Orbital Fractures
Grant D. Gilliland
Dale R. Meyer
Fractures of the orbit are seen in a significant number of patients who have blunt trauma to the face and skull. The prominent position of the orbit within the craniofacial skeleton predisposes this region to injury. Orbital fractures may be limited to the anatomic boundaries of the orbit itself or may be associated with more extensive fractures of the craniofacial skeleton, including the contiguous nasoethmoid, zygoma, maxilla, and frontal (skull) bones. Orbital fractures are of obvious ophthalmologic importance as the bony orbit houses the eye and soft tissues that support the globe, and these structures may also suffer direct or indirect injury. Potential ophthalmic injuries include rupture of the globe (sclera or corneal perforation); iridodialysis; angle recession; hyphema; lens subluxation or dislocation; choroidal, retinal, or vitreous hemorrhage or detachment; and choroidal rupture. Malpositions of the globe (e.g., enophthalmos, hypo-ophthalmos), orbital hemorrhage, direct or indirect traumatic optic neuropathy, extraocular muscle injury, eyelid or canthal malposition, and damage to the lacrimal gland or lacrimal drainage apparatus may also be seen.
The main long-term sequelae of orbital fractures are ophthalmic functional impairment and aesthetic deformity. Thus, it is important that ophthalmologists be familiar with the evaluation and management of blunt orbital trauma and orbital fractures.1, 2 and 3 In cases of more extensive injury to the craniofacial skeleton, the ophthalmologist will likely contribute to the care of the patient as part of a multidisciplinary medical team, including specialists in the area of otolaryngology, oral and maxillofacial surgery, plastic surgery, and neurosurgery.
The past 15 to 20 years have seen remarkable advances in the evaluation and treatment of orbital and craniofacial trauma, largely as a result of the improved ability to diagnose the extent of craniofacial injury using neuroimaging modalities.1, 2, 3, 4, 5 and 6 This period has also witnessed the increased use of rigid internal fixation of craniofacial fractures, including many of those that involve the orbit.6, 7, 8, 9 and 10 This chapter will detail relevant epidemiology, applied orbital anatomy, classification, biophysics of orbital fractures, clinical and radiologic evaluation of orbital fractures, treatment of orbital fractures, future developments in orbital fractures, and associated orbital fracture complications.
EPIDEMIOLOGY
The epidemiology of orbital fractures is similar to that of facial fractures in that men are three times more likely to sustain ocular and orbital trauma than women.11, 12 Common mechanisms of injury include motor vehicle collision, aggravated assault, baseball and softball injuries, tennis and racquetball injuries, hockey puck and stick injuries, elbow and finger injuries, and a multitude of other causes of blunt trauma to the face. Because of the developing bony structures in pediatric patients, orbital floor fractures are uncommon in children younger than 8 years of age.13 Age-related incidence of orbital trauma is typically bimodal, with a peak incidence in late adolescence and again in patients older than 75.12 The incidence of orbital fractures in patients with head trauma is approximately 20%. In asymptomatic patients with a history of head trauma the incidence is much lower at approximately 4%.14
Associations with other fractures, systemic injuries, and ocular injuries are an important consideration when evaluating the patient with an orbital fracture. Cervical spine injuries can be associated with orbital fractures with an incidence of 5% to 8%. The incidence increases when more than one facial fracture is present.15 The prevalence of head injury associated with facial fractures is approximately 65% to 88%. Approximately 50% of patients with orbital floor fractures sustain other facial fractures, and 30% have an associated eye injury.16 The associated eye injury can be serious in approximately 18%, with a 2% to 3% incidence of visual loss associated with orbital fractures.17, 18 and 19 Diplopia is a common symptom in patients with orbital trauma and is present in approximately 50% of adults and the majority of children with orbital fractures. Similarly, extraocular motility limitation is more commonly noted in children than adults with orbital floor fractures. One specific type of fracture pattern is clearly more prevalent in children than adults—the “trapdoor fracture.”20
APPLIED ANATOMY
The orbits are each formed by seven bones of the face and skull: frontal, sphenoid, zygomatic, maxilla, ethmoid, lacrimal, and palatine (Fig. 48.1). The bony orbit and soft tissue structures act to support, protect, and maximize the function of the eyes. Each bony orbit can be considered a four-sided pyramid, open at its base (orbital rims), with the posterior apex at the area of the lesser wing of the sphenoid, which contains the optic foramen. At the orbital apex, the optic nerve exits the orbit through the optic foramen. The depth of the orbit anteroposteriorly is approximately 40 to 50 mm from the inferior orbital margin to the orbital apex. The four sides of the pyramid are the walls of the orbit, divided into inferior (floor), medial, superior (roof), and lateral. The orbital rims that define the anterior orbital aperture are relatively strong bone. The posterior portion of the orbit, composed predominantly of the sphenoid bone, is also relatively strong. In contrast to the relatively thick strong bone at the orbital rims and apex, the middle portion of the orbit is composed of relatively thin bone. This is particularly true of the floor and medial wall of the orbit.1, 21
The orbital roof is an extension of the supraorbital rim and can be considered part of the skull. The roof is composed predominantly of frontal bone separating the orbital contents from the anterior cranial fossa and the frontal sinus. The medial wall of the orbit is formed by the thin orbital plate of the ethmoid bone (lamina papyracea), which separates the orbit from the ethmoid sinus. The lacrimal sac fossa, composed of the lacrimal bone and frontal process of the maxilla, is located in the anterior aspect of the medial orbit and contains the lacrimal sac drainage apparatus. The nasolacrimal duct continues inferiorly through the medial portion of the maxilla via the nasolacrimal canal. Inferiorly, the medial portion of the floor is composed of the orbital aspect of the maxilla and overlies the maxillary sinus. The infraorbital groove runs through the orbital aspect of the maxillary bone, extending anteriorly from the inferior orbital fissure. Fractures of the orbit most commonly involve this portion of the orbital floor medial to the infraorbital groove. The more lateral aspect of the orbital floor is composed of somewhat thicker bone of the zygoma. The superior tip of the palatine bone also contributes to a small portion of the orbital floor posteriorly. The lateral wall of the orbit is composed of the zygoma (anteriorly) and the greater wing of the sphenoid (posteriorly), separating the orbit from the temporal fossa and middle cranial fossa, respectively. The superior orbital fissure lies between the roof and the lateral wall of the orbit posteriorly and is formed by a gap between the greater and lesser wings of the sphenoid. The majority of the motor and sensory nerves to the eye and ocular adnexa pass through the superior orbital fissure. The zygomatic bone establishes not only the shape and contour of the inferior lateral orbital rim, but also the malar eminence (cheekbone). The zygoma has several important points of articulation with the orbit, including the zygomaticofrontal suture (superolateral orbital rim), zygomaticomaxillary suture (inferior orbital rim), and the zygomatico-sphenoidal suture, a broad posterior attachment with the sphenoid bone. The zygomatic arch also articulates with the temporal bone at the zygomaticotemporal suture.
![]() FIG. 48.1 Bony anatomy of the orbit. The orbital rims and orbital apex are composed of relatively thick bone, whereas the middle portion of the orbit is composed of relatively thin bone. |
The bony confines of the orbit determine the potential orbital volume, which is filled by the eye and associated soft tissues. The bony orbit provides a framework for support of the ocular adnexal soft tissues, which include fibroadipose tissue (orbital fat), extraocular muscles, blood vessels, and the cranial nerves serving the eye (II-VI). The orbital fibroadipose tissue contains an elaborate connectivetissue “ligament” system, as described by Koornneef.22, 23 Fibrous tissues extend from the periosteum lining the orbit (periorbita) to the bulbar fascia (Tenon capsule) and fascial sheaths of the extraocular muscles and their check ligaments. This diffuse connective tissue framework supports orbital structures and maximizes the function of the eye, particularly with regard to ocular motility. Restriction of ocular motility may thus occur if this connectivetissue system is compromised significantly by trauma, including entrapment within a fracture site.
The bony orbit also provides important support and attachments for the eyelids. The orbital septum extends from a condensation of periosteum at the orbital rim (arcus marginalis) to help form the intermediate connective tissue lamellae of the eyelids.24 The eyelids are further supported medially and laterally by canthal tendons, which have complex, firm attachments to the bony orbital rim.25, 26 The anatomy of the medial canthal tendon is intricately associated with the lacrimal drainage apparatus and attaches via an anterior crus to the anterior lacrimal crest and a posterior crus to the posterior lacrimal crest. The posterior attachment is particularly important for maintaining adequate eyelid-globe apposition.26 The lateral canthal tendon attaches to the zygoma just inside the lateral orbital rim at the lateral tubercle.25 Displacement of the orbital rims may thus cause distortion of the eyelids and palpebral aperture. This is particularly true in injuries to the nasoethmoid-orbital complex, which can produce telecanthus (widening of the intercanthal distance), as well as in zygomatico-orbital fractures, which can produce displacement (dystopia) of the lateral canthus.
CLASSIFICATION
Most classification schemes separate orbital fractures according to the relative involvement of the walls of the orbit (internal) and the orbital rims (external). The term complex is used to describe orbital fractures with more extensive disruption of the orbital rims and internal walls, usually associated with other fractures of the craniofacial skeleton. Fractures of the walls of the orbit typically involve the floor and medial walls and result in outward displacement of these bones. Smith and Regan27 coined the term blow-out fracture in 1957 to describe these types of internal orbital fractures, and this term has gained widespread popular usage. Blow-out fractures may occur in isolation or with involvement of the orbital rims. The terms pure and indirect have been used to describe blow-out fractures isolated to the internal orbit alone; the terms impure and direct to describe blow-out fractures associated with rim involvement. Although internal orbital fractures generally result in outward displacement (blowout fracture), inward displacement of one or more walls of the orbit (blow-in fracture) can occur, but much less commonly.28, 29 Blow-in fractures may involve any of the internal orbital walls, but most typically occur at the orbital roof. Blow-out fractures tend to result in a net increase in intraorbital volume with the potential for enophthalmos, whereas blow-in fractures result in a relative reduction in orbital volume and the potential for exophthalmos.
Additional terms common to fracture nomenclature elsewhere in the body may also be used to describe orbital fractures. These terms include closed if the fracture is not associated with a violation of the integument (i.e., intact soft tissue and skin) versus open (or compound) if the fracture communicates with an overlying skin laceration. Displacement is used to describe the degree to which a segment of bone is spatially dislocated from its normal anatomic position. Comminution is used to describe the degree of fragmentation of the fracture. Displacement and comminution may be graded from nonexistent (nondisplaced, noncomminuted) or mild to severe. Occasionally a circular segment of the orbital wall may fracture and become displaced, but remain attached on one side, producing a trapdoor fracture.
The degree of bony orbital disruption is frequently related to the amount of energy producing the injury. Manson has proposed a classification of facial fractures based on anatomic location and the pattern of comminution and displacement related to energy6:
Low-energy orbital fractures. These demonstrate simple linear or circular blow-out fractures (or, less commonly, blow-in fractures) of one or two walls, typically the floor or medial wall (with no involvement of the orbital rim).
Middle-energy orbital fractures. These typically involve at least two orbital walls, usually accompanied by fracture of the orbital rim. Usually these fractures do not involve the most posterior portion of the orbit.
High-energy orbital fractures. These include extreme disruption of multiple segments of the orbital rim and orbital walls. Frequently these injuries are circumferential, with three or four walls of the internal orbit destroyed.
Orbital fractures can be classified further by the pattern of involvement with the contiguous facial skeleton. Fractures confined to the anatomic boundaries of the orbit per se are termed isolated fractures. Orbital fractures with significant involvement of the adjacent areas are named accordingly. Zygomatic fractures (also known as trimalar or tripod fractures) typically involve disruption of the zygoma at the zygomaticofrontal and zygomaticomaxillary sutures at the orbital rims and along the zygomatic arch and have variable orbital floor involvement. Many authors refer to such fractures as zygomatico-orbital (or similarly orbitozygomatic) fractures.30 Fractures of the nasoethmoid complex may frequently involve the orbit; these are referred to as nasoethmoid-orbital fractures. Le Fort fractures also deserve special mention. These complex fractures are divided into three categories (Fig. 48.2):
1. Le Fort I. Low, transverse maxillary fractures that do not involve the orbit (in contrast to Le Fort II and III fractures, which may cause complex disruption of the orbit)
2. Le Fort II. Fractures involve the nasoethmoid and maxillary bones with disruption of the inferior and medial orbital walls
3. Le Fort III. Fractures extend from the nasoethmoid region across the orbit, involving the orbital floor and the medial and lateral walls
BIOPHYSICS OF ORBITAL FRACTURES
The location and severity of orbital fractures are determined by the location, direction, and magnitude of forces acting on the craniofacial skeleton. The bony orbital/craniofacial skeleton is known to dissipate incident forces in characteristic ways that determine the resultant fracture patterns. The eye and soft tissues of the orbit may also be compressed by blunt trauma, increasing the hydrostatic (hydraulic) pressure within the orbit. Historically, two theories have been presented as to why fracture of the orbital wall or walls may occur without concomitant fracture of the orbital rim27, 31, 32 and 33:
1. Hydraulic mechanism theory. Increased intraorbital hydraulic pressure is primarily responsible for orbital fracture.
2. Bone conduction (buckling) theory. Bone conduction through the orbital rim directly to the orbital floor can result in isolated fracture of the orbital floor.
Isolated orbital blow-out fractures tend to occur when the orbit is struck at low velocity by objects that have a slightly greater diameter than that of the orbital aperture (e.g., baseballs, fists), the orbital floor being the location most typically involved. The relatively thin bone of the orbital floor tends to become displaced into the maxillary sinus due to the lack of resistance of the underlying airfilled space and to some extent the effects of gravity.
Smith and Regan’s27 classic “hurling ball experiment” offered support for the hydraulic mechanism theory. Using a cadaver, they produced isolated internal orbital blow-out fractures by striking a hurling ball placed over the orbit when the soft tissues were intact. When the soft tissues were removed (by exenteration) and the opposite orbit was struck with a similar force, no orbital fractures were produced. When the striking force was sufficiently increased, the orbital floor and orbital rim collapsed simultaneously.
Small, blunt objects of a diameter less than that of the bony orbit (e.g., golf balls, racquetballs) are generally believed to have a greater likelihood of rupturing the globe while leaving the orbital walls intact. Using an experimental in vivo primate model, however, Green and colleagues32 demonstrated that relatively low energy forces (as low as 2.08 J) applied to the eye alone can also produce orbital blow-out fractures, offering further support for the hydraulic mechanism theory. However, in a cadaveric model Warwar demonstrated that floor fractures may occur at energies of 78 mJ.34 Rhee studies35, 36 have determined cadaveric fractures of the orbital floor occurring at 2.9 and 1.22 J respectively, using the hydraulic mechanism to create the fracture. Gilliland et al.,37 in a large in vivo caprine study, measured not only total fracture energy for the creation of orbital floor fractures (using the hydraulic mechanism), but deconvoluted the energy dissipative mechanisms with intraocular pressure measurements, intraorbital pressure measurements, and highspeed videography with software analysis. This study was in approximate agreement with Green’s and Rhee’s study for the total energy required to produce an orbital floor fracture (approximately 2,350 mJ). Most previous studies were in cadaveric specimens, which may suffer from desiccation of bone, which can affect bone density. Clinically, this energy approximates that of a 30-mph baseball. Energy dissipative mechanisms including eye deformation and eye displacement were shown to account for approximately 10% of the energy dissipation, with the vast majority of energy dissipation being fracture creation.37 Interestingly, temperature was measured as a potential energy-dissipative mechanism and was found not to be a significant factor mitigating against frictional energy dissipation as a cause of orbital fat atrophy in posttraumatic enophthalmos.37 Clinical and laboratory observations verify the preponderance of orbital floor fractures with elevated hydraulic pressures in the orbit. Takizawa examined the shape of the orbital walls through finite element analysis and found that the arched shape of the orbital roof compared to the flat profile of the orbital floor may be responsible for the resistance of orbital roof to elevated hydraulic pressure.38
Direct compression of the orbital rim alone has been shown by others to produce a transient buckling deformation of the walls of the orbit sufficient to produce isolated internal fractures without fracturing the orbital rim, supporting the bone conduction (buckling) theory.33 Indeed high-speed intramaxillary sinus videography has shown elastic buckling without fracture of the orbital floor when a force is applied to the orbital rim alone. At sufficient force the orbital floor fractured without fracturing of the orbital rim.37 Proponents of the bone conduction theory also suggest that this mechanism better explains the etiology of less commonly seen blow-in fractures, particularly those involving the orbital roof.
In reviewing these and other reports on the mechanisms of orbital fractures, it appears clear that the hydraulic mechanism and bone conduction theories are not necessarily mutually exclusive explanations of orbital blow-out fracture pathology. Indeed, Waterhouse demonstrated that both mechanisms can cause orbital floor fractures, although with different fracture characteristics.39 It is also clear that higher-energy forces applied to the orbital skeleton are more likely to produce fractures of the orbital rims as well as the internal orbital walls. Higherenergy injuries to the orbital and midfacial skeletal region are dissipated in characteristic fashion through a system of buttresses, which contribute the principal structural supports of the facial skeleton. Motor vehicle accidents, falls, sports/play accidents, and assaults comprise the most typical events producing orbital fractures.
The pattern of orbital fractures also appears to be affected by growth patterns of the craniofacial skeleton.40 The increased craniofacial ratio and lack of frontal sinus pneumatization of younger children, particularly those younger than 7 years of age, may place the cranium at increased risk for injury relative to the proportionally smaller midface and may in part explain the higher proportion of orbital roof fractures in younger children.29, 40, 41 Continued development of the maxilla, with “unfolding” of the face from beneath the overhanging cranium with increasing age, subsequently exposes the face to increased risk of trauma. Intrinsic to this process is the remodeling of the elastic cancellous bone of the infant’s face into the adult form of more compact, dense bony buttresses connected by thin and brittle membranous bony plates. Thus, the changing anatomy of the cranial and facial structures surrounding the orbit can contribute to a developmentally specific dissipation of impacting energy and a corresponding pattern of orbital and craniofacial fractures.40
Orbital soft tissue injury and deformation also occurs with orbital trauma and (aside from injury to the globe) is responsible for the main sequelae of orbital blow-out fractures: enophthalmos and diplopia.1, 2 and 3, 42, 43, 44 and 45 Enophthalmos may be caused by multiple mechanisms, the most prominent of which is enlargement of the bony orbit (loss of bony support), particularly when more than 50% of the orbital floor is involved. Disruption of the orbital fibroadipose connective tissue system (musculofibrous ligament system) contributes to formation of posttraumatic enophthalmos due to displacement and change in the shape of orbital soft tissue.42, 43 and 44 The loss of bone and ligament support permits posterior displacement and reshaping of orbital soft tissues under the influence of gravity and the remodeling forces of fibrous scar contracture. It is clear that these soft tissue changes are not insignificant and that enophthalmos can worsen within a period of months in some patients.4, 44 Fat atrophy is believed to be a less frequent mechanism of enophthalmos. Temperature elevation has not been shown to be a factor in energy dissipation during impact of a caprine orbit with a steel rod. Therefore, frictional energy dissipation within orbital fat is an unlikely cause of fat atrophy.46 The soft tissue changes following orbital blow-out fracture are predominantly believed to be responsible for the relatively poor results obtained with late reconstruction versus early reconstruction.
Diplopia due to incarceration of the extraocular muscles or surrounding fibroadipose connective tissue may also occur with orbital blow-out fractures. Additionally, direct injury to the muscle or its nerve supply within the orbit can also occur as a result of contusion and ischemia.43, 45, 47, 48
Another clinically important question concerns the strength of an orbital fracture repair at various time intervals after surgery. A common question arises—especially with athletes—as to when they can return to their preinjury activities. There are certainly a multitude of factors that play a role in releasing a patient to return to normal activities, such as associated injuries, status of the eye, presence of hyphema or vitreous hemorrhage, iritis, optic neuropathy, traumatic brain injury, cerebrospinal fluid leak, or other facial, head, or neck fractures. Regarding strength of fracture repair at various time intervals after repair, Gilliland’s study looked at strength of fracture repair at 1, 3, and 6 weeks after injury. In addition, three different implant materials were examined at each of the three time periods. He found that in all instances the strength of the fracture repair was less than the pre-injury strength at 1 week. However, at 3 weeks after fracture repair the strength exceeded pre-injury floor strength46 (Fig. 48.3).
EVALUATION
Clinical Examination
Evaluation of the patient with orbital trauma should proceed in a logical, defined manner.1, 2 and 3 Initial evaluation includes a determination of the extent of associated systemic and ocular (globe) injuries. Life-threatening injuries take highest priority, and initial management to ensure an adequate airway, ventilation, and circulation is essential. More extensive craniofacial injuries may be associated with neurologic damage of the brain or spine. In most cases, the initial patient assessment will have been completed by emergency room personnel and other specialists. If a high index of suspicion for globe injury is present, then the globe should be protected by a shield or similar device. A complete history should be obtained, including the mechanism of injury as well as whether there was any loss of consciousness or other sensory complaints such as decreased vision or diplopia. Assessment of the orbital injury should include as complete an eye examination as possible to assess the presence of rupture of the globe, traumatic optic neuropathy, or an evolving orbital compartment syndrome (i.e., dramatically increased intraorbital pressure secondary to orbital hemorrhage or edema). These conditions require emergent treatment. Assessment of visual function in the emergency setting includes pupillary reaction, visual acuity, and confrontation visual fields. Ideally, slit-lamp examination and dilated fundus examination are performed to assess the degree of injury to the globe. In many cases this may not be feasible because of the condition of the patient, forcing this portion of the ophthalmic examination to be deferred. In particular, pharmacologic pupil dilation (for examination of the fundus) must not be done if the patient has an unstable neurologic status requiring monitoring of pupil reaction. The presence of an afferent pupillary defect (Marcus Gunn pupil) is highly suggestive of traumatic optic neuropathy. If further clinical examination supports the diagnosis of traumatic optic neuropathy and no other contraindications exist, some would advocate treatment with megadoses of corticosteroids, although this has not been shown to be more effective than observation alone.49 In two specific instances of traumatic optic neuropathy, surgery may be indicated. Optic nerve sheath fenestration may be indicated if an intradural sheath hematoma is the cause of the traumatic optic neuropathy. Optic canal decompression may rarely be indicated if bony fragments from the sphenoid wall are clearly impinging on the optic nerve within the bony optic canal. Further orbital evaluation includes an assessment of ocular motility, globe position, eyelid and canthal position, orbital rim palpation, and facial sensation and movement. The face is also inspected and palpated for possible associated facial and nasal deformities. Malocclusion or difficulty opening the jaw may suggest the presence of zygomatic fracture or other more complex facial fracture. Cerebrospinal fluid rhinorrhea may also be noted in cases of injury to the nasoethmoid complex.
Ocular motility examination includes assessment of ocular alignment in primary position and eccentric gaze positions. Any limitation of ocular ductions is suggestive of entrapment (restriction) of the extraocular muscles or associated connective tissue. It is important to remember, however, that diplopia may be caused by any number of ocular motor abnormalities that may be present in the acute trauma setting, such as decompensation of a previous phoria or damage at any level of the central nervous system and peripheral motor unit. Within the orbit, damage to the soft tissues may produce orbital hemorrhage (subperiosteal hematoma or frank intraorbital hemorrhage) and edema. Rarely extraocular muscle laceration/avulsion may also occur.50 Orbital edema is invariably associated with injury to the orbital soft tissues; when severe, the edema may cause mechanical limitation of ocular movement. Blunt orbital trauma may also cause direct injury to the extraocular muscle or its immediate nerve supply, producing extraocular muscle weakness (paresis).
Some degree of head trauma may also accompany orbital injuries, and the potential for higher-level disruption of ocular motility, such as cranial neuropathies or damage to cerebral or brainstem areas serving ocular motor function, should also be considered. The ocular motor examination should therefore be correlated with other clinical and radiologic findings. Forced duction and force-generation testing are certainly helpful in distinguishing ocular muscle restriction from paresis, with the caveat that in the early period after injury, orbital edema or hemorrhage may produce a mild to moderately positive forced duction test. Thus, forced duction testing is most useful when orbital edema has largely subsided. Saccadic velocities can also be helpful in differentiating restrictive from paretic etiologies,48 but such testing is frequently not feasible in the acute setting, and in most cases this determination can be made on the basis of clinical and radiologic findings alone. Patients with limitation of ocular motility subjectively experience binocular diplopia, provided vision is relatively intact in both eyes. In addition to objective measures of ocular motility, the severity (i.e., subjective complaint) of diplopia can be quantitated by determining the range or area of single binocular vision, measured in degrees. Quantization of the field of single binocular vision can formally be done with a device such as a Goldmann perimeter, which is helpful for calculating the degree of visual impairment or disability. Clinically significant diplopia is usually considered to be diplopia within the central 30° of fixation. Diplopia in downgaze is considered to be more poorly tolerated. Of course, occupational and recreational considerations will determine what is significant for an individual patient. When an involved eye without muscle entrapment or strong mechanical restrictions moves more than 10° or 20° on each Hess chart, natural recovery can be expected.51
The position of the globe is also assessed (Fig. 48.4). As detailed previously, outward expansion of the orbital walls (blow-out fractures) can produce enophthalmos. Inward expansion of the orbital walls (blow-in fractures) can reduce orbital volume, potentially producing exophthalmos. In the early period after blunt orbital trauma, orbital edema and hemorrhage can transiently increase the orbital soft tissue volume, producing exophthalmos or “masking” the underlying potential for enophthalmos. As orbital edema subsides, the true globe position becomes evident. Over a more extended period of time (several months), soft tissue changes (cicatrization and, less commonly, fat atrophy) may contribute to the production of late enophthalmos. Indeed displacement of intraconal fat with disruption of ligamentous support has been shown to cause enophthalmos. The shape of the retrobulbar orbital contents changes from a modified cone to a sphere, and the globe sinks backward and downward.42 Globe position can be grossly assessed by direct visual inspection. Viewing the axial projection of the globes from above (“bird’s-eye view”) or from below (“worm’s-eye view”) facilitates a gross determination of axial globe position.
Quantitative exophthalmometry is performed for formal measurement of the degree of axial globe malposition. A difference of >2 mm is considered abnormal. Typically a difference ≥3 mm is required to produce an obvious clinical difference between the two eyes, although pre-existing lid and facial morphology (e.g., prominent dermatochalasis or eyebrows) may make the difference clinically less obvious and minimize disfigurement. It is important to note that standard Hertel exophthalmometry may not be accurate if the lateral orbital rim is disrupted. In such cases, the Naugle exophthalmometer may be useful in that it measures the position of the globe relative to the maxilla and frontal bone. Early (<4 weeks after injury) exophthalmometry has been shown to correlate poorly with predictions of enophthalmos, whereas late exophthalmometry readings (>4 weeks) were more predictive of late enophthalmos.52 CT imaging with volumetric analysis can detect 1-mL volume changes in the bony orbit, which correlate with 1 mm of enophthalmos. Therefore, a 2-mL change in orbital volume detected on CT could be expected to be clinically significant with 2 mm of enophthalmos as measured by exophthalmometry.53 The globe may also be displaced in a nonaxial direction, generally toward the area of least resistance. Hypo-ophthalmos (i.e., downward displacement of the globe) associated with disruption of the orbital floor is the most common nonaxial type of displacement. Globe dystopia can be assessed by the relationship of the globe to the surrounding anatomic landmarks and the contralateral eye (if not involved by the injury). Typically with hypo-ophthalmos, the globe sinks downward and the inferior corneal limbus may drop below the lower eyelid margin, producing a “setting sun” appearance. Globe dystopia can be measured with a ruler, noting the distance and direction of globe displacement in millimeters. In extreme cases of orbital floor disruption, the globe may actually subluxate into the maxillary sinus.54
![]() FIG. 48.4 A patient with blunt left orbital trauma demonstrates moderate enophthalmos of left eye. The patient had marked limitation of supraduction. |
Eyelid and canthal appearance may also be affected in numerous ways by blunt orbital trauma. Almost invariably, eyelid edema and ecchymosis is noted, in some cases sufficient to shut the eye completely, making examination difficult. Mechanical ptosis may also occur secondary to edema. Direct or indirect injury to the levator muscle or aponeurosis may also occur after blunt trauma. Eyelid lacerations are also common, varying from superficial to complex, depending on the nature of the injury. All eyelid lacerations should be examined and irrigated with saline, particularly if the wounds are contaminated. In some cases, the lacerations may facilitate approach for repair of orbital or other facial fractures. When eyelid lacerations involve the eyelid margin medial to the lacrimal puncta, injury to the canaliculus should be suspected and evaluated further by diagnostic lacrimal (canalicular) probing or irrigation. Displacement of the medial canthus may occur with soft tissue injury (laceration) or with fractures of the medial nasoethmoid-orbital region, which may result in telecanthus (widening of the distance between the medial canthi). Coexistent damage to the canaliculi, lacrimal sac, or other portions of the lacrimal system may be associated with such injuries. In rare cases of extreme orbital or craniofacial injury, traumatic hypertelorism (increase in the intraorbital distance) may occur as a result of disruption and lateral dislocation of the entire bony orbit or orbits. Lateral canthal displacement may also occur after soft tissue injury or fractures involving the lateral orbital rim. Most typically, inferior lateral canthal dystopia occurs with zygomatico-orbital (i.e., tripod or trimalar) fractures when the fracture segment is displaced and rotated inferiorly.
Examination should also include palpation of the bony orbital rims and periocular soft tissues. Crepitus (a crackling sound) on palpation is a sign of air trapped in the soft tissues (emphysema). Orbital and subcutaneous eyelid emphysema is most typically associated with fractures of the medial orbital walls, although communication with any of the perinasal sinuses may potentially allow air to dissect into the soft tissues. Usually the emphysema produces minimal complications, and the patient is simply instructed not to increase nasopharyngeal pressure (i.e., avoidance of nose blowing, sneezing, Valsalva maneuver). Occasionally the accumulation of air within the orbit can be sufficient to produce some mass effects that can potentially cause central retinal artery occlusion or compressive optic neuropathy and severe visual loss.55 Extreme vision-threatening orbital emphysema may necessitate decompression by direct aspiration of the air pockets.
Palpation of the globe and orbital soft tissues directly can also be used to estimate the degree of resistance to retropulsion as a rough gauge of the intraorbital pressure or tension. This should be performed only if a ruptured globe has been ruled out. Intraorbital pressure can be increased as a result not only of air accumulation, but more typically of edema or hemorrhage, which may be sufficient to produce an orbital compartment syndrome. When the globe or optic nerve is compromised by increased orbital pressure, emergency measures are required to reduce orbital pressure, including lateral canthotomy/cantholysis and other adjunctive medical and surgical treatment.2 As alluded to earlier (in the “Classification” section), inward displacement of portions of the orbital wall may also produce compression of vital ocular structures. Although inward displacement of the orbital roof is probably the most common location, inward displacement of the relatively thicker lateral wall of the orbit (zygoma) may potentially create the most serious damage to the globe and orbital soft tissues. In some cases, bony disruption and associated edema may be sufficient to produce optic nerve compression or superior orbital fissure syndrome, manifested by ipsilateral ptosis of the upper eyelid, proptosis, ophthalmoplegia, hypoesthesia of the ophthalmic branch of the trigeminal nerve, and dilation/fixation of the pupil. In such cases, prompt reduction of inwardly displaced orbital fractures is indicated. Adjunctive high-dose corticosteroids can also be considered.56
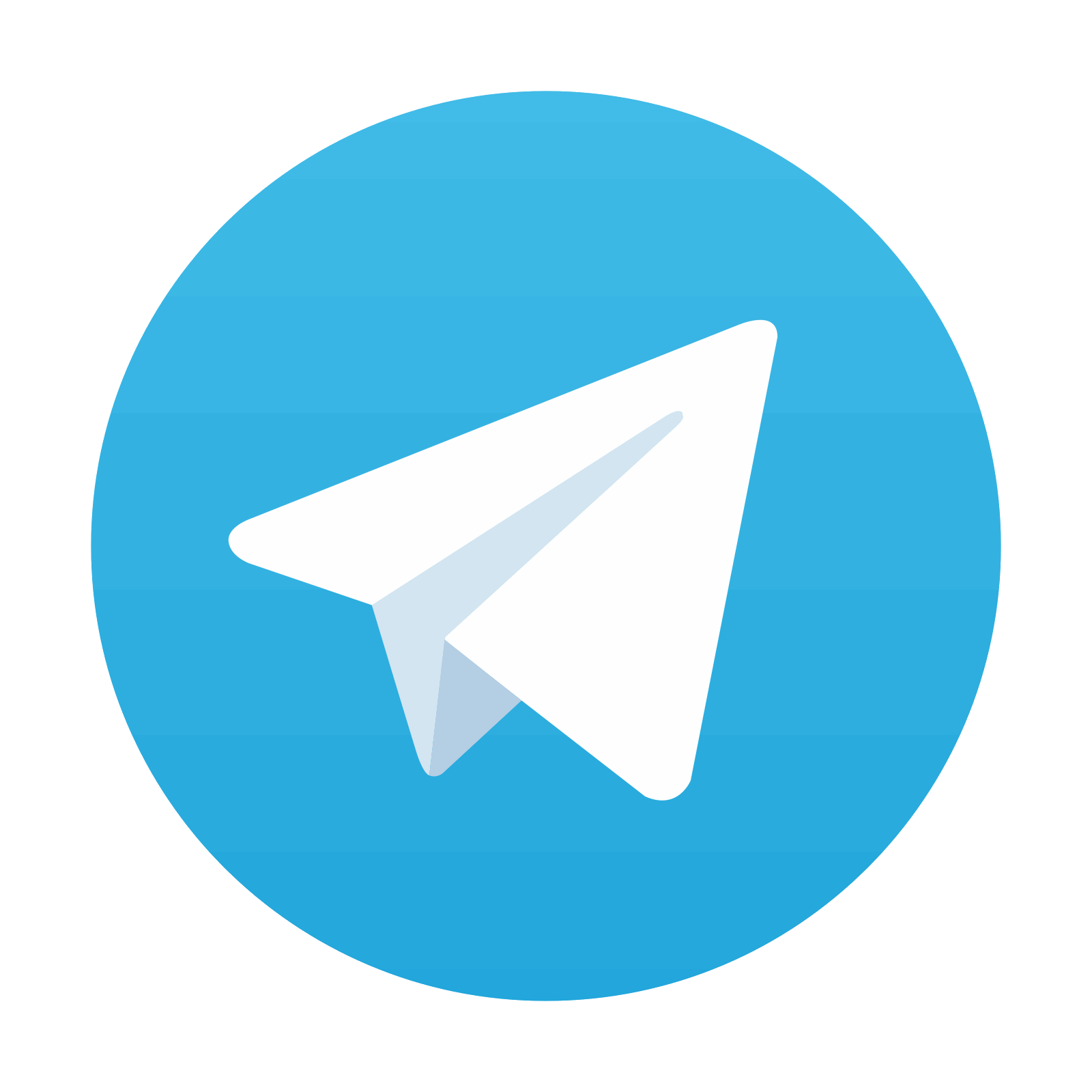
Stay updated, free articles. Join our Telegram channel

Full access? Get Clinical Tree
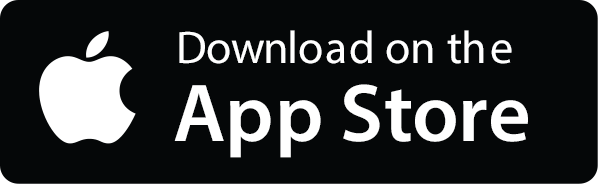
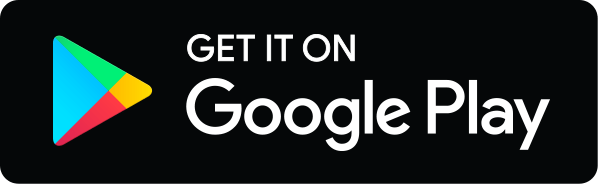