Pathogenesis of Cataracts
J. Samuel Zigler Jr.
Manuel B. Datiles III
Introduction
The primary functions of the ocular lens are to transmit incident light and to focus it on the retina. This requires that the lens be transparent, a condition dependent on the highly regular organization of the cells of the lens and the high degree of short-range order of the proteins in the lens cytoplasm.1 Protein concentration in lens fiber cells is extremely high, resulting in an index of refraction significantly greater than that of the surrounding fluids and enabling the lens to refract incident light. Cataract occurs when the lens loses its transparency by either scattering or absorbing light such that visual acuity is compromised. Cataracts can result from genetic, metabolic, nutritional, or environmental insults or can be secondary to other ocular or systemic conditions, such as diabetes or retinal degenerative diseases.2 By far the most important risk factor is age; aging-related cataract constitutes the great majority of all cataracts. This type of cataract is a major public health problem in the United States. In developing countries, where the availability of surgical facilities is limited, aging-related cataract is the leading cause of blindness. Because no efficacious nonsurgical therapy currently exists for cataract, the problem is expected to increase in magnitude as the world population becomes progressively older in coming decades.
To begin to understand the pathogenesis of cataract, it is necessary to recognize that it is not a single disease. Even aging-related cataracts vary greatly in the location of the opacity in the lens, the morphology and appearance of the opaque region, and the rate of progression of opacification. Because human aging-related cataract is caused by multiple interacting factors, elucidation of the underlying molecular mechanisms leading to opacification has been difficult. Most current knowledge about the pathogenesis of cataract has come from the study of live animal or in vitro models using animal lenses or lens cells in culture, because intact human cataract tissue has become very difficult to obtain with the advent of phacoemulsification procedures. Although numerous model systems are available and some have been investigated in detail, significant questions can be raised concerning how relevant they are to human age-related cataract. Inherent differences exist (discussed below) between the human lens and lenses from other species; in addition, experimental studies are usually conducted on relatively young animals or utilize lenses from young animals. In this chapter, the emphasis is on human aging-related cataract and what is known about the mechanisms underlying the disease process.
Aging-Related Cataracts: General Features
It would be a gross oversimplification to consider aging-related cataract as a single disease. There appear to be three major types of aging-related cataracts–cortical, nuclear, and posterior subcapsular–which differ in both the location in which the opacity initially appears and in the pathology underlying the opacification. Many risk factors may be common to all three types of aging-related cataracts and, although cataracts often begin as a pure type, as they mature they typically become mixed cataracts.
Cortical cataracts (Fig. 72.1A) occur in the outer region (about 25%) of the lens and are characterized by vacuoles, water clefts, and spokes. It is generally believed that most cortical cataracts are osmotic in nature–that is, water accumulates in, or between, the lens cells usually as a result of ionic imbalances. The electrolyte imbalances likely arise as a result of damage to the lens cell membranes, especially those of the lens epithelial cells, which play the major role in maintaining the ionic and metabolic homeostasis of the entire lens.3 Such damage could compromise the normal permeability characteristics of the membrane or damage specific membrane proteins responsible for ion transport. In cortical cataracts, potassium levels decrease, whereas sodium, chloride, and calcium increase, with the resulting imbalance leading to an influx of water.4 The vacuoles or “lakes” containing this water have a low refractive index relative to the protein-rich cytoplasm in the fibers, and the discontinuities create light scatter and cataract.
![]() Figure 72.1. A. Cortical cataract. Arrow points at a spoke opacity in midperiphery of lens. B. Nuclear cataract–slit-lamp photograph showing opacification of the nuclear area. |
Nuclear cataracts (Fig. 72.1B) occur in the central region of the lens and appear to involve an acceleration of processes that occur during aging even in the normal lens. The proteins accumulate postsynthetic modifications, especially resulting from oxidation, leading to formation of protein aggregates that scatter light.5 The proteins in the nucleus also become progressively more pigmented with age; in some nuclear cataracts the color can become dark brown or even black. In such cases, cataract may result from absorption of light rather than light scattering. In contrast to the cortical cataract, nuclear cataracts tend to become harder and less hydrated than normal, age-matched lens nuclei.
Posterior subcapsular cataracts occur at the posterior pole immediately beneath the lens capsule (Fig. 72.2). These cataracts may result from improper posterior suture formation or from abnormal differentiation of lens fibers.6 In the latter instance, lens epithelial cells may migrate to the posterior pole. Posterior subcapsular cataracts are formed after radiation with x-rays and long-term corticosteroid therapy; they occur secondary to retinal degeneration diseases; they also occur idiopathically.
The Genetic Factor
Although the significance of genetics in congenital cataract is very well established with some three dozen genes identified in which mutations can cause human cataract,7 the role of genetics in aging-related cataract is less well defined. Epidemiologic studies7,8 have demonstrated that a positive family history is a major risk factor for aging-related cataract. Studies on twins have concluded that genetics accounts for approximately 50% of both cortical and nuclear aging-related cataracts.9,10 Specific genes have not been identified, however, and it is likely that in most affected individuals the genetic component derives from multiple genes that affect the susceptibility of the lens to environmental insults and other cataractogenic factors. The genetic aspects of aging-related cataract have been discussed in depth in a recent review by Shiels and Hejtmancik7 and in Chapter 74 of this work11
Nuclear Cataract
Nuclear cataracts are the most common form of aging-related lens opacity; because of their central location in the lens, such opacities have a severe impact on vision. Nuclear cataracts are characterized by increased pigmentation, increased modification of lens proteins, and a general hardening or stiffening of the nuclear region of the lens (nuclear sclerosis). These changes tend to occur as the lens ages, even in the absence of nuclear opacification, but are exacerbated in nuclear cataracts. Thus, increasing age is the most important risk factor for nuclear cataract development, but a variety of other associations have been identified by epidemiologic studies.12,13 The most prominent molecular feature of nuclear cataract is oxidation, which is evident in the modifications occurring to the lens crystallins (and other lens proteins) and in the decreases seen in the critical cellular reducing compound glutathione.
Decades of work on the effects of oxidative stress on lens proteins have built on the seminal work of Antoinette Pirie,14,15 and Ruth van Heyningen16, and colleagues, 17 Working at the Nuffield Laboratory of Ophthalmology, Oxford University in the 1960s and 1970s, they demonstrated that exposure of lens protein solutions to sunlight caused the proteins to become heavily pigmented, to cross-link, and to aggregate.14,15,16,17 These are all features that are present in proteins from nuclear cataracts. The proteins of the lens nucleus are extremely long lived owing to the complete lack of protein synthesis in the bulk of the lens. Thus, there are proteins in the center of the adult lens that were synthesized prior to birth. They progressively accumulate a variety of post-translational modifications,18 many of which are associated with aging and not specifically, or preferentially, with cataractogenesis. However, the oxidation of the sulfur containing amino acids, cysteine and methionine, is characteristic of cataracts and not of aging normal lenses.19,20 Oxidation of cysteine side chains eliminates protein–sulfhydryl (SH) groups with the formation of protein–protein disulfide crosslinks or mixed disulfides between protein–SH groups and the low molecular weight thiols cysteine and glutathione.21 The maintenance of free–SH groups is essential to the functional integrity of the lens. The redox balance of lens cells is largely determined by the concentration of glutathione. Normally present at millimolar concentrations, even in the lens nucleus, glutathione has long been known to decrease markedly in human cataracts and in virtually all forms of experimental cataract.21 The biology of glutathione in aging-related nuclear cataracts will be further considered below.
Largely because of the work of three laboratories, it now appears that the fundamental processes involved in nuclear cataractogenesis are being established. Although each of the three investigators (Frank Giblin, Oakland University, Rochester, MI; Roger Truscott, Universities of Sydney and Wollongong, Australia; and David Beebe, Washington University, St. Louis, MO), have made distinct contributions and applied different approaches to the problem, their work, in aggregate, is highly consistent and complementary. Truscott’s interest in nuclear cataract dates back some 30 years to studies analyzing oxidation of proteins from such lenses. However, during the past decade he and his colleagues have made very important contributions to the understanding of the etiology of nuclear cataract through their work in several areas. One of these concerns the chemistry of the pigments present in the lenses of primates and certain other diurnal species. Human lenses from young individuals are yellow owing to the presence of low molecular weight kynurenine compounds derived from the aromatic amino acid tryptophan. The first such compound from the lens was identified in 1971 by van Heyningen.16 With increasing age, the nuclear region of the lens becomes progressively darker in color and the pigmented compounds become protein bound. Previous studies had demonstrated that the pigmented proteins from cataractous lenses possess photosensitizing capability and could generate the reactive oxygen species singlet oxygen and superoxide anion when exposed to near ultraviolet (UV) radiation at wavelengths capable of penetrating into the lens.22,23 In a series of publications, the Truscott laboratory has identified a number of kynurenines and derivatives that are present in the human lens, they have described the pathways whereby these compounds are produced, and they have shown that these UV filters absorb ultraviolet radiation that otherwise could have detrimental photochemical effects on the lens and retina.12 Although these compounds are beneficial in the free form, it was also confirmed that when bound to lens proteins, as occurs with increasing age in the lens nucleus, these UV filters become stronger photosensitizers which can, in the presence of oxygen and UV radiation, generate singlet oxygen, superoxide anion, and hydrogen peroxide within the lens nucleus24 Hydrogen peroxide, although producing little direct damage, can be converted into highly reactive and damaging species in the presence of transition metals.25,26 Both iron and copper have been reported to be elevated in concentration in nuclear cataracts.12 It appears highly likely that the hydroxyl radical, or similar species, produced endogenously within the lens is a major factor in nuclear cataract development.
To maintain homeostasis and functional integrity in the face of the chronic oxidative stress to which it is exposed,27 the lens must balance the stress with endogenous antioxidant defenses. Central to this defense is the tripeptide glutathione. The laboratory of Frank Giblin has been instrumental in establishing the significance of the glutathione redox cycle in protecting the lens from oxidative damage (Fig. 72.3).28 There is a gradient of reduced glutathione within the lens, with levels being much higher in the metabolically more active peripheral regions of the lens where the molecule is synthesized and where it can be reduced following oxidation. Although the lens nucleus undoubtedly requires less glutathione than the cortex, which is exposed to oxidative stress from external sources as well as that produced endogenously, adequate glutathione must be present in the lens nucleus to balance the oxidative stresses. A critical factor in determining how much glutathione is in the lens nucleus may be the so-called lens barrier, which has been described by the Truscott laboratory.29 Although the physical basis for the barrier and its physiologic function in the normal lens remain unclear, the location of the barrier at or near the nucleus–cortex boundary strongly suggests that it plays a major role in the distinctive structural and functional properties of these two regions of the lens.30 Because the barrier has been shown to impede the movement of glutathione, UV filters, and other small molecules, its potential impact on nuclear cataract formation is great.
![]() Figure 72.3. Glutathione redox cycle and its relation to the hexose monophosphate (HMP) shunt and the detoxification of H2O2 by glutathione peroxidase. |
Essential to the oxidative stress mechanisms cited above is the availability of oxygen. It has long been recognized that the lens exists in a reduced oxygen environment, which is a critical factor in controlling the oxidative stress to which it is exposed throughout life.13 Using novel technology, the oxygen concentration has been determined at various points around and within the lens.31 These studies have demonstrated a steep decreasing gradient of oxygen from the lens periphery to the center. The utilization of available oxygen by mitochondria, which are present only at the periphery, appears to be responsible for maintaining the gradient and the very low oxygen level in the nucleus. The importance of keeping nuclear oxygen extremely low and the significance of oxidation in nuclear cataract development are strongly supported by two clinical situations, hyperbaric oxygen therapy and vitrectomy surgery both of which produce a high incidence of nuclear cataract.
The association of hyperbaric oxygen treatment with subsequent nuclear cataract formation was first reported by Palmquist et al.32 Giblin et al.33 and Gosselin et al.34 have followed up this observation by conducting extensive studies on the effects of hyperbaric oxygen exposure to animals that have demonstrated decreases in glutathione and protein sulfhydryls, increased formation of protein mixed disulfides, increased nuclear light scatter, as well as structurally altered lens cell membranes. These changes are all characteristic of human aging-related cataract. Further, aggregation of crystallins has been clearly demonstrated in vivo by dynamic light scattering in the lens nucleus of guinea pigs after multiple hyperbaric oxygen treatments.35 Beebe13 has investigated the effects of vitrectomy surgery on the oxygen concentrations around and within the lens. It has been recognized for some time that patients 50 years of age or older who undergo vitrectomy have a 60% chance of developing nuclear cataract within 2 years.13 Surgical removal of the vitreous body in patients was shown to produce a spike in oxygen tension within the eye during the procedure; further, oxygen tension remained at higher than normal levels for many months after the surgery.36 It was postulated that the vitreous gel normally impedes diffusion of oxygen from the region adjacent to the retina where oxygen is high owing to proximity to retinal vessels. This keeps oxygen tension low in the anterior vitreous chamber near the posterior aspect of the lens. When the vitreous gel is removed surgically, it is replaced by fluid, which does not provide a comparable level of inhibition of diffusion. An analogous, although less extreme, situation exists in many older individuals who have not had vitrectomy surgery. The vitreous body gradually degenerates with age, leading to liquefaction (i.e., the conversion of vitreous gel into liquid). This is a highly variable condition, but if the hypothesis is correct, one would predict that individuals with more liquefaction would be at greater risk for nuclear cataract. In an analysis of donor eyes, Harocopos et al.37 found that eyes with increased vitreous liquefaction had increased nuclear opacification. No increase was found for other types of cataract in these eyes, which is consistent with the idea that the nucleus is at particular risk from increased exposure to oxygen.
In addition to the limited antioxidant defenses present in the nuclear region of the lens, another factor that could contribute to the susceptibility of this area of the lens to cataract may be the loss of α-crystallin,38 a major structural protein of the lens. α-Crystallin inhibits the aggregation of damaged proteins (and thereby the formation of light-scattering centers) through its chaperonelike activity.39 A recent study on human subjects, indicates that loss of α-crystallin potentiates the formation of nuclear cataract and that the amount of α-crystallin remaining in the lens nucleus can be monitored in vivo by dynamic light scattering.40 This novel technology may have clinical value as a predictor of future cataract development.
Thus, compelling data from different laboratories, derived from a variety of experimental approaches, model systems, and clinical findings indicate that aging-related nuclear cataract is an oxidative disease and that the level of molecular oxygen to which the lens is exposed is a critical determinant in its etiology.
Cortical Cataracts
<txt>Age-related cortical cataracts are less common than nuclear cataracts in whites, but are more common than nuclear cataracts in Asians and Africans.41,42,43 Epidemiologic studies have linked cortical cataracts to UV damage from sunlight,44 aging, and metabolic disorders, such as diabetes, diarrhea, and dehydration.45 The importance of genetic predisposition in age-related cataract is becoming established.7,8,9,10,11,46,47,48,49 Recent twin studies have led to estimates that genetic heritability of cortical (as well as nuclear, but not posterior subcapsular cataract) is approximately 50%.9,10 This may help explain why cortical cataracts are more common in African-Americans compared with Caucasians who are exposed to the same risk factors.42,43 These clinical genetics studies also calculated that individual environmental factors contributed 26% to 37% and age 11% to 16% toward the causation of cortical cataracts.
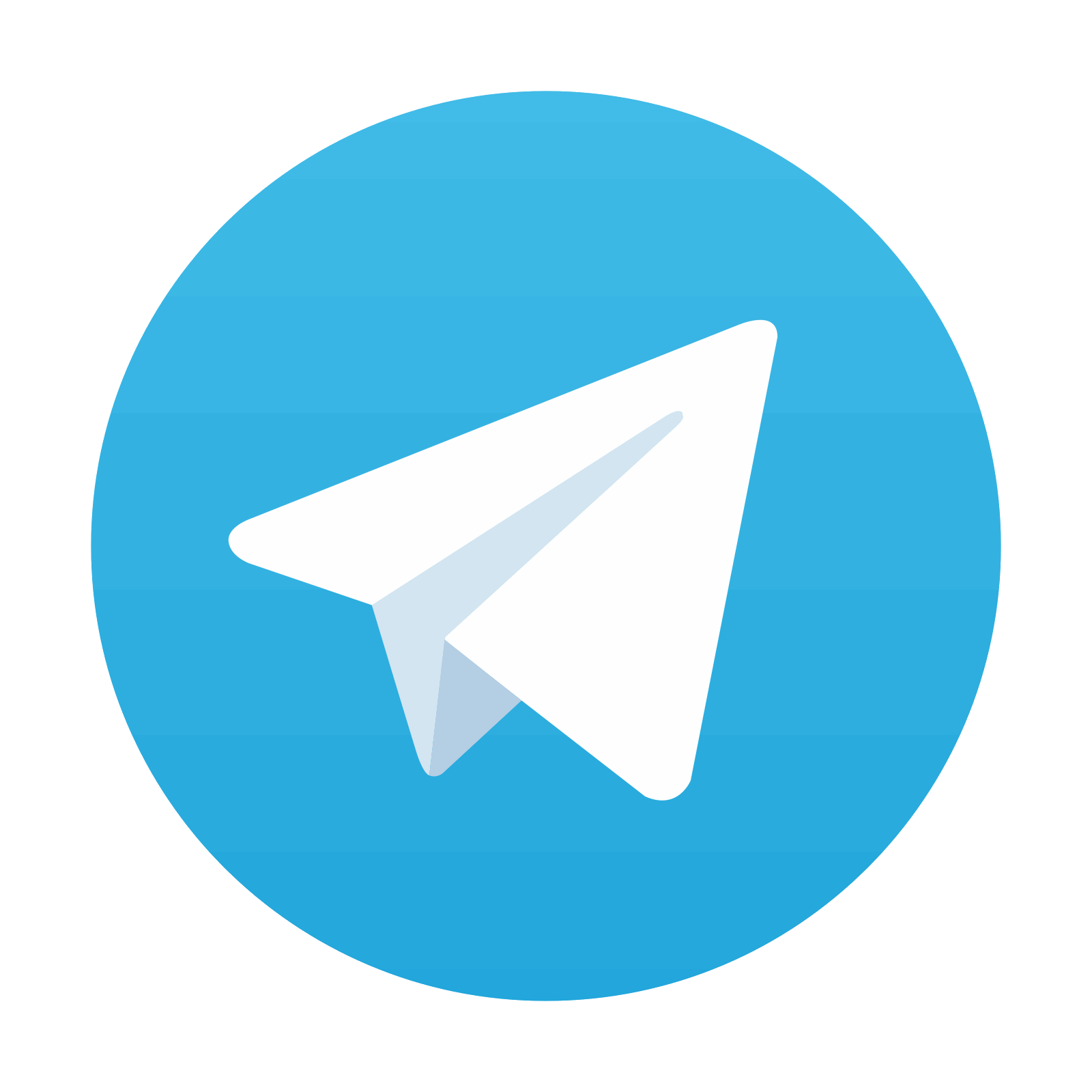
Stay updated, free articles. Join our Telegram channel

Full access? Get Clinical Tree
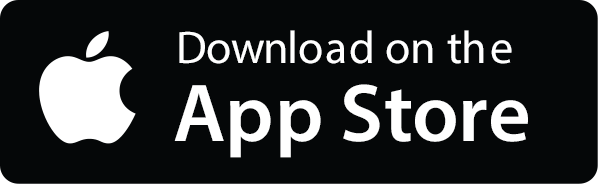
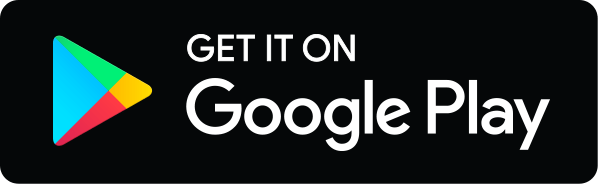