2 Michael A. Lemp As Chapter 1 documents, dry eye is a widespread problem affecting hundreds of millions of people throughout the world.1 In the United States alone, between 30 and 50 million people are thought to experience some degree of dry eye symptoms, and more than 9 million have moderate to severe dry eye disease.2,3 Although the extent of the prevalence of dry eye has been recognized only recently, clinicians have long known that dry eye symptoms are common, a fact further corroborated by surveys reporting about a 30% incidence of dry eye symptoms as a presenting complaint in patients seeking ophthalmological attention. Several pathogenetic mechanisms of dry eye have been proposed during a period spanning 40 years. In the 1960s it was generally thought that dry eye was caused by a decrease in the secretion of the lacrimal glands, resulting in desiccation of the ocular surface (although that term was not to be used until the late 1970s). Several studies reported a significant decrease in tear volume in patients with dry eye compared with normal subjects. Newer methods such as fluorescein dilution were used to examine tear secretion rates, which were also decreased. Changes observed on the corneal and conjunctival surfaces were surmised to be the result of desiccation. By the end of the 1960s, attention was being drawn to another phenomenon—instability of the tear film in patients with symptoms but normal tear secretion as measured by Schirmer’s 1 and 2 tests. The concept of the “mucin-deficient dry eye” was introduced.4,5 In these patients there was evidence of extensive inflammation of the conjunctiva with a loss of the mucin-producing goblet cells of the conjunctiva. Conditions associated with this form of dry eye included hypovitaminosis A, erythema multiforme (Stevens-Johnson syndrome), ocular pemphigoid, and extensive trachoma. The hallmark of the mucin-deficient dry eye was very rapid breakup of the tear film. In the late 1970s, the concept of the “ocular surface” as not only a contiguous structure comprising the cornea, conjunctiva, and lids but as an integrated functional unit was introduced. Newer methods allowing for the identification of intracellular keratin profiles of epithelial cells led to the knowledge that corneal and conjunctival epithelial cells could be distinguished; in addition, their degree of terminal differentiation could be determined.6 The mechanisms and pathways by which the outer epithelial surface of the eye is renewed were explicated with location of stem cells in the pericorneal limbus; the centripetal movement of increasingly differentiated corneal cells toward the central cornea and surface gave rise to the “x,y,z hypothesis” in which the production of new cells with centripetal migration toward the corneal center and surface was balanced by the exfoliative loss of aging cells from the surface.7 Shortly thereafter, attention was drawn to the role of the meibomian glands of the eyelids in the pathogenesis of evaporative tear loss. During the 1980s and 1990s, a series of publications detailed the role of the lipid layer (derived from the meibomian gland secretions) of the tear film in retarding loss of aqueous tears in normal subjects.8–10 In patients with obstructive meibomian gland dysfunction (MGD), the rate of evaporative tear loss increased from the normal 40% to almost 100%. Analyses of lipid profiles of human meibum have shown specific alterations in patients with MGD.11 Subsequent studies have documented that MGD is present in approximately two thirds of patients presenting with dry eye and that this is true in the most severe form of dry eye (i.e., Sjögren’s syndrome) and in a mixed dry eye population presenting with complaints of ocular irritation.12,13 MGD then assumes an even more prominent place in dry eye disease than lacrimal gland hyposecretion (see later). For many years, clinicians have recognized that dry eye is more common in women, particularly in those in the perimenopausal or postmenopausal age range. It has been assumed that this is related to a decline in circulating estrogen levels characteristic of menopause. More recently, considerable literature has called this assumption into question and has implicated androgen insufficiency as a more likely culprit.14 These conclusions are based on animal models that clearly show the effects of androgen deprivation on lacrimal and meibomian gland secretion and the effects of androgens in suppressing inflammation in these glands. Human studies have shown a high prevalence of MGD in patients with a rare genetic disease called complete androgen insensitivity syndrome, as well as in patients being treated with antiandrogen drugs. These studies have led to attempts to treat dry eye, and in particular MGD, with topically applied androgens; late-stage clinical studies are under way. In the past two decades, considerable literature detailing features of inflammation in dry eye has been published.15 These studies have described animal models of dry eye in which inflammation is a prominent feature resulting in tissue destruction. An increase in proinflammatory cytokines in the tears of animal models of dry eye and in human subjects with dry eye has been documented. Histopathological evidence of increased infiltration of inflammatory cells in the lacrimal glands, ocular surface, and meibomian glands of patients with dry eye has led to the conclusion that inflammation is a critical feature of the dry eye disease process and results in clinically significant tissue destruction. This is thought to be true not only in Sjögren’s-associated dry eye but also in non-Sjögren’s dry eye. What is not entirely clear is where inflammation enters into the sequence of events leading to ocular surface damage in dry eye. It is thought, for example, that in dry eye associated with systemic immune-mediated disease, inflammatory infiltrates are first seen in the subepithelial mucosal tissue of the conjunctiva. In other forms of dry eye, inflammatory infiltration of the glandular and epithelial structures might be the initial presentation of inflammation. The role of other factors, such as increased osmolarity of the tear film in the causation of inflammation, has been described. The global role of inflammation in the pathogenesis of dry eye is increasingly accepted and has formed the basis for new treatment strategies. In 1998 Stern et al proposed that the ocular surface and the associated glandular structures (i.e., lacrimal and meibomian) form a functional unit linked by a neural system.16 In this concept, the sensory nerves of the ocular surface regulate aqueous tear production by the lacrimal glands. Stimuli from the external environment (mechanical, thermal, or chemical) activate sensory receptors on the cornea and conjunctiva; these impulses form the afferent arc of a feedback system. After reaching the central nervous system, this activity results in an efferent arc to the epithelia and glands of the ocular surface, stimulating secretion of glandular and epithelial components of tears. These tear substances (cytokines, growth factors, and hormones) act to protect the ocular surface and regulate cellular regeneration. This concept has recently been enlarged to include the epithelia of the nasolacrimal ducts. Not only the lacrimal and meibomian glands but also the mucin-producing cells of the conjunctiva are stimulated to secrete in this system. Thus, the sensory receptors of the cornea, conjunctiva, and outflow tracts act as sentinels monitoring the environment of the ocular surface and responding to perturbations by altering signals to modify secretory activity, which in turn attempts to maintain the normal homeostatic environment and respond to injury. Certain factors, such as inflammation, downregulate the sensory receptors, limiting the ability of the ocular surface to respond to disease. Hyperosmolarity of the tear film is recognized as a global feature of dry eye states.17 It is associated with several other changes seen in dry eye, such as inflammation, tear instability, increased evaporation, interactions between components of tears, and desiccation of the ocular surface. In 1941, von Bahr18 first proposed that the tear film of dry eye patients was characterized by increased tear osmolarity, but Mastman et al were the first to measure these differences in 1961.19 Subsequently, a considerable literature has emerged documenting a shift to a hyperosmolar tear film in dry eye subjects.20 Progress in these studies has been limited by the difficulties involved in the methodologies employed to measure tear osmolarity. The osmolarity characteristics of tear film are largely due to the salt concentration of tears; tear proteins play a relatively small role. The most widely used osmometry instruments have employed two surrogates for tear salt concentration (or osmolarity), that is, freezing point depression or vapor pressure measurement. Both of these technologies are better suited to measuring larger volumes of biological fluids such as obtained from serum, urine, or blood. Each technology involves sample sizes frequently unobtainable from dry eye patients and requires transfer of samples to test chambers and a degree of technical expertise in test performance and end point judgment not available in most clinical settings. For these reasons, as well as for time and money constraints, tear osmometry has been restricted to the research setting. With these caveats, however, several research centers have documented that increased tear osmolarity is a feature of all types of dry eye, including aqueous tear deficiency and evaporative dry eye due to MGD and contact lens wear. A discussion of the mechanisms by which elevated tear osmolarity is thought to produce ocular surface damage follows in the next section. In recent years, attention has been directed to another global feature of dry eye disease—instability of the tear film.17 Tear film instability is a more rapid than normal disruption of the preocular tear film. Normally, the tear film covering the cornea and conjunctiva is continuous between blinks. If blinking is prevented, the tear film will eventually develop dry spots or areas of discontinuity. The length of time between the last complete blink and the appearance of the first randomly occurring dry spot is defined as the breakup time (BUT). This interval is usually measured by staining the tear film with sodium fluorescein dye and observing the interval with the aid of a cobalt blue filter. These values are normally in excess of 10 seconds. An alternative way of measuring stability is the so-called noninvasive BUT in which a grid image is projected onto the cornea and the first appearance of the first disruption of the image is timed. The latter method is associated with longer BUTs.
Pathogenesis and Classification of Dry Eye Disease
Key Points
♦ Changing Concepts of the Pathogenesis of Dry Eye Disease
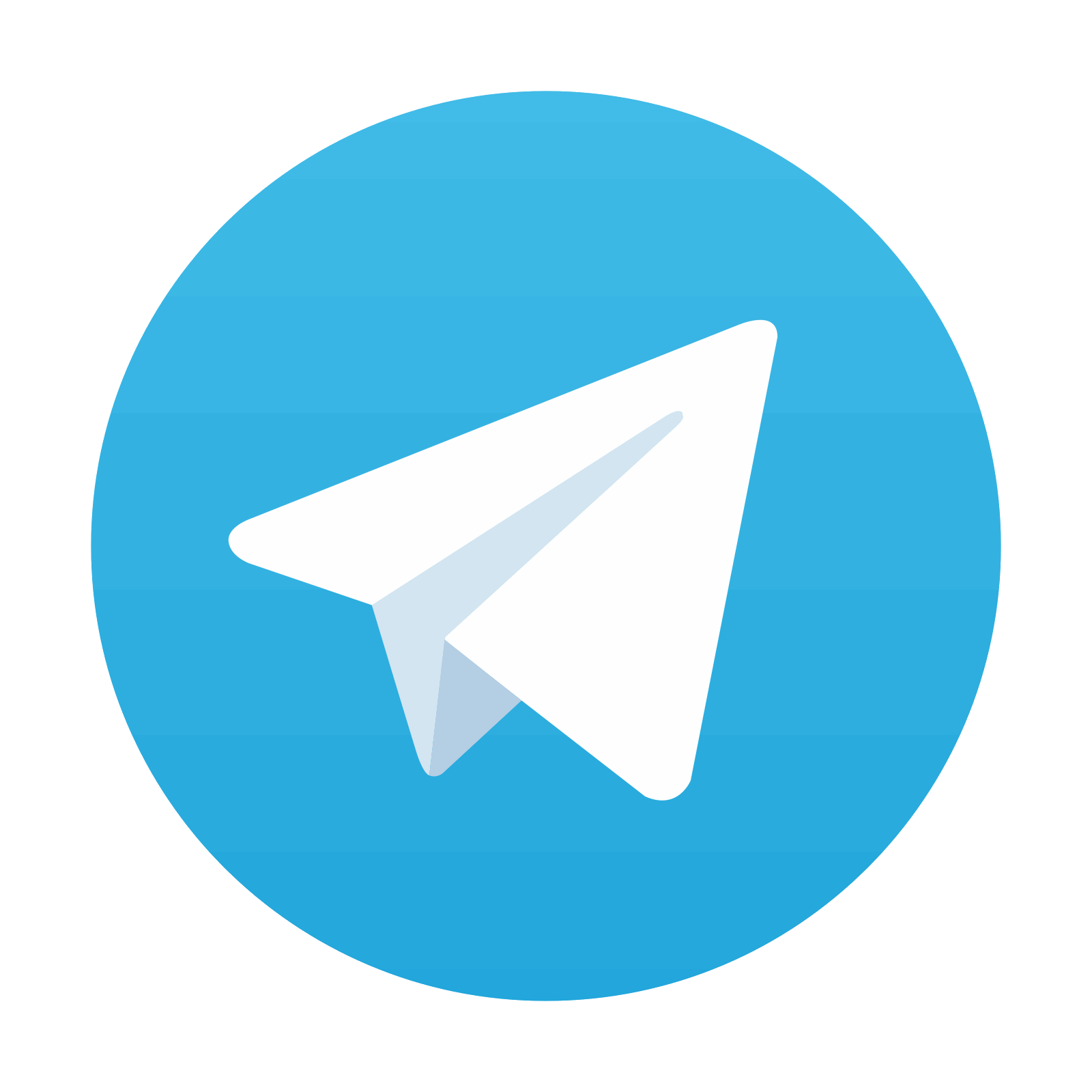
Stay updated, free articles. Join our Telegram channel

Full access? Get Clinical Tree
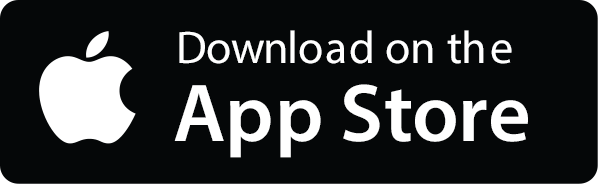
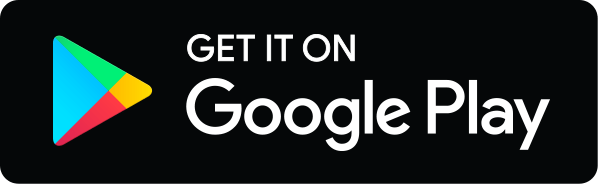