Introduction
A knowledge of pathology is the key to good clinical practice, and the deeper this knowledge the better the practice. Less well recognized is that a knowledge of pathology also underpins much of the work of the ophthalmic and vision scientist and offers wide scope for research ideas. This chapter describes basic aspects of disease processes with reference to specific entities relevant in ophthalmology.
Cell and tissue damage
Cells and tissues die as part of normal physiological homeostasis and different tissues have different susceptibility to death. Indeed, different processes occur as cells die and are ultimately cleared away. Similarly, tissues have different capability for regeneration and this applies to all of the particular ocular tissues.
Agents or conditions that can cause direct damage to ocular cells and tissues
There are a wide variety of noxious agents, and processes or deficiencies capable of causing damage to the eye and some of these are described here.
Reduced oxygen supply
Central retinal artery occlusion causes reduced oxygen flow to the retina, which becomes opaque due to oedema.
Physical agents
- •
Mechanical trauma is the commonest form of ocular injury. Blunt trauma describes mechanical injury in which the globe remains intact. Anteroposterior deformation separates the delicate attachments between the intraocular structures, e.g. the zonular fibres, rupture of which causes lens dislocation. Separation of the attachment of the ciliary muscle to the scleral spur leads to collapse of the trabecular meshwork and secondary glaucoma (angle recession glaucoma). Penetrating and perforating injuries are caused by a wide variety of weapons, tools, sporting equipment and domestic utensils, and almost inevitably lead to severe disorganization within the ocular structures.
- •
Extremes of temperature such as cryotherapy are used surgically to induce an adhesive scar by freezing and thawing in the peripheral retina during the prophylaxis or treatment of retinal detachment. Cryoablation is also used to destroy the ciliary body in cases of intractable glaucoma, the aim being to suppress aqueous formation.
- •
Light exposure – excess exposure to ultraviolet light may lead to an overproduction of free radicals by photons (e.g. hydrogen peroxide, superoxides) with insufficient free radical scavengers (e.g. vitamin A, superoxide dismutase and glutathione transferase) with resultant tissue damage. For example, damage may occur to the corneal epithelium in snow blindness. Photon bombardment of the photoreceptors at the macula over six or more decades has been suggested as a contributing factor in age-related macular degeneration. Lasers used in ophthalmology cause tissue damage dependent on the wavelength, the site of absorption and the quantity of energy released. In panretinal photocoagulation, which is used to treat diabetic vasoproliferative retinopathy, individual burns destroy localized patches of the outer third of the retina and the underlying RPE cells. Reactive proliferation of RPE at the edge of the burn leads to clinically visible pigmentation around a white circle, which is the result of a ‘scar’ formed by glial cells. Transpupillary thermotherapy (TTT) uses infrared light to heat (to 40°C) choroidal melanomas and bring about tumour cell necrosis. Several types of laser are used in ophthalmology to treat various eye diseases, and newer lasers such as the nanolaser are continuing to be developed (see Table 9-1 ).
TABLE 9-1
Lasers used in ophthalmology
Type of laser
Wavelength (nm)
Application
Argon (CW)
Green (457, 488, 514, 610)
Ablation of RPE/outer retina (in diabetic retinopathy)
YAG/Nd
Pulse 1064
Disruption of lens capsular membranes; destruction of ciliary body (glaucoma)
Excimer (CW)
193
Radial keratometry
Remodelling of corneal surface; refractive keratometry
CW, continuous wave; YAG/Nd, yttrium-aluminium-garnet/neodymium.
- •
Ionizing radiation – each of the three types of ionizing radiation in common use in medical practice has applications in ophthalmology:
- •
charged particles (electrons and α-particles)
- •
uncharged particles (neutrons)
- •
electromagnetic radiation (X-rays and γ-rays).
- •
- •
Proton beam therapy is now an accepted choice for the external treatment of ocular melanomas, while γ-emitters such as 106 Ru and 60 Co can be applied topically to the sclera over a melanoma in the form of a radioactive plaque. X-rays and γ-rays are used for the external beam treatment of retinoblastoma.
Radiation from any source has a profound effect on nuclear DNA, with fracture of chromosomes, dislocation and translocations. The consequence is tissue destruction and suppression of cell division, which is essential for the ablation of tumour tissue. The side-effects for non-tumour tissues are:
- •
Endarteritis – infiltration of the vessel wall by inflammatory cells and proliferation of spindle cells (myofibroblasts, intimal fibroplasia) within the internal elastic lamina. There is endothelial cell swelling and fibrin deposition. Narrowing of the vessel lumen contributes to tumour destruction by ischaemic necrosis (see figure). A long-term secondary complication of radiation arteritis is dilation of the capillary bed (telangiectasia). Radiation vasculopathy causes leakage of plasma constituents in retinal vessel walls.
- •
Loss of hair, teeth and glandular tissue. Irradiation of the orbit damages lacrimal gland tissue and leads to a dry eye.
- •
Massive necrosis of normal tissue when the radiation dose is excessive.
- •
An increased risk of mutations, with malformation in offspring and the induction of a second malignant tumour.

Chemical agents
Toxic chemicals of any sort will damage the delicate ocular tissue when applied externally. Acid and alkali burns are encountered most commonly in clinical practice, but detergents can also cause significant damage.
- •
Acid burns – acids (hydrochloric, nitric and sulphuric) and acidic fluids coagulate proteins, so that diffusion through the cornea and the sclera into the eye may be limited. Necrosis of epithelial and stromal cells leads to reactive fibrosis in the conjunctiva with eyelid distortion (entropion and ectropion). Corneal scarring requires keratoplasty to restore vision.
- •
Alkali burns – alkalis, such as ammonia and sodium hydroxide, pass through tissue easily and the high pH is sufficient to destroy the cells of the lens, uveal tract and retina as the alkaline fluid diffuses through the vitreous as far as the optic nerve.
Toxins
Toxins are generally proteins derived from bacteria, plants and animals (e.g. snakes). Some have been purified and have value as therapy such as botulinum toxin. However, most toxins from organisms cause severe tissue damage, such as those from Pseudomonas aeruginosa which may cause a severe lytic keratitis (see Ch. 8 ). Tobacco and alcohol abuse may lead to toxic-nutritional optic neuropathy with damage to the papillomacular bundle. Similarly, many drugs used to treat systemic disease can have toxic side-effects for the retina, e.g. ethambutol for tuberculosis.
Viruses
Viruses that commonly cause ocular damage include herpes simplex virus and herpes zoster virus (see under Inflammation , p. 492 ).
Dysregulated immunity
Several autoimmune diseases, such as rheumatoid arthritis and multiple sclerosis, affect the eye and will be discussed under Inflammation. Those with reduced immunity due to human immunodeficiency virus may develop retinitis due to cytomegalovirus.
Nutritional deficiencies
Vitamin A deficiency leads to night blindness and later xerophthalmia and keratomalacia. Vitamin B 12 deficiency is implicated in tobacco/alcohol optic neuropathy.
Genetic abnormalities
It is not surprising that errors occur during complex genetic activities. Germ cells and proliferating somatic cells (including stem cells) are susceptible to such errors. When abnormalities arise in germ cells the abnormality may be transmitted to the next generation. Abnormalities in somatic cells may result in cell death or malignant transformation. Genetic abnormalities may involve whole chromosomes, parts of chromosomes, gene clusters or single genes.
Chromosomal abnormalities
- •
Polypoidy occurs when chromosomal numbers are increased by an exact multiple of normal. This may be seen in hypertrophied muscle cells and ageing cells but does not usually occur in gametogenesis as it is incompatible with life.
- •
Aneuploidy occurs when the number of chromosomes is increased, usually by one (trisomy). This may occur in Down syndrome.
- •
Structural abnormalities may arise during replication and may result in duplication and deletion of gene clusters of single genes.
Single gene defects.
These are caused by defects in single genes. They usually affect the structure of the resulting protein product and can have wide-ranging effects. They can be inherited as an autosomal dominant (e.g. neurofibromatosis type 1; Box 9-2 ), autosomal recessive (e.g. Wilson’s disease ( Box 9-3 ), Tay–Sachs disease) or sex-linked (e.g. some forms of retinitis pigmentosa) disorder. Whilst most genetic disorders occur in nuclear DNA, it should be remembered that mitochondria also contain DNA in chromosomes, which are self-replicating and encode enzymes involved in oxidative phosphorylation. When mutations occur in mitochondrial chromosomes, the defect is passed on via the ovum because the sperm does not have cytoplasmic constituents; thus mitochondrial disorders are maternally transmitted (see Ch. 3 , p. 138 and Box 9-4 ).
This is an autosomal dominant condition with almost complete penetrance due to a mutation in the NF-1 gene on chromosome 17. It is characterized by café-au-lait spots and neurofibromas on the skin. Ocular abnormalities include the following:
- •
Eyelids – café-au-lait spots and neurofibromas can affect the lids. Plexiform neurofibromas may cause cosmetic and functional problems.
- •
Orbit – proptosis may occur because of optic nerve gliomas or orbital plexiform neurofibromas. Bony defects may occur in the orbital floor or roof.
- •
Anterior segment – Lisch nodules, melanocytic hamartomatous lesions of the iris are a defining feature of NF1. Enlarged corneal nerves and conjunctival, episcleral and scleral involvement by neurofibromas may occur.
- •
Glaucoma – the major cause of glaucoma is infiltration of the angle by a neurofibroma obstructing aqueous flow. Goniodysgenesis may also occur.
- •
Retina and choroid – choroidal hamartomas consist of a mixture of melanocytic and neuronal elements. Hamartomas of the retina and retinal pigment epithelium may also occur.
- •
Optic nerve – optic pathway gliomas are the cause of most visual morbidity associated with NF1. They occur in 15% of patients and are usually juvenile pilocytic astrocytomas. They can involve the optic nerve, chiasm, optic tract and hypothalamus.
This is an autosomal recessive conditions due to mutations in the Wilson’s disease protein ( ATP7B ) gene which encodes a cation transport enzyme that transports copper into bile and incorporates it into ceruloplasmin. Abnormal transportation of copper results in its accumulation, principally in the liver and brain. This leads to cirrhosis and neurological and psychiatric symptoms.
The ophthalmic findings, which rarely cause significant visual loss, are:
- •
Kayser–Fleischer rings – these are rings in the cornea caused by deposition of copper in Desçemet’s membrane.
- •
Sunflower cataracts – due to brown or green pigmentation beneath the anterior lens capsule.
Inherited mitochondrial diseases are rare and mainly affect the central nervous and musculoskeletal system. Abnormal mitochondria are found in the periphery of skeletal muscle fibres and have a characteristic ‘ragged-red’ appearance with Gomori trichrome stain as shown in the figure below.

There are significant ophthalmic signs and symptoms in the following conditions:
- •
Leber’s hereditary optic atrophy – point mutations occur in mitochondrial DNA resulting in abnormal ATPase 6. Young males lose vision because of demyelination in the optic nerve; the papillomacular bundle is most severely affected.
- •
Chronic progressive external ophthalmoplegia (CPEO) – this is a slowly progressive, bilateral symmetrical ocular muscle dystrophy that starts in late childhood or early adulthood. Ptosis, external ophthalmoplegia and occasionally a pigmentary retinopathy occur.
- •
Kearns – Sayre syndrome – initial signs and symptoms are similar to CPEO but pigmentary retinopathy is more common with the greatest effect on the macula. Cardiac conduction abnormalities present after the development of ophthalmoplegia.
- •
MELAS syndrome (mitochondrial encephalomyopathy, lactic acidosis and stroke-like episodes) – onset is usually in childhood with muscle weakness, recurrent headaches, vomiting and seizures. Stroke-like episodes involve temporary weakness on one side of the body. Eye signs include external ophthalmoplegia, atypical pigmentary retinopathy and nuclear cataract.
Mechanism of cell death
Cells can die through a variety of processes, some of which cause more ‘bystander’ tissue damage than others. The nature of the injurious agent (e.g. if it is an organism, its virulence) or set of conditions (e.g. if it is a nutritional deficiency, then the level of deficiency) determines the outcome for the cell or tissue. Mild injury may be reversible. Severe injury will result in death either by necrosis or by apoptosis.
Necrosis
Necrosis describes death of a cell or group of cells, typically following severe hypoxia, physical or chemical injury. It is always pathological. There is a rapid depletion of intracellular energy systems. The cell membranes and intracellular organelles are disrupted and typically there is an inflammatory reaction.
Apoptosis
Unlike necrosis, apoptosis, or programmed cell death, is important in both health and disease. It is a rapid process usually affecting single cells within a population of healthy cells. Apoptosis is an active process in which proteins are cleaved by caspase enzymes which break up the nuclear scaffold and cytoskeleton (see Ch. 4 , p. 157 ). The caspases then activate DNAses which degrade nuclear DNA. The next phase involves early phagocytic recognition with expression of phosphatidylserine in the outer layers of the cell membrane due to flipping of phospholipid from the inner layers. The molecular events occurring in apoptosis are outlined in Figure 9-1 .

Sublethal cell injury
Various injurious agents will cause reversible cell damage without cell death. This may result in the following cellular changes:
Hydropic swelling.
This occurs when cells are incapable of maintaining ionic and fluid homeostasis. This leads to a loss of function of plasma membrane energy-dependent ion pumps. The cell and organelles become swollen, often with cytoplasmic vacuolation. Blunt trauma may cause widespread retinal oedema (commotio retinae), which may be caused by transient spasm of the retinal vessels, producing ischaemia and damage to endothelial cells with leakage into the tissue. However, it is equally likely to result from transient interruption of axoplasmic flow in the ganglion cell processes.
Atrophy.
This is a simple decrease in cell size or number resulting in shrinkage of affected tissues and organs. Blunt trauma may also cause shearing of photoreceptors, leading to atrophy of the photoreceptors and focal hypertrophy of the retinal pigment epithelium (RPE) (see below) which extends into the retina (pseudoretinitis pigmentosa).
Lesser degrees of damage may allow the cell time to adapt so that it is able to withstand injury. Mechanisms of adaptation include:
Hypertrophy.
This occurs when the cells increase in size, often as a result of increase in functional demand. This can occur in the RPE following injury (see above).
Metaplasia.
This is a change from one type of differentiated tissue to another. In dry eye there is a reduction in tear production and this results in a change of the normal conjunctival epithelium with goblet cells to stratified squamous type.
Healing and repair
The eye, like any other tissue, responds to injury by an initial acute inflammatory response followed by vascularization and wound closure in a fibroblastic scarring response. These responses may fundamentally alter the architecture and specific function in the tissue and can have serious effects on vision. For example, the wound response of the retina to retinal hole formation and detachment is to stimulate glial proliferation, termed proliferative vitreoretinopathy (PVR). This proliferation does not, however, restore retinal and choroidal integrity but has the opposite effect by contracting and shortening the retina, to the point that retinal reattachment may be surgically impossible. Similar serious consequences result from scarring in other ocular tissues.
Healing in the cornea usually leads to a corneal opacity (scar)
The corneal epithelium regenerates at the limbus (limbal stem cells, see Ch. 4 , p. 211 ) and spreads rapidly across the cornea. Bowman’s layer does not regenerate. Stromal keratocytes transform into fibroblasts to heal stromal wounds. Transparency is lost because the collagen fibres are not aligned properly (see Ch. 4 , p. 203 ). Desçemet’s membrane does not regenerate. The corneal endothelium fills in defects by sliding and in so doing deposits secondary layers in Desçemet’s membrane: the membrane is elastic and there is often recoil at the edge of a deficit.
Iris
The presence of fibrinolysins in the aqueous inhibits fibrin clot formation, and scar tissue does not appear in the iris stroma; this, plus the continuing flow of aqueous from posterior to anterior chamber of the eye, leads to the persisting patency of defects made by iridectomy and iridotomy procedures (with beneficial clinical results). Reactive proliferation of the iris pigment epithelium may occur in response to trauma.
Lens
The lens epithelium responds to some forms of trauma by undergoing fibrous metaplasia, but in general the lens does not heal in the true sense but rather responds to trauma by becoming opaque (cataract).
Retina
Damaged nerve cells are replaced by glial cells (gliosis), which are derived from perivascular astrocytes and Müller cells. In the RPE there is proliferation and metaplasia to fibrous tissue. A combination of glial cells and metaplastic RPE cells is found in preretinal membranes, particularly when such cells migrate via a retinal hole. In cases of retinal detachment, this can lead to extensive gliosis/fibrosis with a fixed, inoperable retina: this condition is termed proliferative vitreoretinopathy (PVR) and is the leading cause of failure in retinal detachment surgery. Currently there is no effective preventive treatment.
Choroid
The melanocytes of the choroid do not proliferate in response to trauma; scar tissue in the choroid is derived from scleral fibroblasts.
Sclera
Scars are formed by proliferation of episcleral fibroblasts.
Optic nerve
Trauma is followed by axonal loss and demyelination with reactive proliferation of glial cells and connective tissue cells.
Inflammation
What happens in acute inflammation?
Inflammation is the dynamic process by which living tissues react to injury ( Fig. 9-2 ). The injurious agent may be physical, chemical, infective or immunological. The classic signs of acute inflammation are redness, heat, swelling, pain and loss of function. These signs can be explained by changes occurring at the microscopic level. Hyperaemia is associated with microvascular changes and accounts for redness and heat. Following injury there is an initial period of vasoconstriction, followed by capillary and then arteriolar dilatation. This is due to the direct effect of injury on the vessels as well as release of chemical mediators from damaged cells. Exudation then occurs, accounting for swelling. This is the increased passage of protein-rich fluid through the vessel wall into the interstitial tissue. This increase in fluid results in dilution of toxins and provides protective antibodies, fibrin and various factors to promote healing. Passage of protein is mediated by direct endothelial injury and chemical mediators, including histamine, bradykinins and leukotriene. Fluid movement is due to increased filtration pressure in part due to hyperaemia and loss of proteins from the capillaries. Following exudation, leucocytes (neutrophils and monocytes) migrate to the site of injury. These cells come in contact with the vascular wall as the flow decreases (margination of neutrophils). They then migrate through the vessel wall by expression, initially of selectins (on endothelial cells), and later of integrins on neutrophils (see Ch. 7 , p. 386 ). Following transendothelial migration and extravasation, the subsequent movement of leucocytes is controlled by chemotaxis. The cells move in response to an increasing concentration gradient of the chemotactic agents. Chemotactic agents are released from other leucocytes (cytokines), complement components (C3a), arachidonic acid derivatives (leukotrienes) or pathogenic bacteria. Once within the tissues, leucocytes clear the injurious agent by phagocytosis. This process involves opsonization of bacteria by complement components before engulfment within the leucocyte. Once within the leucocytes the lysosomes fuse with the phagosome and bacteria may be killed by oxygen-dependent formation of free radicals or by activation of lysosomal enzymes. Various chemical mediators are also released from inflammatory cells or are present within plasma. Those released from inflammatory cells include histamine, serotonin, prostaglandins and leukotrienes as well as a range of cytokines. These mediators may result in vasodilatation (serotonin, prostaglandins), increased vascular permeability (histamine, leukotrienes) and lymphocyte proliferation and macrophage activation (cytokines). Those present in plasma are interrelated cascade systems, including the clotting cascade, fibrinolysis, complement system and bradykinin system. The outcome of acute inflammation depends on various factors, including the infecting organism and the extent of tissue necrosis. Inflammation may resolve, suppurate, repair with organization and scarring, or progress to chronic inflammation.

Inflammation in the eye has special features which are characteristic of the tissue involved. For instance, in the cornea a small traumatic erosion may resolve without scarring. However, bacterial keratitis may lead to formation of a corneal abscess that may ultimately heal with extensive scarring. Ongoing chronic inflammation may occur with herpes simplex stromal keratitis. The ‘privileged’ status of the eye modifies its response to trauma and infection and thus modifies the overall inflammatory response.
How does infection cause inflammation?
Infection occurs when microorganisms invade the sterile internal body tissues and then multiply. The local reaction to infections is usually inflammatory and is evoked by cell damage and death. There are many classes of organisms which cause inflammation (see Ch. 8 ) and each does so in different ways. Some of the pathology induced by these organisms is briefly described here.
Bacteria
Intraocular bacterial infections are rare but highly destructive. The pathogenicity of bacteria varies according to the species (see Ch. 8 , p. 473 ) and this is reflected in the nature of the immune response to the organism.
Pyogenic infection.
Pyogenic bacterial infection of the intraocular compartment is termed endophthalmitis and may be exogenous or metastatic. Panophthalmitis (in which the infection involves the whole of the ocular and periocular tissues) describes a rapid and devastating tissue destruction, which may be complete by 48 hours ( Fig. 9-3 ). Gram-positive cocci are among the commoner organisms that produce a purulent infection when introduced into the eye either by accidental or surgical trauma or by blood spread. The vitreous is an ideal medium for bacterial proliferation, in that the sites for ingress of inflammatory cells (e.g. retinal vessels, the optic disk and pars plana) are some distance from the proliferating pathogens. (Therapy may also be less than optimal because the barrier imposed by the endothelium of the retinal blood vessels impedes the diffusion of antibiotics; see Ch. 6 , p. 341 .)

Neutrophils are the predominant cell type in metastatic endophthalmitis, i.e. when bacteria enter the eye via the bloodstream, as in bacterial endocarditis or after contamination of an indwelling intravenous catheter. During bacterial killing, neutrophils may release lytic enzymes that can lead to the total destruction of all layers of the retina.
The ocular coats normally provide an excellent barrier to invasion by bacteria. However, if the cornea is in some way compromised, as for instance during the inappropriate use of steroids in corneal ulceration, the prolonged and incorrect use of contact lenses or inadequate wound closure after intraocular surgery, direct bacterial entry through the cornea may occur.
Several factors increase the risk of endophthalmitis ( Box 9-5 ).
- •
The major factor in non-accidental post-surgical endophthalmitis is active host infection, e.g. bacterial conjunctivitis.
- •
The major factor in accidental post-injury endophthalmitis is retention of infected foreign material, especially organic material.
- •
Poor surgical technique, inadequate wound closure and leaking blebs, e.g. after drainage procedures, can cause endophthalmitis.
- •
Contaminated intraocular lenses are a common cause of late low-grade bacterial endophthalmitis.
- •
Debility, chronic corneal ulceration and immunosuppression are rare causes of endophthalmitis.
- •
Contact lens wear with poor hygiene remains a cause of severe intraocular infection if corneal ulceration occurs.
Chronic infection.
The mycobacteria (tuberculosis, leprosy), the actinomycetes (skin and lung infection) and the spirochaetes (syphilis, yaws) are responsible for chronic destruction of tissue. Borrelia burgdorferi , a spirochaete transmitted by ticks, may cause arthritis, neurological disease and conjunctivitis (Lyme disease). Bartonella spp., which causes cat scratch disease, has also been implicated as a cause of neuroretinitis. Pathogens that produce a chronic infection induce humoral and cellular responses in the host (see Ch. 7 ); the latter is characterized by macrophages, lymphocytes and plasma cells. Many of the pathogenic organisms, such as Mycobacterium tuberculosis , have the capacity to survive within the host macrophage. In the case of M. tuberculosis , macrophages attempt to limit the spread of the organism by accumulating around the dead and dying (necrotic) cells killed by the organism. The macrophages then become more elongated, taking on an epithelial morphology (epithelioid macrophages). This collection of epithelioid macrophages is known as a granuloma ( Fig. 9-4 ). Fusion with neighbouring macrophages forms a characteristic multinucleate giant cell (Langhans cell). The central mass of dead tissue within the granuloma appears cheese-like macroscopically: hence, the terms ‘caseous necrosis,’ ‘caseating’ and ‘caseation’.

Viruses
The following viruses most commonly cause ocular disease: herpes simplex virus, herpes zoster virus (varicella group) and cytomegalovirus.
Herpes simplex keratitis.
Type 1 herpes simplex virus causes superficial corneal ulceration which is finger-like or dendritic within the epithelium. Primary herpes simplex infection usually occurs through the oral mucosa, the lips or the skin of the face. This is followed by transneural and, subsequently, latent viral infection in the neurones of the trigeminal ganglion and the sympathetic ganglia. The virus is morphologically detectable by ultrastructural investigation within the corneal cells (keratocytes and epithelial cells) and nerves ( Fig. 9-5 ). A variety of possible triggers (ultraviolet light, cold) reactivate the virus, which migrates along the sensory nerves to the corneal epithelium. The virus then invades, replicates and spreads within the epithelium. Effective antiviral therapy has dramatically reduced the complications of herpes simplex keratitis. These include chronic fibrosis and scarring (disciform keratitis) with stromal vascularization and persisting chronic inflammation: leakage of lipid-rich plasma into the corneal stroma leads to pale yellow deposits (secondary lipid keratopathy).

Herpes zoster ophthalmicus.
Herpes zoster virus infects the ganglia and branches of sensory nerves such as the trigeminal nerve. When the virus is reactivated, it replicates and produces vesicle formation in the skin in the distribution of the affected nerve or its branches. The eyelids, conjunctiva, cornea and uveal tract are involved in the inflammatory process. A lymphocytic infiltrate appears around the long and short ciliary nerves and is present in the choroid and ciliary body.
Acute retinal necrosis.
Acute retinal necrosis has only been recognized as a disorder in the past three decades. The condition can be unilateral or bilateral and usually occurs in immunocompetent individuals. Acute retinal necrosis is usually caused by infection with herpes simplex or varicella zoster virus. Morphological distinction of these viruses can be difficult in retinal biopsies, and polymerase chain reaction ( Box 9-6 ) or in situ hybridization ( Box 9-7 , Fig. 9-6 ) may be more helpful in providing an accurate diagnosis. Enucleation specimens show sectorial or massive haemorrhagic retinal necrosis, associated with vitreous exudation and choroidal inflammatory cell infiltration. Intranuclear viral inclusion bodies can be seen by light microscopy. Ultrastructural examination reveals viral particles within retinal neurones and within the RPE and vascular endothelium. The virion appears as a central electron-dense core with a surrounding layer (the capsomere) and an outer envelope. The dimension of the infective viral particle is 190–220 nm (see Ch. 8 ).
Polymerase chain reaction (PCR) involves the selective amplification of specific segments of DNA. The basic reaction involves repetitive cycles of DNA synthesis. Each cycle consists of three steps:
- •
Denaturation – the first step involves denaturation of the target nucleic acid, which renders it single stranded
- •
Annealing – denaturation is followed by annealing of synthetic oligonucleotide primers specifically designed to hybridize to the target nucleic acid region
- •
Extension – the third step involves extension from the annealed primer catalysed by a DNA polymerase enzyme. In a typical PCR analysis 20–40 cycles are carried out with successive products becoming templates for subsequent cycles such that there is exponential amplification of the target region.
The basic technique may be adapted for various applications.
1
Detection of Genetic Mutations and Deletions (E.G. Leber’s Hereditary Optic Atrophy)
- •
Direct sequencing of the PCR product
- •
Detection of single-strand conformation polymorphisms (SSCP)
- •
Detection of restriction fragment length polymorphisms (RFLP).
2
Detection of Pathogens
PCR is useful for detecting various pathogens, particularly viral pathogens (e.g. hepatitis B, cytomegalovirus, herpes simplex virus).
3
Detection of Changes in Gene Expression
Reverse transcription PCR is based on the comparison of the amount of PCR product generated with the amount produced from a known concentration or copy number of control amplification targets in the same reaction. This technique can be useful for studying clonality in lymphoma.
In this technique single-stranded complementary nucleic acid sequences can join with specific DNA or RNA sequences in cells or tissues and these hybridization sites can be identified by the addition of a fluorescent in situ hybridization (FISH) or enzyme-labelled probe.
- •
FISH is utilized to detect chromosomal gains, losses and translocations in neoplasms (particularly lymphoma).
- •
In situ hybridization may be used for assessing clonality in lymphoid proliferations and detecting virus nucleic acids in infections with Epstein–Barr virus or cytomegalovirus.

Progressive outer retinal necrosis.
In immunocompromised individuals, herpes simplex and herpes zoster viruses may cause destruction of the outer retina without the accompanying vitritis, retinal vasculitis or papillitis usually associated with acute retinal necrosis.
Cytomegalovirus retinitis.
Before antiretroviral therapy became available, cytomegalovirus retinitis was a common ocular infection in individuals with acquired immunodeficiency syndrome (AIDS). It is characterized by progressive areas of retinal necrosis, usually without haemorrhage. Infected cells are often enlarged with a characteristic owl-eye inclusion body ( Fig. 9-7 ).

Chlamydia
Chlamydia are a genus of bacteria that are considered to be unique in the sense that they are obligate intracellular microorganisms (see Ch. 8 ). On a global scale, ocular infection by Chlamydia trachomatis (see Ch. 8 ) is one of the commoner causes of human blindness. The organism is spread by direct contact and is also insect-borne. In an environment with poor hygiene the disease flourishes but the Chlamydiaceae respond to broad-spectrum antibiotics, particularly tetracyclines, and as living standards have improved, the incidence of blindness as the result of trachoma has declined ( Box 9-8 ). Chlamydial conjunctival infection in developed countries, for instance, is much less sight-threatening than in less developed nations and is associated with genitourinary infection.
Trachoma is described in four stages:
- •
In stage I there is epithelial infection with early lymphoid hyperplasia and polymorphs within the conjunctival stroma, which is oedematous.
- •
Stage II is often subgrouped into type A, in which the lymphoid follicular reaction predominates, or type B, in which there is fibrosis with the formation of papillae. The latter reaction probably represents the effect of secondary bacterial infection. The papillae are formed by fibrovascular proliferation within the thickened and inflamed stroma. The cornea is involved at this stage with ingrowth of a fibrovascular pannus onto the superior corneal periphery.
- •
Stage III is characterized by fibrous replacement of the inflammatory tissue.
- •
In stage IV there is contraction within the palpebral conjunctival stroma so that there is internal deformation of the lids (entropion) and trichiasis, which leads to abrasion of the cornea by the lashes. Suppression of tear production is the result of inflammation and fibrosis within the lacrimal gland and its ductular system. Secondary changes occur in the conjunctival epithelium, e.g. stratification and loss of goblet cells, and these also impair tear film stability.
Fungi
(see Ch. 8 ) In Europe the most important ocular fungal pathogens include:
- •
Candida spp.
- •
Aspergillus spp.
- •
Mucor spp.
Mucormycosis is the result of a blood-borne infection and occurs in patients with poorly controlled diabetes or who are immunocompromised. The fungus has a predilection for the lumen of blood vessels, which are occluded by secondary thrombosis: the organism parasitizes the ophthalmic artery and its branches, leading to necrosis of the orbital tissues, the nose and the eye ( Fig. 9-8 ).

Some fungal infections are more prevalent in certain regions of the world: e.g. in the USA, where coccidioidomycosis, cryptococcosis, histoplasmosis and blastomycosis are all reported as ocular pathogens.
Protozoal and metazoal infections
(see Ch. 8 ) Several organisms from this group cause ocular pathology.
Toxoplasma gondii .
This is the commonest protozoal parasite to infect the eye. Congenital infection may occur when a woman becomes infected for the first time during pregnancy. Infection causes a classical tetrad of clinical features (meningoencephalitis, hydrocephalus, intracranial calcification and retinochoroiditis). Disease severity depends on in which trimester the infection occurs. Congenital ocular toxoplasmosis is a recurring and progressive disease because of the persistence of the parasite as bradyzoites within tissue cysts, which can reactivate. Foci of reactivation can be seen as an irregular area with associated vitreous haze at the border of a retinochoroidal scar.
Acquired infection is usually from cysts in undercooked meat or from drinking water containing oocysts from soil contaminated with cat faeces. Acquired infection is commonly asymptomatic, although some individuals will develop a ‘flu-like illness with lymphadenopathy. Retinochoroiditis may occur as a result of acute acquired infection, but in contrast with congenital infection there is no pre-existing scar. Toxoplasma retinochoroiditis is less common in immunocompromised patients than cytomegalovirus retinitis but can cause extensive retinal necrosis. Individual parasites (tachyzoites) and cysts (containing bradyzoites) can be identified in paraffin sections ( Fig. 9-9 ).

Acanthamoeba spp.
The incidence of acanthamoebal keratitis initially increased with greater use of soft contact lenses, but this form of keratitis it is now less common because of increased realization of the importance of hygiene in the handling of the contact lenses. Acanthamoeba is a free-living protozoan parasite and the main source of contamination is the fluid in the contact lens case and scales on taps. These loci often contain bacteria that provide nutrition for the protozoa. In the cornea, the acanthamoebae phagocytose remnants of dying keratocytes and polymorphs ( Fig. 9-10 ). The organism can be difficult to identify without the use of immunohistochemistry. Acanthamoebal keratitis is painful, but does not normally penetrate the cornea, remaining as a superficial keratitis; it responds only slowly to appropriate therapy.

Toxocara canis .
The adult worm of Toxocara canis , which can be several centimetres long, lives in the intestinal tract of the puppy and the eggs are passed out with the faeces. Infants may ingest the eggs, which release second-stage larvae in the stomach. The larvae of Toxocara can pass easily through the body tissues and the living organisms do not elicit an inflammatory response. The pathological consequences are usually related to death of the organisms, which heralds the onset of an immune response. The most sensitive organs appear to be the liver, lung, brain and eyes where both a delayed-type and immediate-type hypersensitivity response may occur. In the eye there are three possible outcomes:
- •
At the posterior pole, a slowly developing low-grade fibrous reaction within the retina can produce a tumour that resembles a retinoblastoma.
- •
In the mid-periphery, a more rapid active inflammatory reaction, which is characterized by the presence of numerous eosinophils, is followed by exudation into the retina and subretinal space, and then secondary retinal detachment.
- •
Inflammation and fibrosis occur in the vitreous base over the pars plana, and can induce a form of posterior uveitis, easily mistaken for pars planitis.
Other parasites
In equatorial climates, infection by helminths such as Wuchereria spp., Loa loa , Ascaris and several other nematode larvae causes retinal damage and inflammation when microfilariae migrate into the retina and vitreous. Remarkably, the level of inflammation can be relatively low grade and there develops a mottled pigmentary retinopathy (diffuse unilateral neuroretinitis, DUSN).
Inflammation may occur without infection
Many forms of intra- and extraocular inflammation occur without evidence of infection and in these cases autoimmune, allergic or otherwise termed ‘immune-meditated’ inflammatory mechanisms are envisaged.
Granulomatous inflammation
Macrophages accumulate often with multinucleate giant cells to form granulomas around a focus of tissue destruction. In addition to infectious diseases mentioned above, it is also a feature of a number of non-infectious diseases. Immunologically, these reactions often represent a delayed-type hypersensitivity response (DTH response; see Ch. 7 ) mediated by T-helper type 1/17 cells in which macrophages represent the effector cell. These granulomatous reactions include the following types.
Reactions to endogenous materials
Products of plasma, blood or cell breakdown can induce a giant cell granulomatous reaction when released into tissue. The commonest eyelid granuloma is the chalazion, which is a reaction to a blocked meibomian gland duct ( Fig. 9-11 ). Rupture of a cyst (either an epidermal inclusion cyst or a dermoid cyst) releases keratin, which is irritant and induces a chronic granulomatous reaction. Red cells and plasma in the extracellular matrix provoke an inflammatory reaction and the cellular response depends on the presence of fibrinolysins (plasmin and plasminogen activator) in the tissue fluids. In the anterior chamber, fibrin is diluted by the aqueous and is rapidly dissolved by fibrinolysins present in the aqueous. Thus, the cellular response to red cells is restricted to migration of macrophages from the iris vessels into the anterior chamber. A similar reaction occurs in the vitreous after bleeding from a tear in the retina or from torn preretinal vessels in vasoproliferative retinopathy. Similar haemogranulomas also occur within the orbit after trauma followed by bleeding (traumatic blood cysts).

Reactions to exogenous non-biological materials
Implantation in tissue of vegetable or organic matter such as wood excites a similar cellular response to that of sutures derived from cotton or synthetic materials. Synthetic fibres or fragments of plant are seen in polarized light as birefringent particles surrounded by macrophages and lymphocytes. Metallic fragments are slowly dissolved in tissue fluids, but elements such as iron are toxic to the retina, which undergoes neuronal loss, so that metallic foreign bodies in the vitreous or retina are especially dangerous. Brass contains copper and tin, and the reaction to copper ions is pyogenic for reasons that are as yet unknown. On occasion, similar reactions may be seen to materials used in ophthalmic surgery ( Box 9-9 ).
Plastic encircling bands made from silicone are used to indent the sclera in detachment surgery, in part because they produce little inflammatory reaction. However, there is always a surrounding fibrous capsule. In contrast a giant cell granulomatous reaction occurs around sutures. Particles of glass or the plastic used for intraocular lenses (polymethylmethacrylate; PMMA) do not stimulate a marked inflammatory reaction: the membranes on the posterior lens capsule are derived from metaplastic lens epithelial cells, not inflammatory cells. The silicone plastic plates and tubes (Molteno tubes and setons) that are designed to drain aqueous in advanced neovascular glaucoma do not excite an inflammatory response, although like the encircling bands the drainage orifices can be blocked by a fibrous capsule. Of the viscous fluids instilled to replace ocular fluids, hyaluronic acid (Healon®) is inert, while silicon oil employed in retinal detachment surgery stimulates a low-grade macrophage reaction after the oil becomes emulsified (see figure).

Non-granulomatous inflammation
Lymphocytes and plasma cells are found in a number of conditions in which the aetiology and pathogenesis are unknown. Many clinical forms of anterior and posterior uveitis, e.g. Behçet’s disease, are characterized by diffuse and intense lymphocyte and plasma cell infiltration as well as a prominent neutrophil infiltration. Lymphocytic perivasculitis is a feature of demyelinating disease (multiple sclerosis) in the optic nerve and of retinal vasculitis. In endocrine exophthalmos there may be focal clusters of lymphocytes (lymphorrhages) within the extraocular muscles (see p. 505 ).
Inflammation due to autoimmune disease
The basic mechanisms of autoimmune disease pathophysiology have been dealt with elsewhere (see Ch. 7 , p. 439 ). As indicated above, many inflammatory diseases of the eye and adnexae occur in the absence of identifiable causative organisms. Some are associated with generalized connective tissue disease or diseases with a recognized autoimmune aetiology. Others are restricted to the eye and periocular tissues. The following section describes some of these disorders.
Sjögren syndrome
Sjögren syndrome is a disorder of the lacrimal gland, acinar glands of the conjunctiva (glands of Wolfring and Krause, see Ch. 1 , p. 84 ) and salivary glands. The secretory acinar tissue of the lacrimal gland is destroyed by a lymphocytic infiltrate, with formation of lymphoepithelial lesions. Impaired secretion of saliva and tears leads to a dry mouth and dry eyes, respectively, and there is an associated loss of goblet cells in the conjunctival epithelium with squamous metaplasia of the surface epithelium. Patients with primary Sjögren syndrome have specific antibodies (anti-Rho, La).
Rheumatoid eye disease
Destruction of ocular tissue in rheumatoid arthritis is T cell-mediated but also includes an immune complex-mediated vasculitis. Impairment of blood flow to the anterior segment causes a necrotizing scleritis and peripheral corneal ulceration. Spontaneous central corneal ulceration (often without an inflammatory cell infiltration) occurs in rheumatoid disease (corneal melt), a phenomenon that is promoted by release of metalloproteinases. If the destruction of collagen in the sclera is severe, the tissue undergoes fibrinoid necrosis with a granulomatous reaction around the necrotic sclera. Thinning of the sclera leads to exposure of the underlying uveal tract (scleromalacia perforans) but ciliary body prolapse and perforation are uncommon. In some patients the inflammatory process is slower and is accompanied by a reactive fibrosis with massive thickening of the sclera (brawny scleritis). A localized inflammatory reaction at the posterior pole of the eye causes macular oedema and the mass may simulate a malignant melanoma (posterior nodular scleritis) with or without an exudative retinal detachment.
Other ocular surface disease
Autoimmune disease is seen in the skin of the eyelid in bullous diseases such as pemphigus and mucous membrane pemphigoid, which are extremely debilitating diseases and very difficult to treat. In the conjunctiva, autoimmune responses against basement membrane components and the attachments of the epithelium lead to severe stromal fibrosis. Immunofluorescence studies performed on tissue submitted in Michel’s transport medium show linear deposition of immunoglobulin G and sometimes C3 along the basement membrane ( Fig. 9-12 ). Detachment of epithelium from the basement membrane leads to inadequate protection and predisposes to secondary inflammation and the exudation of fibrin. In the fornix, a fibrinous exudate provides a scaffold for fibroblastic migration; the subsequent scarring process leads to adhesions between the eyelids and the globe (symblepharon).

Lens-induced uveitis
A cataractous lens contains proteins that are the breakdown products of the primary soluble crystallins and other proteins (see Ch. 5 ). Leakage of lens protein into the anterior chamber, either spontaneously or as a result of trauma, may induce a massive giant cell granulomatous reaction. Macrophages and lymphocytes enter the anterior chamber from dilated blood vessels in the iris and ciliary body, and may enter the lens cortex itself directly through a rupture in the lens capsule. There are commonly significant numbers of neutrophils and eosinophils in the inflammatory infiltrate. The inflammatory cells then pass directly through the epithelium of the pars plana during the associated cyclitis ( Fig. 9-13 ).

Leakage of lens protein into the anterior chamber does not automatically induce a prominent inflammatory response. Sometimes lens protein is relatively inert and induces an uncomplicated macrophage response. This may be associated with a rise in pressure caused by outflow obstruction by engorged macrophages (phakolytic glaucoma). If the rupture is acute, the outflow system is blocked by lens matter (lens particle glaucoma).
Sympathetic ophthalmia
A bilateral granulomatous inflammation of the choroid, ciliary body and iris (panuveitis) can occur after injury to one eye; the injury usually includes uveal incarceration within the sclera. Subsequent sensitization to previously sequestered ocular antigens leads to posterior uveitis mediated by major histocompatibility complex class II-restricted CD4 + T cells, which affect both the exciting eye and contralateral sympathizing eye. This immune response is directed against ocular self-antigens. The uveal tract becomes considerably thickened by an inflammatory infiltrate that includes collections of macrophages ( Fig. 9-14 ). The inflammatory process also involves the retinal pigment epithelium with the accumulation of macrophages (essentially small granulomas) at this site (Dalen–Fuchs nodules).

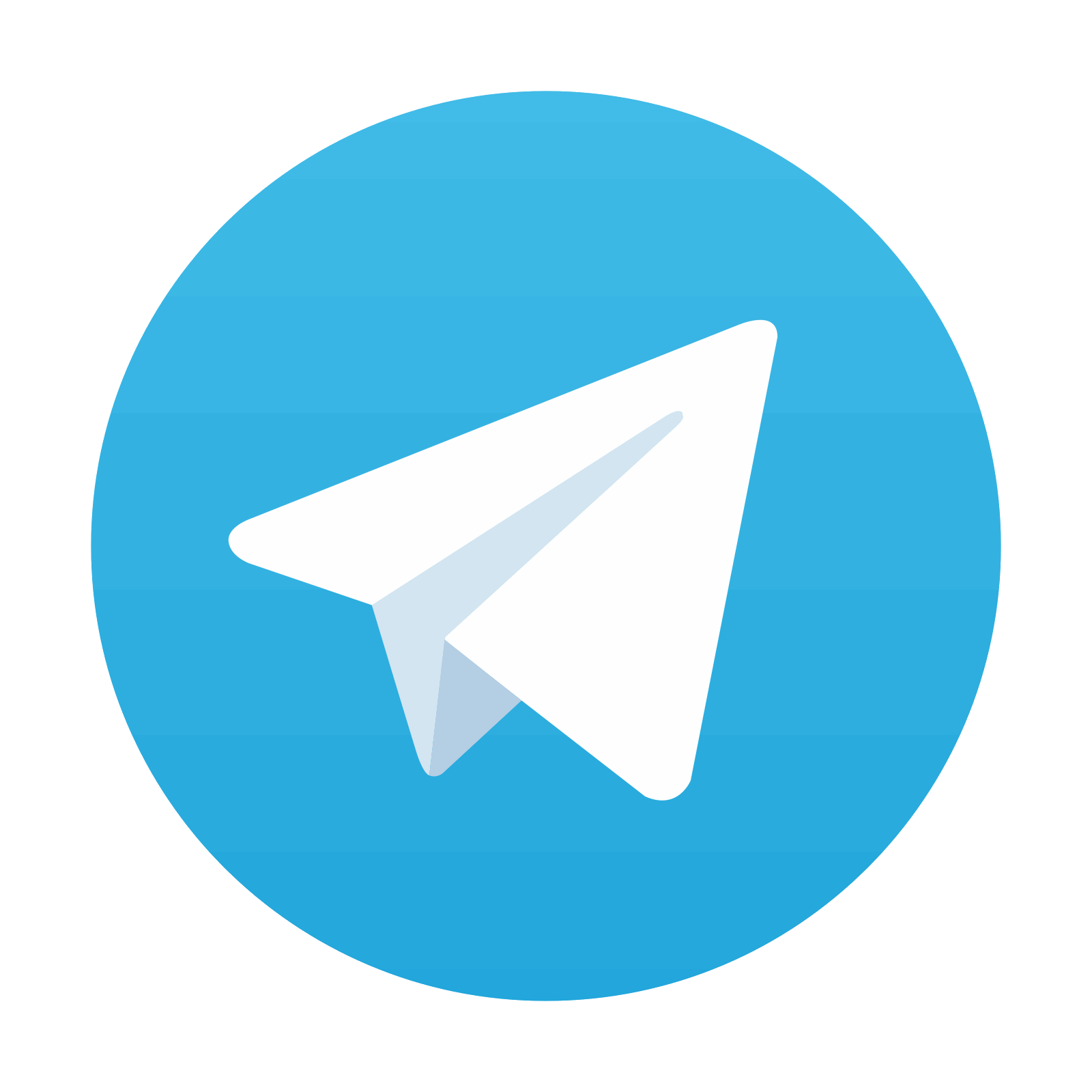
Stay updated, free articles. Join our Telegram channel

Full access? Get Clinical Tree
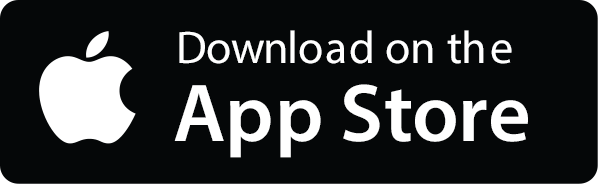
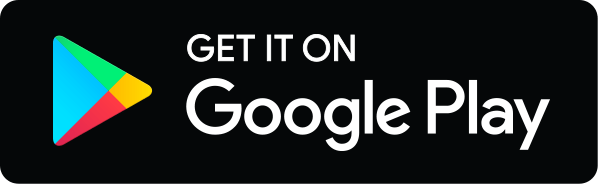
