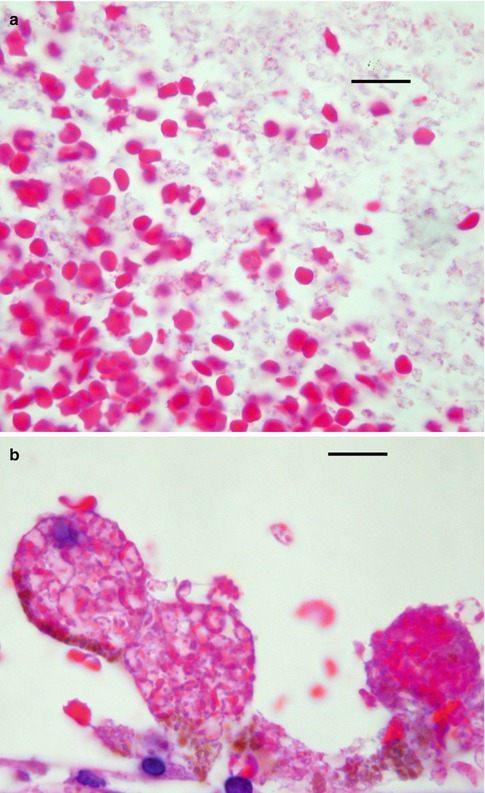
Fig. 8.19
(a) Vitreous hemorrhage shows both intact red blood cells to the left and erythroclasts to the right (bar = 20 μ). (b). Vitreous hemorrhage with macrophages filled with degenerating red blood cells (bar = 20 μ)
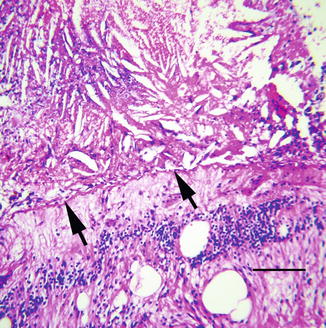
Fig. 8.20
Site of remote vitreous hemorrhage just anterior to internal limiting membrane (arrows) of retina shows numerous cholesterol clefts and chronic inflammation. Cystic edema is noted in the retina (bar = 60 μ)
Histopathology: Red blood cells that elude phagocytosis undergo slower, alternative forms of degradation. Hemoglobin from these cells is degraded through other pathways, but the walls of erythroclasts usually demonstrate deposits of denatured hemoglobin (i.e., Heinz bodies). Clinically, ghost cells residing in the vitreous appear as ochre-colored membranes. Histologically, the hollow carcasses of red blood cells are easily visible. With Giemsa stain, numerous Heinz bodies are seen stuck to the walls of these degenerating cells. Erythroclasts are relatively inflexible compared to normal erythrocytes. If ghost cells from a vitreous hemorrhage find their way into the anterior chamber, they tend to become lodged in the trabecular meshwork, causing elevated intraocular pressure, or so-called ghost cell glaucoma.
Extracellular breakdown of vitreous blood can also lead to the liberation of free hemoglobin from the red cell membrane, where, for reasons not understood, the molecules aggregate into 10–20 μ spherules (Fig. 8.21). These free, unbound clumps of hemoglobin are referred to collectively as hemoglobin spherulosis. The recognition of this morphological curiosity can be confirmed by immunohistochemical stains for hemoglobin A [48].
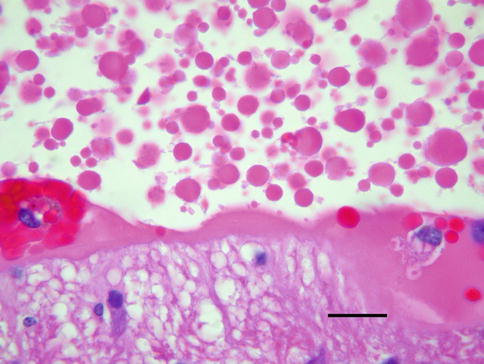
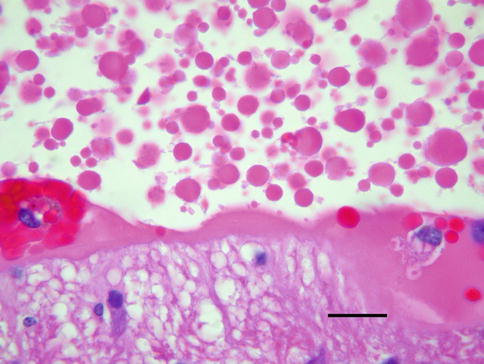
Fig. 8.21
Remote vitreous hemorrhage shows numerous globules of free hemoglobin, a process collectively referred to as hemoglobin spherulosis (bar = 20 μ)
The rate of clearance of vitreous blood is highly variable, ranging from months to 2 years, depending in part on the volume of initial hemorrhage and its location. Other than secondary syneresis, vitreous blood can also contribute to the formation and propagation of membranes.
8.8 Membranous Proliferations
The growth of membranes on the surface of the retina and in the vitreous cavity is a manifestation of a heterogeneous group of conditions. There is no universally accepted method of classifying vitreous membranes. One scheme based on anatomical and physiological criteria places membranes into three groups: (1) epiretinal membranes (ERM), (2) ocular ischemia-related retinal neovascular membranes, and (3) proliferative vitreoretinopathy (PVR). These membranes have overlapping characteristics morphologically and cytologically [49]. Unlike PVR and retinal neovascularization, however, ERMs are avascular.
8.8.1 Epiretinal Membranes
Epiretinal membranes are found on the inner surface of the retina and are composed of mixtures of glial cells, transformed retinal pigment epithelium (RPE), fibrocytes, macrophages, and myofibroblasts. Epiretinal membranes are associated with several common conditions like macular holes and retinal tears, but most have no identifiable cause (Fig. 8.22a). By default, this latter group is called idiopathic ERM. It is assumed that microscopic breaks in the internal limiting membrane are a conduit for cells to reach the retinal surface. The prevalence of idiopathic ERM increases with age. The predominant cell type in epiretinal membranes is a fibrous astrocyte or metaplastic fibroblast, but on occasion can consist of cuboidal cells growing in a monolayer (Fig. 8.22b). These cuboidal cells are a presumptively retinal pigment epithelium and may lack identifiable melanin. The pathology of ERMs is more thoroughly reviewed under disorders of the retina.
