Central retinal artery occlusion (CRAO) presents as sudden, catastrophic visual loss and is therefore, one of the most important topics in ophthalmology. Similarly, branch retinal arterial, or better termed arteriolar, occlusion (BRAO) causes sudden segmental visual loss that may recur to involve other branch retinal arterioles. The incidence of CRAO has been estimated to be 1 to 10 in 100,000.1 Symptomatic BRAO is even less common. Yet, acute retinal arterial occlusions (RAOs) are among the major causes of acute visual loss. Accurate and timely diagnosis of RAO is critical because permanent retinal damage may ensue by 4 hours following the initial ischemic insult.2 Additionally, visual deficits may be the initial presenting sign in individuals at risk of morbidity from systemic embolic events. The arterial blood supply to the retina and choroid is composed of two main systems both derived from the ophthalmic artery. The ophthalmic artery is the first branch of the internal carotid artery and enters the orbit through the optic canal below the optic nerve. The central retinal artery (CRA), the primary source of blood supply to the retina, is the first intraorbital branch of the ophthalmic artery that passes into the optic nerve at 8 to 15 mm behind the globe to provide the blood supply to the inner retina.2 The outer retina and choroid receive blood supply from the short posterior ciliary arteries (PCAs) that branch distally from the ophthalmic artery. The main branches of the CRA course horizontally throughout the superficial nerve fiber layer (NFL), intermittently diving into the deeper layers. From their terminal arterioles and venules, precapillary and capillary tributaries sprout nearly perpendicular to the retinal surface, bending into the deeper retinal layers where they anastomose laterally to form distinct microvascular capillary systems. The superficial system resides predominantly within the NFL and ganglion cell layer. Approaching the optic disc, the NFL component is more prominent and the capillary bed is referred to as the radial peripapillary capillary (RPC) plexus, but as it moves toward the fovea, these vessels predominantly localize within the ganglion cell layer and are referred to as the superficial capillary plexus (SCP). The RPC plexus plays an important role in the development of several lesions such as cotton wool spots (CWS) that are often located along its distribution.2 The deeper system is composed of an intermediate capillary plexus (ICP) and a deep capillary plexus (DCP) located at the inner and outer planes of the inner nuclear layer (INL), respectively. Consequently, in the region of the central macula, an intricate array of capillary networks exists within a superficial, intermediate, and deep trilaminar system that are interconnected by perpendicularly oriented diving vessels. It has been proposed that these individual layers of the retinal vasculature may be differentially affected by ischemic retinal vascular disease.3 Notably, the foveola receives its blood supply from the choroidal circulation and is not supplied by the CRA or its branches. An important anatomical variation among 15 to 20% of the population is the presence of a cilioretinal artery that usually arises from the peripapillary choroid or directly from one of the short PCAs. The cilioretinal artery has a characteristic hooklike appearance at its site of entry into the retina at the optic disc margin, usually on the temporal side and less frequently elsewhere. The size and area of the retina supplied by a cilioretinal artery vary widely from a minute artery supplying a small area of the peripapillary retina to supplying half or even the entire retina.2 When present, the cilioretinal artery may provide additional blood supply to the retina, including the nerve fibers from the foveal photoreceptors, which could result in areas of preserved central vision in cases of central retina artery occlusion (CRAO) as demonstrated in ▶ Fig. 8.1. However, it is also possible for the cilioretinal artery to occlude in isolation, causing central visual field loss with a perfused macula region. Fig. 8.1 Multimodal imaging of acute central retinal arterial occlusion in a 24-year-old female with light perception in the left eye and 20/40 vision in the fellow eye. Left column shows affected eye and right column represents normal fellow eye. Color fundus photograph demonstrates retinal whitening with a “cherry-red spot” at the (a) foveola. Note the spared area nasal to optic nerve perfused by the cilioretinal artery. Fluorescein angiography (FA) demonstrated delayed transit and late hyperfluorescence consistent with (b) macular edema and increased thickness on (c) optical coherence tomography (OCT). Furthermore, decreased arterial perfusion is evident in the macula (yellow dashed region) on FA by comparing areas of hypofluorescence to the (d) fellow eye. OCT angiography of similar regions demonstrates flow void with more capillary detail. This further enables enhanced visualization of the (e) foveal avascular zone. BRAO usually results from embolic obstruction of a division of the retinal artery, while CRAO results from occlusion of the retinal artery before it branches either at or proximal to the optic disc. CWSs are common, acute, nonspecific retinal lesions, seen in retinopathies due to a whole host of conditions. They are the result of occlusion of the terminal retinal arterioles in the nerve fiber and ganglion cell layer, with focal nonperfusion of the retinal capillaries in their distribution, resulting in acute focal inner retinal ischemia and infarction.2 Individuals with RAO typically present with unilateral, painless sudden loss of vision or scotoma due to interruption of the blood supply to the retina. Acute obstruction of the retinal artery with subsequent collapse of capillary flow leads to axoplasmic stasis, intracellular edema, and ischemic necrosis of the inner retinal layers. This causes opacification of the NFL and inner retina, creating a glassy and whitened appearance within 15 minutes to several hours following the injury. This opacification is densest at the posterior pole, given the macular region, unlike the rest of the retina, has more than one layer of retinal ganglion cells, and it is the thickest part of the retina.2 The foveola assumes a “cherry-red spot” appearance because it remains nourished from the choroidal circulation, and thus, the retinal pigment epithelium and choroid remain intact underneath the fovea, while the surrounding retina opacifies ( ▶ Fig. 8.1). If the CRA becomes occluded, complete loss of vision occurs even though the central foveolar circulation is not affected owing to ischemia of the emanating NFL. Pigmentary changes are usually absent because the retinal pigment epithelium remains unaffected. The blood column in both the arteries and the veins can become segmented with separation of serum from Rouleau stacking of the red corpuscles leading to a “box-carring” appearance ( ▶ Fig. 8.2). The clinical appearance of retinal whitening resolves in 4 to 6 weeks over which course the obstructed retinal artery will usually recanalize and reperfuse with resolution of the edema. Nonetheless, the inner retinal damage is permanent, leading to atrophy, retinal vascular attenuation, and optic nerve pallor. Hence, visual acuity and/or field loss often do not recover after RAO, given the retinal damage becomes established rapidly with no effective way to reverse the obstruction. Conventional advocate treatments consist of ocular massage to dislodge a CRA embolus, reduction of intraocular pressure by various medical and surgical means to increase retinal perfusion pressure, vasodilation of the CRA by sublingual isosorbide dinitrate, rebreathing of expired CO2 in a bag, or breathing carbogen or retrobulbar vasodilators, antiplatelet therapy, and heparin therapy. Fig. 8.2 Multimodal imaging of acute branch retinal arterial occlusion in a 60-year-old female. Visual acuity was 20/40 in both eyes. However, the patient complained of a field defect in her left eye. (a) Fundus photo demonstrates multiple cotton wool spots along with retinal whitening, box-carring or cattle-trucking of flow and refractile lesions within the blood vessels. (b) Fluorescein angiography and (c) optical coherence tomography angiography at the (g) radial peripapillary capillary, and (i) superficial and (j) deep capillary plexus levels outline regions of decreased perfusion that correspond to (a,f) retinal whitening. B-scans through the areas of nonperfusion reveal absence of flow signal over areas of (h, k) hyper-reflectivity. Color-coded perfusion maps show the extent of (d,e) involvement. Establishing a clinical diagnosis of RAO is usually not difficult during the acute phase when retinal whitening and blood flow/vascular alterations are evident on examination. However, these signs may not be obvious very early in the course of disease, in eyes with partial RAO or several weeks after onset. Ancillary imaging studies consisting of intravenous fluorescein angiography (FA) and spectral-domain optical coherence tomography (SD-OCT) are useful to evaluate the presence and extent of retinal nonperfusion, foveal contour, and macular edema, to search for other vascular abnormalities, and to determine the presence or location of retinal emboli. Imaging may also be useful in atypical cases or if acute signs are not apparent. In the acute setting, FA delineates the extent of retinal involvement, seen as capillary nonperfusion and absent or delayed filling of the blood vessels distal to the obstruction. It specifically shows delays in both the arteriovenous transit time and retinal arterial filling with normal choroidal filling. Ophthalmic artery or carotid artery obstruction should be considered if there is delayed choroidal filling. In the chronic setting, FA may show arterial narrowing with normal fluorescein transit following resolution and recanalization of RAO. FA mainly provides information about large vessel flow and SCP but does not allow for assessment of the morphology of the DCP or RPC. In addition, assessment of the deeper retinal capillaries with FA may further be limited by light scattering from the opacified inner retinal layers.4 SD-OCT can be utilized to determine the specific retinal level of edema or subsequent atrophy during the chronic phase, when FA may no longer display any perfusion deficit. Optical coherence tomography angiography (OCTA) may have advantages over other imaging techniques. It enables three-dimensional and en face visualization of flow across desired slabs through the retinal and choroidal vasculature. Thus, it can reveal deficiencies involving acute flow interruption in RAO. In addition, it provides en face segmented imaging of the DCP and RPC, which are poorly imaged with FA, and reveals finer details of the SCP.5 Precise localization of retinal capillary ischemia with OCTA in RAO may help determine subsequent visual prognosis.2,4 Furthermore, it is noninvasive utilizing motion contrast and obviating the need for injectable dyes. This is especially beneficial for patients with concomitant medical problems who may not be able to tolerate dye-based angiography. ▶ Fig. 8.1 demonstrates a case of CRAO with a typical “cherry-red spot” and notable cilioretinal artery perfusion on clinical examination. FA and OCTA images have been compared to one another and between fellow eyes outlining areas of nonperfusion and demonstrating finer capillary details using OCTA. A case of acute BRAO is presented in ▶ Fig. 8.2. The retinal capillary nonperfusion is more easily discernable on OCTA than on FA. In addition to providing detailed imaging of perfusion status, the coregistered B-scans can also be used to assess retinal thickness and macular edema. Individual assessment of RPC, SCP, and DCP is also possible. Together, these allows for more accurate evaluation of the level and extent of involvement in the setting of arterial occlusions. Examples of such differential perfusion have been presented in a case of chronic BRAO ( ▶ Fig. 8.3) and CWS ( ▶ Fig. 8.4). Fig. 8.3 (a) Optical coherence tomography angiography (OCTA) of chronic branch retinal artery occlusion in an 18-year-old male with a prepapillary vascular loop. (b) Radial B-scan reveals inner retinal atrophy inferotemporal to the macula. OCTA shows decreased capillary perfusion in both retinal capillary networks on 3 × 3 and 6 × 6 mm scans. The extent of capillary nonperfusion appears less in the (c, d) superficial capillary network slabs compared to the (e, f) deep. Furthermore, arteries and arterioles remain perfused over areas of ischemia.
8.2 Pathogenesis and Diagnosis
8.3 Features of Arterial Occlusion on Optical Coherence Tomography Angiography
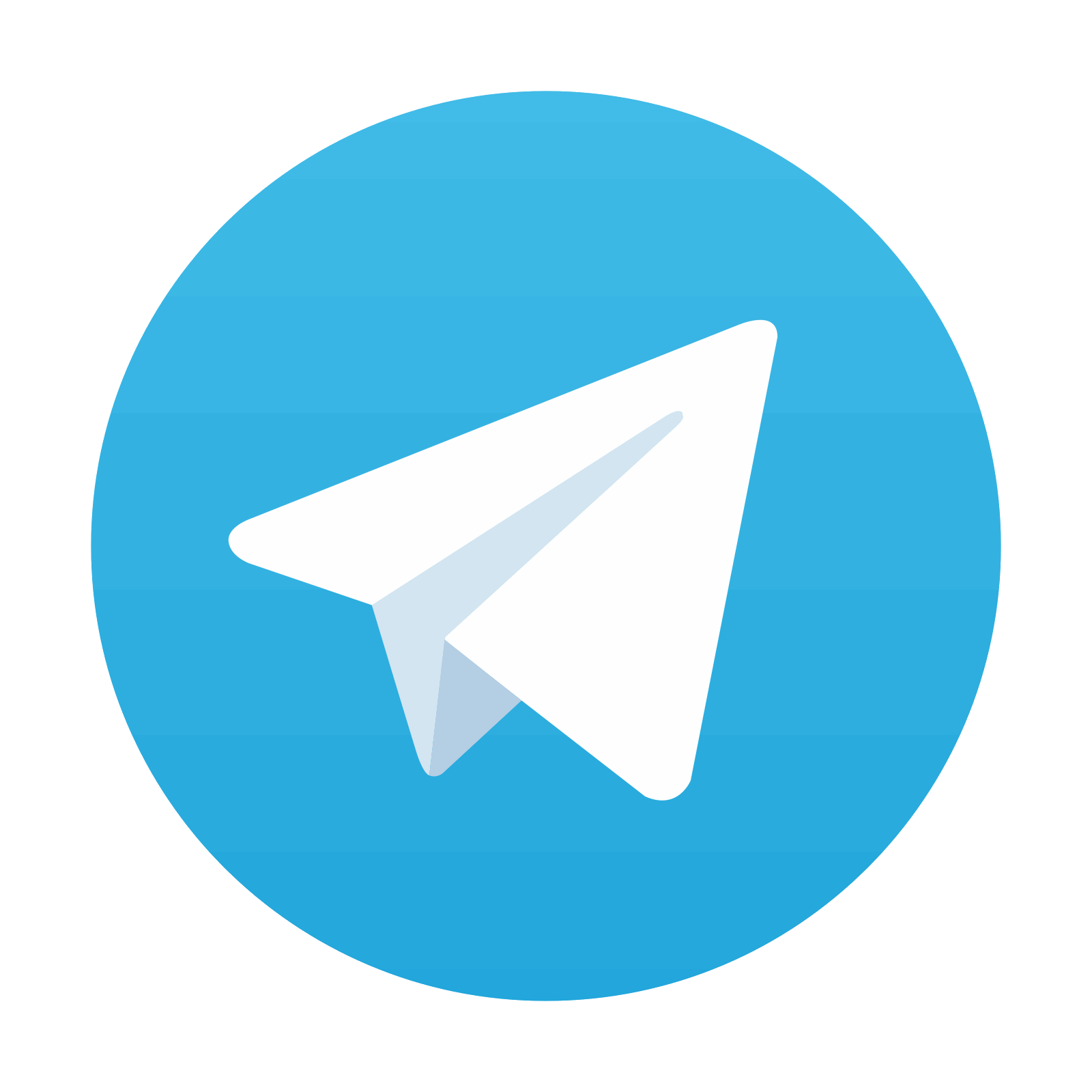
Stay updated, free articles. Join our Telegram channel

Full access? Get Clinical Tree
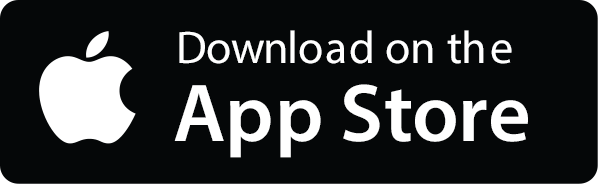
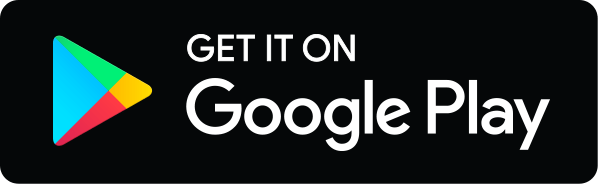