Optical Coherence Tomography in the Management of Age-Related Macular Degeneration
David Rhee
Darrell Baskin
INTRODUCTION
Since 1991, optical coherence tomography (OCT) has evolved from an experimental imaging system to an integral part of standard-of-care therapy for vitreoretinal diseases. Nowhere has this been truer than in the area of age-related macular degeneration (AMD). OCT is critical for nearly every aspect of AMD management, such as aiding diagnosis, establishing treatment and retreatment guidelines, and monitoring the response to treatment.
The United States Food and Drug Administration approved Macugen (OSI Pharmaceuticals/Eyetech, Melville, NY) and Lucentis (Genentech, Inc., South San Francisco, CA) in 2005 and 2006, respectively, as intravitreal injections for the treatment of all subtypes of neovascular macular degeneration. These marked the first commercially available treatments targeting vascular endothelial growth factor (VEGF). Additionally, multi-center, double-masked, placebocontrolled trials demonstrated that Lucentis is the first treatment for neovascular AMD that could actually improve vision.1,2 Worldwide, this has led to an upsurge in the number of intravitreal injections for the typical retina practice and has resulted in a significant rise in the use of OCT for AMD.
Due to the widespread increase in patients presenting to clinics requiring intravitreal injections, a quick, uncomplicated yet thorough method of retinal imaging to augment the clinical examination is needed. Fluorescein angiograms are an important aspect of the initial examination of new patients with AMD, but they prove too impractical for repetitive use. The typical angiogram costs several hundreds of dollars, requires a needlestick, and involves the injection of a systemic medication with the rare, but documented, complication of anaphylaxis. In addition, fluorescein angiograms require absolute cooperation and little or no movement on the part of the patient for each of the dozens of images acquired.
OCT technology, in contrast, is noninvasive and rapid. There are no systemic medications given, there are no known complications of the laser, and the entire scan encompasses a few seconds, which facilitates image acquisition and patient turnover. In addition, the easy and precise registration of a patient’s macula allows visit-to-visit monitoring of retinal pathology.3,4,5 In fact, the American Society of Retina Specialists Practices and Trends Survey in 20076 showed that 68.9% of retinal surgeons who responded used OCT alone for follow-up testing in AMD, as opposed to only 5.75% who use fluorescein angiography. Moreover, the majority of retinal surgeons who were polled used only OCT or OCT coupled with visual acuity change to determine retreatment in AMD. This represents a significant paradigm shift in practice patterns for the management of AMD, and it is unlikely to reverse in the foreseeable future.
NORMAL RETINAL ANATOMY SEEN ON OCT
The unprecedented speed and resolution of OCT technology has allowed ultramicroscopic structural evaluation of normal retinal architecture. OCT allows alterations due to macular pathology, such as AMD, to be easily identified as deviations from this normal architecture.7,8 In addition, the reproducibility of the measurements allows direct comparisons of the same pathology through time.3,4,5
In order to understand the effects of AMD seen with OCT, a thorough comprehension of the normal morphology of the macula is required. Our knowledge of the normal macular anatomy has evolved from numerous studies correlating pathologic specimens to OCT images.9,10 The following section will pay particular attention to the elements of macular morphology that undergo changes in AMD.
Sclera and Choroid
While the sclera and choroid are not typically thought of as areas of pathologic interest in AMD, OCT can highlight changes found in these layers that may occur with certain types of AMD. For instance, hyperpigmentation secondary to retinal pigment epithelium (RPE) hyperplasia in nonneovascular AMD leads to decreased visualization of the choroid. To understand this phenomenon, a detailed understanding of the normal OCT anatomy and how the choroid and sclera are represented is paramount.
While anterior segment OCT imaging modalities have been quite successful at visualizing the sclera anterior to the pars plana with a longer wavelength laser source,11 imaging of the posterior sclera has been historically difficult with standard, commercially available OCT machines. Although newer, ultra-high-speed OCT machines have improved scleral visualization,12 the widely used, time-domain-based Stratus OCT (Carl Zeiss Meditec, Dublin, CA) and newer commercial spectral-domain or Fourier-based OCTs do not adequately visualize the posterior sclera due to signal degradation, which occurs for a variety of reasons.
OCT imaging relies on analysis of reflected light from tissues (Fig. 23B.1). Optically reflective tissue, such as hard exudate or hemorrhage is represented by positive pixels (colored or gray-scale), whereas optically transparent media, such as the vitreous or serous fluid is represented by the absence of pixels.
The increased backscattering of reflected light from optically reflective tissue often has a two-fold effect on the OCT image. The first is that the pixels from highly reflective tissue will register a higher value than adjacent lesser reflective tissue. This leads to a brighter point on the OCT image or a different color (these are based on false coloring schemes provided by the OCT software—traditionally yellow/red for highly reflective, and green for less reflective, and black for optically transparent). Secondly, increased backscattering leads to pronounced shadowing of deeper, more posterior layers. This can be focal or diffuse, depending on the pathology. An important point to note is normal tissues also contribute to this shadowing effect by blocking the laser source.
Areas that are fluid filled, and therefore optically transparent, also have a significant effect on deeper layers. Fluid appears to dampen the laser’s effect through immediately adjacent posterior layers both diffusely, such as with cystoid macular edema, and focally, as with an inner retinal vessel (Fig. 23B.2).
Because the laser source from the OCT must pass through successive layers of reflective tissue, including the posterior hyaloid, the cellular layers of the retina, the RPE, the choriocapillaris, and the choroid, prior to reaching the sclera, enough signal degradation occurs that the scleral wall is usually absent on OCT images. The larger caliber vessels of the choroid compared to the much smaller choriocapillaris blunt the laser’s reflections with shadowing so much that the normal macular OCT typically shows only the first few vessel layers of the choroid, with absence of any deeper signals (Fig. 23B.1). Commercial SD-OCT machines do not appear to provide any advantage in this aspect. Thus, in the normal OCT of the macula, some aspects of the choroid are visible, and the sclera is not present.
Retinal Pigment Epithelium/Bruch’s Membrane/Choriocapillaris Layer
Recently, advances in experimental swept-source or frequency-swept OCTs have allowed inferential imaging and resolution of three distinct layers, consisting of the RPE, Bruch’s membrane (BM), and the smaller vessels of the choriocapillaris.13,14,15,16,17 However, for the majority of the commercially available time-domain and spectral-domain OCT imaging systems, the normal RPE is visually indistinguishable from both Bruch’s membrane and the choriocapillaris.
The RPE/BM/Choriocapillaris complex (or external band) typically appears as a strongly backscattering layer. The melanin in the RPE and the vascular nature of the choriocapillaris cause significant optical scattering. In addition, the thicknesses of Bruch’s membrane and the choriocapillaris typically range from 2 to 4 microns and 6 to 10 microns, respectively,18 but the advertised axial resolution of the Stratus OCT and the spectral-domain OCTs are 10 microns and 4 to 6 microns, respectively. The highly reflective nature and close proximity of the RPE and choriocapillaris combined with the limitations of axial resolution lead to the appearance of a single, hyperreflective (red on false color imaging) layer that is uniform in thickness and intensity throughout a typical 6-mm foveal scan (Figs. 23B.3 and 23B.4).
Therefore, the neurosensory retina traditionally corresponds to all the retinal layers anterior to this reflective band. As will be discussed later, the recognition of this anatomic landmark is critical for the management of AMD because it differentiates subretinal fluid (i.e., neurosensory detachment) from sub-RPE fluid (i.e., pigment epithelial detachment).
Neurosensory Retina
The photoreceptors are also an important OCT landmark because they delineate the outermost extent of the neurosensory retina. Immediately superficial to the RPE/BM/Choriocapillaris layer is another highly reflective band that represents the junction between the inner and outer segments of the photoreceptors (Fig. 23B.4). It is believed that this reflection may be a result of the difference in refractive index between the inner segments, which are relatively hypopigmented, and the outer segments, which have membranous segments rich in rhodopsin.19,20,21,22
The intervening hyporeflective layer between the RPE/BM/Choriocapillaris band and the junction of the inner and outer photoreceptor segments appears to correlate to the outer segments of the photoreceptors themselves. Oftentimes, there is an elevation of the IS/OS junction present in the area of the pathologic umbo (Fig. 23B.5), which may correspond to the lengthening of the photoreceptor outer segments of the cones within the fovea seen on histologic specimens.19 This is a normal finding and does not indicate the presence of other optically transparent media such as fluid.
On higher resolution systems, including some of the commercial spectral-domain systems and experimental ultra-high resolution or high-definition OCTs, the outer segment layer is represented by a third reflective band (Fig. 23B.4). This band is not resolvable by the lower resolution Stratus OCT, however, the clinical relevance of this band has yet to be proven in the management of retinal disease.
The nuclear layers of the retina, including the ganglion cell layer, the inner nuclear layer, and the outer nuclear layer contain the cell bodies of the neurosensory retina. These three layers are the most optically transparent in the retina, and appear dark green/black on false color imaging (Fig. 23B.6). They are separated from one another by the outer plexiform, inner plexiform, and nerve fiber layers or, the axonal layers of the eye, which are highly reflective and backscattering. The alternating pattern of nuclear and axonal layers leads to a striated pattern on the OCT image of the neurosensory retina (Fig. 23B.6). The nerve fiber layer is the only cellular layer of the retina that approaches the reflectivity of the junction of the inner and outer segments, and this reflectivity increases with the growing thickness of the nerve fiber layer as it marches toward the optic nerve. Thus, one can determine the nasal side of the macular OCT by judging the thickness of the nerve fiber layer.
The final structure visible in the neurosensory retina is the external limiting membrane. This is clearly visible with the ultrahigh resolution OCT and, less obviously with commercial time-domain and spectral-domain OCTs, as a thin, backscattering layer on the border of the posterior third of the outer nuclear layer. The external limiting membrane is not a true membrane, and is seen histologically and on OCT because of the nature of the alignment of the photoreceptors and the Müller cells.22,23,24
Preretinal Layers
In the absence of any pathology, the internal limiting membrane is not seen on the OCT. This is mainly due to the highly reflective nature of the nerve fiber layer. Similarly, the posterior hyaloid is not normally observed, unless it is separated from the nerve fiber layer such as with a posterior vitreous detachment or vitreomacular traction. In these situations, the posterior hyaloid appears in the vitreous cavity as a thin backscattering layer that may be inserting on the macula (in the case of vitreomacular traction or a senile macular hole) or may be hovering above the macula in the normally optically transparent vitreous (in the case of a posterior vitreous detachment) (Fig. 23B.7).
CHANGES IN THE OCT WITH AMD
It is of critical importance to be familiar with the normal OCT of the macula because any deviation from the norm may be secondary to pathology. The following sections will deal with the OCT findings that occur with the common pathologic changes in AMD. However, despite the incredible diagnostic resolution of OCT for AMD, no substitute exists for a detailed history, clinical exam, and fluorescein angiography, in addition to OCT, in the diagnosis of both dry and wet AMD. OCT should never be used as the sole method of macular examination.
Nonneovascular or Dry AMD
Although OCT is often utilized with the diagnosis and management of neovascular (also known as “wet”) AMD, OCT imaging can be incredibly informative in the diagnosis and management of nonneovascular or “dry” AMD.
To understand the architectural changes evident on OCT in AMD, one must understand that the OCT image is created by a laser light source passing through tissue. As previously discussed, thicker and more reflective tissue will cause more backscattering and subsequently less penetration of the laser into deeper tissue. This leads to attenuation of the incident light and will appear as shadowing of the more posterior layers of tissue. It is not hard to imagine that a thinner retina would lead to increased penetration of the laser and more incident light, or more backscattering from deeper, more posterior layers of the eye. Such is the case with pathologic myopia and age, where the thinner retina allows more of the posterior choroid and sometimes, the sclera, to be seen.
Similar to the myopic eye, diffuse thinning of the retina appears to occur with age, an effect exaggerated at the capillary-free zone of the macula.25 This leads to blunting of the foveal reflex, and a loss of overall retinal thickness. In the OCT of the nondiseased, aged eye, therefore, attenuation of the incident light from backscattering of the cellular retinal layers is reduced. In this case, a deeper zone of choroidal vessels may be visualized.
RPE Derangements
The diffuse thinning of the retina with age is often associated with AMD, as this a disease process primarily affecting elderly patients. In AMD however, this thinning is coupled with characteristic derangements of the RPE, which may be exhibited clinically as pigmentary clumping. This may lead to irregular thickening and/or discontinuity of the RPE/BM/Choriocapillaris layer on OCT imaging (Fig. 23B.8). The irregular thickening may balance out the diffuse thinning of the retina due to age, such that the net reflections from the choroid may be close to normal. However, the discontinuities in the RPE may lead to increased penetration of the laser and more choroidal backscatter.
Drusen and Drusenoid Pigment Epithelial Detachments
Drusen are a well-documented fundus abnormality associated with nonneovascular AMD.26,27 Histologically, the drusen associated with AMD are deposits of extracellular material that have collected external to the RPE basement membrane and the inner layers of Bruch’s membrane,28,29,30 which are classically referred to as basal linear deposits. This is in contrast to the basal laminar deposits associated with basal laminar drusen and Mallattia Levitinese which are typically located within the basement membrane of the RPE. While basal linear and basal laminar deposits can be clearly distinguished on pathologic slides, the resolution of OCT is not detailed enough to differentiate the two. Both appear as irregular thickening and/or elevation of the RPE/BM/Choriocapillaris band.
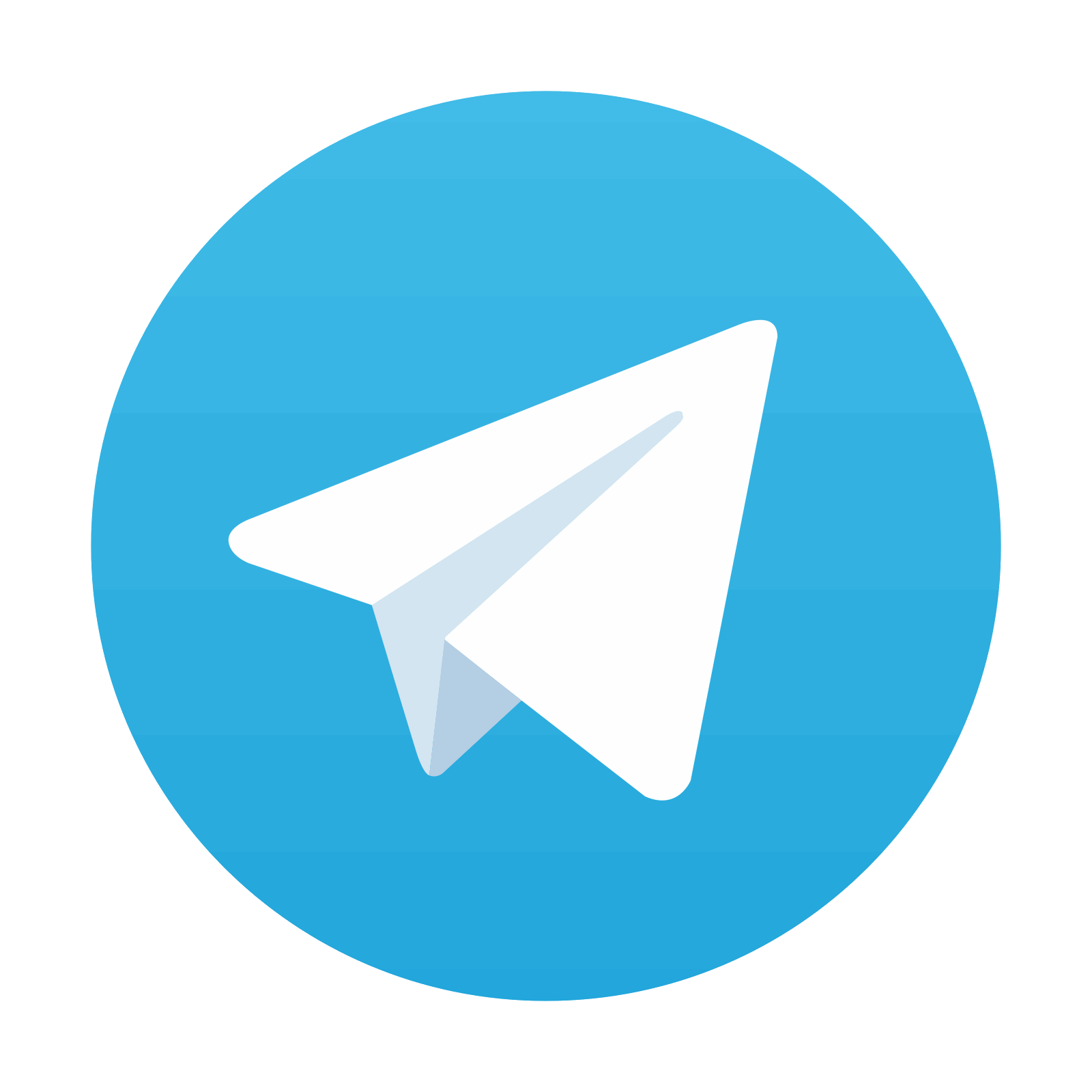
Stay updated, free articles. Join our Telegram channel

Full access? Get Clinical Tree
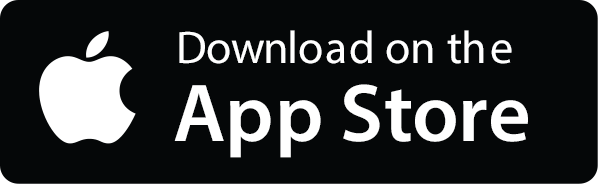
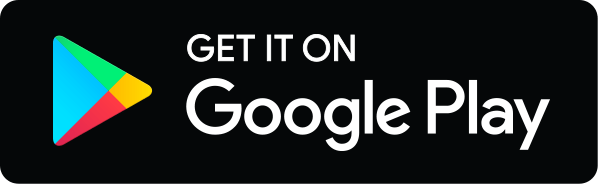