Pathologic Correlates in Ophthalmoscopy
Julie M. Rosenthal
Alexander R. Gaudio
Vitaliano B. Bernardino Jr.
Edward A. Jaeger
Learning the pathologic correlates in ophthalmoscopy is an integral part of ophthalmic education. A sound base of knowledge of why lesions appear as they do can help us integrate clinical information and pathologic substrates and lead to a diagnosis, as well as a better understanding of the pathogenesis of the disease. However, before learning about abnormal findings, it’s important to review the normal appearance of the fundus.
THE NORMAL FUNDUS
Just as skin color varies between individuals, the color of the normal fundus varies from person to person. The color is related to the amount of pigmentation in the retinal pigment epithelium (RPE) and choroid, as well as the choroidal blood flow. For example, in those with albinism, the fundus appears bright orange because the pigment epithelium has little pigment and thus does not contribute much to fundus color. At the other end of the spectrum is congenital melanosis oculi, as in the patient with nevus of Ota. The fundus appears gray-orange because the normal choroidal melanocytes are so dense that they mask much of the orange color of the choroid. In focal areas of choroidal and pigment epithelium loss, the retinal tissue is transparent, and one sees the white sclera beneath. This is why colobomata appear white on ophthalmoscopy (Fig. 7-1).
![]() Figure 7-1. Fundus photograph of coloboma. The retinal pigment epithelium and choroid are focally absent. |
The macula contains xanthophyll pigment, which imparts a yellow color. With the exception of this yellow area, the normal neurosensory retina is transparent. The loss of retinal transparency, or the acquisition of color by the neurosensory retinal tissue, indicates a pathologic change. In focal areas where the retina is missing, the orange color of the underlying choroid is enhanced. This color difference is useful to keep in mind when searching for breaks on ophthalmoscopy (Fig. 7-2).
The normal internal limiting membrane (ILM) imparts the reflection from the surface of the retina seen on ophthalmoscopy. Müller cells secrete this true basement membrane. The absence of this “healthy sheen” may indicate pathologic changes at the level of the ILM such as a preretinal membrane (Fig. 7-3). The composition of the blood column and transparency of the blood vessel wall determine the color of the normal retinal blood vessels. States of poor oxygenation, such as pulmonary disease, extremely elevated lipid levels (i.e., lipemia retinalis) (Fig. 7-4), and opacification of the blood vessel wall (i.e., arteriosclerosis) (Figs. 7-5 and 7-6), alter the normal color of the blood vessels. The optic nerve head appears pink due to the vascular supply to the surface of the disc. This color is altered by loss of this vascularity or by replacement of the neural tissue by opaque glial tissue (Fig. 7-7).
![]() Figure 7-4. Cream-colored artery and vein in a patient with an extremely high blood lipid level (lipemia retinalis). |
![]() Figure 7-5. Section of retina demonstrating marked thickening of the arteriolar (A) wall as seen in arteriolosclerosis. Notice the compression of the adjacent venule (V). |
![]() Figure 7-6. Fundus photograph of opacified blood vessel (arteriolosclerosis) in the region of a previous branch retinal vein occlusion. Notice the neovascular tuft at the bifurcation. |
![]() Figure 7-7. Fundus photomicrograph of optic atrophy. The disc has lost its pink color due to loss of vascularity and replacement of neural tissue by opaque glial tissue. |
The different portions of the retina vary in makeup. The ganglion cell layer and nerve fiber layers are displaced centrifugally from the fovea in the macula. This anatomic variation causes lesions to appear differently depending on their location in the retina. For instance, on ophthalmoscopic evaluation, conditions that affect those layers are not seen in the fovea. Tay-Sachs disease, in which storage materials accumulate in ganglion cells, thus involves cells that are not present in the fovea. The storage material in the ganglion cells causes the normally transparent retinal tissue to opacify. The retina surrounding the fovea is affected by this opacification, but the fovea itself, which lacks the abnormal cells, still appears red due to the normal choroidal color—explaining the appearance of the foveal cherry-red spot. The opacification of the perifoveal retina, which appears white on ophthalmoscopy, is not seen beyond the macula. The extramacular retina contains a reduced concentration of ganglion cells, so is not affected (Fig. 7-8). The appearance of other lesions may be explained by the oblique orientation of the outer plexiform layer, wherein the rods and cones connect to the bipolar layer. The accumulation of fluid in the outer plexiform layer of the macula (the Henle layer), or the hydropic swelling of Müller cells causes cyst-like spaces to form.1,2 The cyst-like spaces are not lined by an epithelium and thus are not true cysts. They are oriented along the oblique pane of the Henle layer and appear to fan out from a central zone like the petals of a flower (Fig. 7-9). The fluorescein angiographic (FA) appearance of cystoid macular edema (CME) is frequently described as petaloid. Optical coherence tomography (OCT) studies of this fluid demonstrate cystic pockets of fluid in the macula (Fig. 7-10).1,2
![]() Figure 7-10. A. Fluorescein angiographic appearance of cystoid macular edema described as having a petaloid pattern. B. Spectral-Domain OCT showing cystlike spaces in the macula. |
The centrifugal displacement of the ganglion cell layer and nerve fiber layer in the fovea causes the oblique outer plexiform layer to lie superficially. Nerve fiber layer hemorrhages (flame-shaped hemorrhages) are thus not seen in the fovea. The ophthalmoscopic appearance of exudates and early retinal necrosis are similarly affected by these modifications of retinal cytoarchitecture.
COLOR CHANGES IN THE FUNDUS
The diseased fundus may change color to white, gray, yellow, black, or red. The area of involved retina affects the color and shape of hemorrhage, and the same disease may alter the color differently depending on its location. Fresh hemorrhage on the surface appears bright red, whereas fresh hemorrhage under the RPE appears much darker. Hemorrhage in the nerve fiber layer (Fig. 7-11A) dissects along the plane of the layer, parallel to the orientation of the ILM (Fig. 7-11B). Similarly, hemorrhage between the RPE and Bruch membrane also spreads in a plane parallel to the orientation of the membrane (Fig. 7-12). However, the adhesion of the RPE to the Bruch membrane limits the extent of the hemorrhage, whereas a nerve fiber layer hemorrhage has no such delineating structure. Fresh nerve fiber layer hemorrhage is bright red with feathery borders, whereas a subretinal pigment epithelial hemorrhage is brown-black with sharp borders (Fig. 7-13).
![]() Figure 7-12. Section of the eye showing subretinal hemorrhage. (Courtesy of Ralph C. Eagle, Jr., M.D., Philadelphia, PA.) |
The source and composition of emboli in the retinal vasculature may be identified by color. White emboli are composed of calcium and may originate from a calcified cardiac valve or calcified atheromatous plaque. Yellow emboli are often made of cholesterol and lipid, originating from noncalcified atheromatous plaques in the carotids (Hollenhorst plaque) (Fig. 7-14). Platelet emboli can be identified by their gray-white color. However, the appearance of emboli may not be a reliable indicator of their source and interobserver variability is high.3
White Changes in the Fundus
The collagen content of fibrous connective tissue imparts a white color (remember that the sclera is white). Retinal choroidal colobomata appear white due to the collagen content. On ophthalmoscopy, the temporal white crescent is so colored due to direct visualization of the sclera (Fig. 7-15). In high myopia the RPE and choroid are often stretched to such an extent that they do not reach the temporal margin of the disc. Therefore, the transparent retina is not present. The RPE contributes the black rim of the crescent seen near the disc in highly myopic eyes. Disciform scarring in macular degeneration appears white due to fibrous tissue proliferation from the choroid through breaks in the Bruch membrane into the subpigment epithelial or subretinal spaces.4 (Fig. 7-16). Vascularized membranes may appear white due to the contribution of fibrous tissue by fibroblasts in the adventitia of retinal vessels (Fig. 7-17A), such as those in proliferative diabetic retinopathy (Fig. 7-17B) or arterioles after vascular occlusion (Fig. 7-6). In arteriosclerosis, collagen accumulation in the vessel wall may cause it to thicken (Fig. 7-5) and impart a copper or silver color to the blood vessel (“copper/silver wiring”).
RPE injury often results in scar formation (Fig. 7-18). Pigment within this scar indicates collagen deposition from pigment epithelial metaplasia (Fig. 7-19). Injured nonpigmented epithelium can undergo similar fibrous metaplasia and contribute to membrane formation, such as cyclitic membranes in the ciliary body.
![]() Figure 7-18. Fundus photograph of pigmented scar secondary to retinal pigment epithelial hyperplasia induced by injury. |
Fibrous tissue may also grow into the fundus through a perforating wound. Scleral tissue does not heal by proliferation and bridging of a dehiscence. Rather, the episclera and choroid provide the necessary tissue for healing. This episcleral tissue may grow into the eye (fibrous ingrowth) and can be seen at the site of trauma, as well as in surgical scleral wounds, such as sclerotomy portals for pars plana vitrectomy (Fig. 7-20).
Tissues of the central nervous system use the process of gliosis for repair. Remember that the neurosensory retina’s embryologic origin is from an outpouching of the central nervous system. Therefore, the retina also achieves repair through gliosis. The Müller cell, likely responsible for this repair, may migrate through a break in the ILM and help to form a preretinal membrane. It may also enter the retro-retinal space to form membranes there. The tissue may be either transparent when thin or white when abundant. In nonglaucomatous optic atrophy, such as that seen after an infarct of the optic nerve (i.e., arteritic or nonarteritic optic neuropathy), the loss of tissue substance is accompanied by the proliferation of glial tissue. The gliosis, along with the decrease in the vascular supply to the nerve head, accounts for the whiteness of the disc (Fig. 7-7). This is also the pathologic explanation for the waxy pallor of the optic disc seen in retinitis pigmentosa, through decreased vascular supply to the nerve or vascular compression.5
Gliosis and fibrosis may also occur simultaneously. Preretinal membranes can be composed entirely of glial cells (Müller cell derivatives), or may involve the proliferation of both RPE cells and glial cells, especially in the presence of a retinal break. Breaks allow the pigment epithelial cells to gain access to the preretinal space. Often, cells with ultrastructural features of both fibroblasts and smooth muscle cells—myofibroblasts—can be found in preretinal and subretinal membranes.6 These cells are believed to originate from pigment epithelial cells. The myofibroblasts can secrete collagen and can contract, explaining the wrinkling of the internal limiting membrane seen in “macular pucker” and proliferative vitreoretinopathy (PVR). These cells have also been implicated in the contraction of iris neovascular membranes (ectropion uveae), cyclitic membranes, and the organization of intraocular hemorrhage.7
The white “snowbank” seen on the inferior pars plana and retinal periphery in eyes with chronic pars planitis is made up of organized inflammatory protein and cells, with contributions from glial cells and fibroblasts (likely derived from nonpigmented ciliary epithelium).8 Old vitreous hemorrhage, when organized, may appear white for similar reasons.
Myelin also appears white. The difference in appearance between white and gray matter in the central nervous system is due to myelin produced by oligodendroglia. Normally, myelinization of the optic nerve axons proceeds centrifugally from the nervous system and halts at the level of the lamina scleralis. However, sometimes the myelin may extend past the lamina scleralis into the retina. Recall that the axons of the optic nerve constitute the nerve fiber layer of the retina and that the cell bodies of these axons reside in the ganglion cell layer. Therefore, myelinated nerve fibers in the retina have a superficial appearance ophthalmoscopically due to their location. The myelinization does not halt abruptly, lending them the feathery edges seen on exam (Fig. 7-21).
![]() Figure 7-21. Myelination of the nerve fibers extending beyond the disc. Notice the feathery edges to the termination of the myelin. |
Cotton-wool spots, such as those seen in hypertensive retinopathy, represent focal retinal infarcts at the level of the nerve fiber layer. They may be the results of ischemia-induced transection of the axons at this layer. If axoplasmic transport continues to the point of interruption, the material transported will accumulate at the zone of transection in bulbous microscopic swellings, which, to early microscopists, resembled cells and were called “cytoid [cell-like] bodies” (Fig. 7-22). Some believe that cotton-wool spots represent axoplasmic debris in ganglion cell axons and are signs of underlying retinal pathology.9
Cotton-wool spots are seen mainly in the posterior pole of the retina (Fig. 7-23) The reason for this geographic restriction is not clear. Occlusion of the most superficial radially oriented peripapillary capillaries (confined in distribution to the posterior pole) has been implicated in the pathogenesis of cotton-wool spots.9 It is also possible that nerve fiber infarcts in the periphery are not visualized because there is insufficient inspissated axoplasmic material in this location.
![]() Figure 7-23. Multiple cotton-wool spots along with retinal hemorrhages in a superior temporal branch retinal vein occlusion. |
Necrotic retina also appears white. Necrosis may result from various inflammatory conditions, including viral, fungal, and protozoal (Toxoplasma) retinitis. Each type of retinitis appears, at least in part, as a white retinal lesion. Cytomegalovirus retinitis (Fig. 7-24) resembles a pizza pie with an admixture of white (retinal inflammation) and red (hemorrhage) (Fig. 7-25). The retinal abscesses of fungal retinitis are white. Likewise, the satellite lesion of retinochoroiditis caused by active Toxoplasma (the choroidal inflammation is merely in response to the primary retinal infection) is white (Fig. 7-26). The white appearance of a lesion from inactive Toxoplasma results from the destruction of neurosensory retina, retinal pigment epithelium, and choroid to permit a direct view of the sclera (Fig. 7-27). Necrosis in retinal-derived neoplasms is white; the appearance of regressed (necrotic) retinoblastoma often is described as “cottage cheese.”10
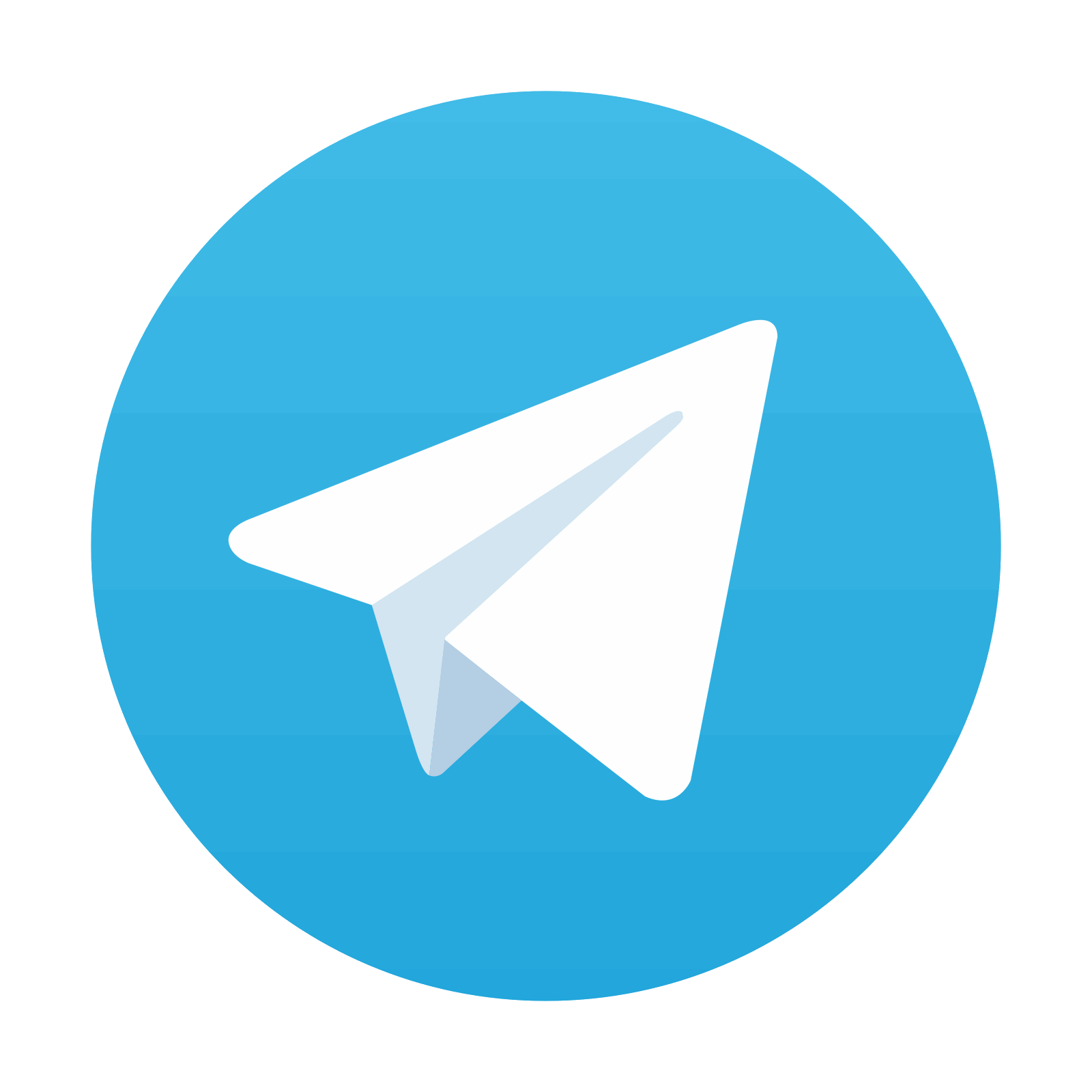
Stay updated, free articles. Join our Telegram channel

Full access? Get Clinical Tree
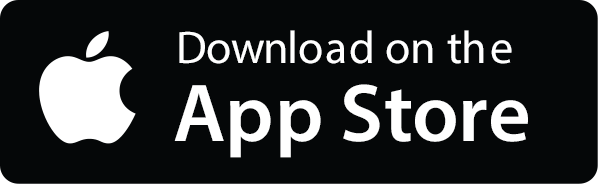
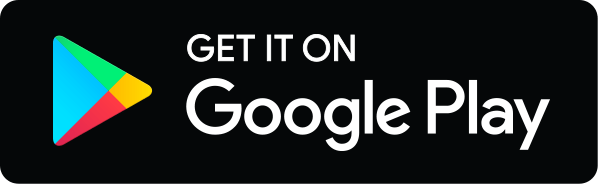