Introduction
Over the past 25 years, there has been a dramatic uptake in the usage of optical coherence tomography (OCT) as a noninvasive clinical tool to evaluate the structural anatomy of the macula and optic nerve head. Following its initial release as a research tool in the early 1990s, it reached mainstream clinical usage in the early 2000s with time domain technology, but really took off after 2005 with the release of Fourier domain or spectral domain OCT, which featured vast improvements in imaging resolution due to the higher scanning speeds coupled with motion correction technologies, such as eye tracking.1 Present-day clinical retina practice is characterized by a combination of a clinical exam, OCT imaging to evaluate the structural anatomy of the macula, fluorescein angiography (FA) to evaluate the retinal vasculature and identify sites of leakage or staining, and on occasion indocyanine green (ICG) angiography to better evaluate the deeper choroidal circulation. Practice patterns as defined by the graduating Retina Fellows at the Retina Fellow Forum have shown an increasing reliance on OCT imaging to define clinical activity and a reducing usage of FA in the diagnosis and management of retinal diseases ( ▶ Fig. 1.1, ▶ Fig. 1.2). Since 1961 when FA was first used to image the retinal vasculature, it has been the gold standard for evaluating the retinal vasculature and retinal conditions characterized by leakage or staining. Although the capabilities of FA are well known to all retina specialists, so too are its risks, related to the intravenous injection of a dye, which is associated with nausea, vomiting, and anaphylactic shock.2,3,4,5 It also requires a significant investment in time, equipment, and well-trained personnel to perform properly.
Fig. 1.1 Decreasing utilization of fluorescein angiography on new patients with WET ARMD. ARMD, age-related macular degeneration.
(Data from graduating retina fellows at the North American Retina Fellow Forum, 2010–2015.)
Fig. 1.2 Decreasing utilization of fluorescein angiography for patients with WET ARMD on Anti-VEGF therapy. ARMD, age-related macular degeneration; VEGF, vascular endothelial growth factor.
(Data from graduating retina fellows at the North American Retina Fellow Forum, 2010-2015.)
1.2 So What Is Optical Coherence Tomography Angiography and Why Is It So Exciting?
Over the last few years, the advances in imaging speeds and resolution of OCT platforms have resulted in the ability to detect blood flow by motion contrast and by extension provide an en face view of the retinal vasculature. The underlying principle behind this is that sequential B-scans are taken of the SAME retinal location and then subjected to analysis to determine if there was any change in the amplitude or phase of the scan. If changes are detected, this signifies movement in the retinal tissue of this location ( ▶ Fig. 1.3). The movement is inferred to be due to the flow of red blood cells in the vasculature, although there are occasional artifacts that can create a “false” impression of movement of the retina. The obtained signal can then be amplified (ex SSADA—split spectrum amplitude decorrelation angiography) and digitally processed to provide an en face view of the vasculature at different layers of the retina. Various motion correction technologies are also typically applied to the data to further enhance the quality (signal-to-noise ratio) of the obtained images ( ▶ Fig. 1.4).6,7,8,9,10,11,12 The data are obtained in the typical manner that a structural OCT cube scan is done and in the same time sequence approximately 3 to 4 seconds. So, it importantly does not change the flow in a busy clinical retinal practice. Even better, and without question the most outstanding feature of OCT angiography (OCTA) is that this is all done without the injection of a contrast dye, so no intravenous (IV) and no risks of allergic reactions. OCTA is NONINVASIVE!
Fig. 1.3 Basic principle behind optical coherence tomography (OCT) angiography: sequential B-scans are taken of the retina at the exact same location. These scans are then compared to look for any changes in the signal. When present, this is deemed to represent movement in the retina at this location and inferred to represent blood flow. The deviation in the signal is mathematically assessed to provide a decorrelation signal representing the amount of blood flow at that point on the retina.
Fig. 1.4 Motion correction technology. (a) Horizontal priority fast transverse scans (Fast-X), (b) vertical priority fast transverse scans (Fast-Y) are taken, and then (c) software analyzed (Optovue’s AngioVue system 2015) and merged to create a better-quality image free of motion artifacts including residual axial motion and transverse saccadic motions.
1.3 Making Sense of the Information Obtained in an OCTA
As every OCTA obtained is essentially a cube scan, it is a three-dimensional (3D) assessment of the retinal vasculature unlike traditional fluorescein or ICG angiography, which is two dimensional. As a result, to properly evaluate the data, an approach has to be taken to make sense of the volume of information. One approach could be to evaluate the scans from the inner retinal surface right down to the choroid in a continuous manner. This could be done manually or by creating a movie file of the scans. Both of these options require significant physician time and as a result there would be reluctance to use the data in this manner. In an attempt to simplify the data, most commercially available OCTA machines have taken the cube and split it into slabs to reflect a known anatomic layer of the retinal vasculature, referred to as autosegmentation. For instance, the AngioVue software on the Optovue OCTA splits the volume cube up into the following four slabs:
Inner retinal slab extends from 3 µm below the internal limiting membrane to 15 µm below the inner plexiform layer. This incorporates the known anatomic location of the superficial retinal vascular plexus, which is generally what we see on traditional FA ( ▶ Fig. 1.5).
Middle retinal slab extends from 15 µm below the inner plexiform layer to 70 µm below the inner plexiform layer and incorporates the known location of the deep retinal capillary plexus. This plexus is poorly seen on traditional FA and beautifully seen on OCTA. Evaluating this region on OCTA is already providing insights into the pathology of conditions such as parafoveal telangiectasia (PFT) type 2b, paracentral acute middle maculopathy (PAMM), and retinal angiomatous proliferation (RAP), which we were unable to visualize clearly on traditional FA ( ▶ Fig. 1.6).
Outer retinal slab extends from 70 µm below the inner plexiform layer to 30 µm below the retinal pigment epithelium (RPE) reference line. This region anatomically corresponds to a part of the retina within which there is NEVER any vasculature in a normal individual. As a result, this slab should always be empty or blank unless there is pathology ( ▶ Fig. 1.7). This slab can be very useful to identify type 2 (subretinal) neovascular membranes.
Choriocapillaris extends from 30 µm below the RPE reference to 60 µm below the RPE reference. It incorporates the choriocapillaris and allows detection of early type 1 (sub-RPE) choroidal neovascular membranes (CNVM; ▶ Fig. 1.8).
Fig. 1.5 Inner retinal slab (Optovue’s AngioVue system) extends from 3 µ below the internal limiting membrane to 15 µ below the inner plexiform layer. (a) The optical coherence tomography (OCTA) image obtained looks very similar to the view obtained on traditional fluorescein angiography. (b) The traditional anatomic structure of the superficial retinal vascular plexus.
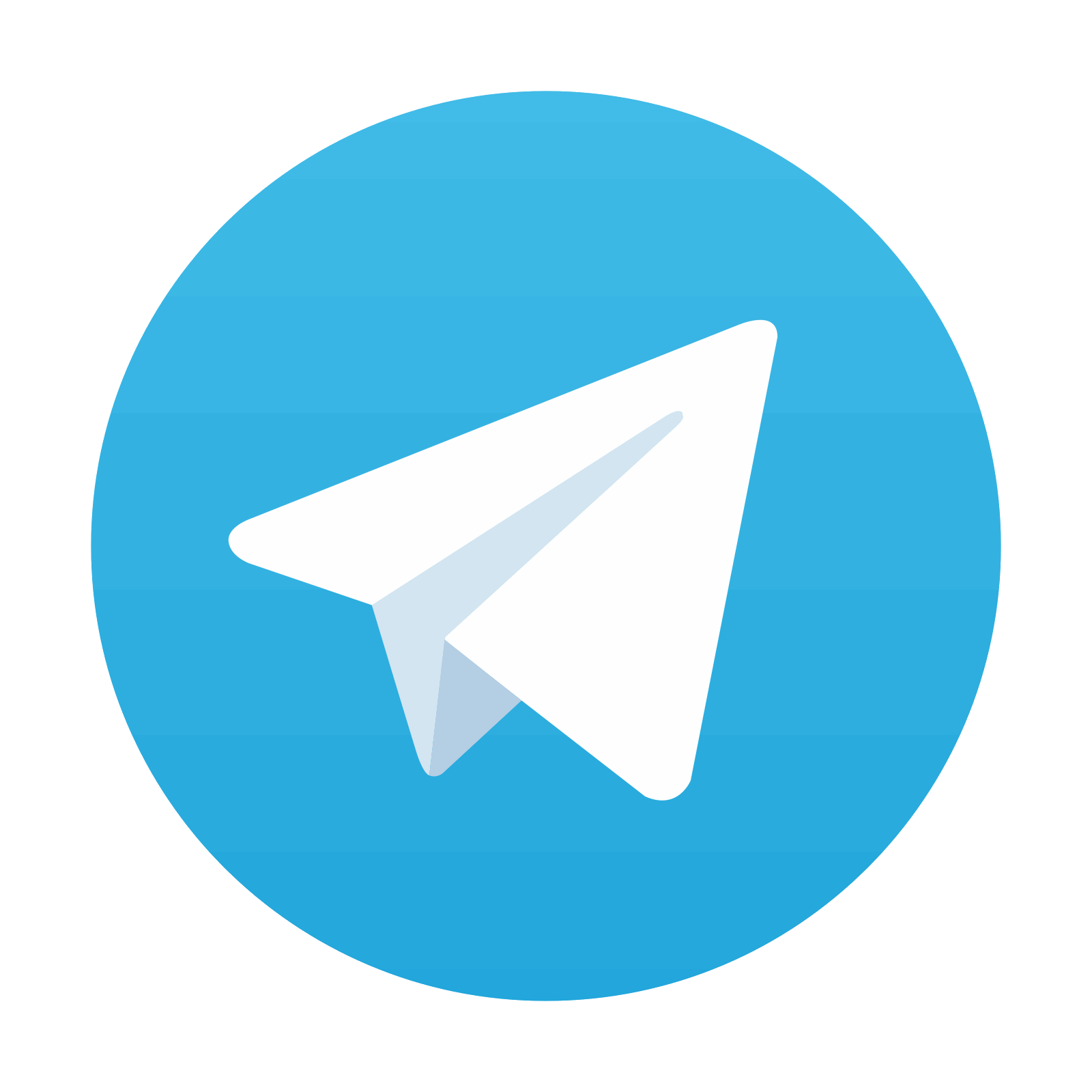
Stay updated, free articles. Join our Telegram channel

Full access? Get Clinical Tree
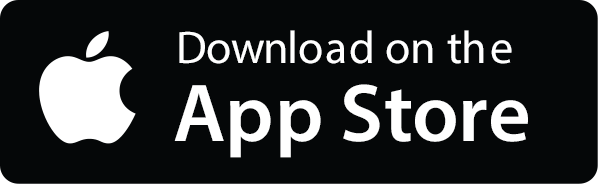
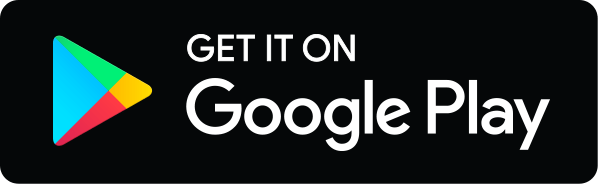