Optovue AngioVue The underlying technology of the Optovue AngioVue system is the Avanti SDOCT wide-field imaging system. The Avanti system utilizes an 840-nm light source with a bandwidth of 50 nm and has an A-scan rate of 70,000 scans per second.1 The system has an axial resolution of 5 µm, transverse resolution of 15 µm, and an A-scan depth of approximately 3 mm. The fast scanning speed is needed for acquiring the large angiography dataset in a reasonable time. To generate the OCTA image, the system acquires three-dimensional (3D) data acquisition volumes consisting of 304 × 304 A-scans in approximately 3 seconds and then uses orthogonal registration and merging of two consecutive scan volumes to obtain the 3 × 3, 6 × 6, or 8 × 8 mm OCTA volumes. AngioVue DualTrac Motion Correction Technology (MCT) provides enhanced flow visualization and ultraprecise-motion correction. During the OCTA capture, infrared (IR) video is used for tracking on each fast-X and fast-Y scans so that each OCTA image will have minimum eye motion artifacts. The tracking improves patient comfort by allowing blinks and fixation drifts during acquisition. Before motion correction, the system utilizes a split-spectrum amplitude-decorrelation angiography (SSADA) algorithm to extract the OCTA information2 from each fast-X and fast-Y OCTA scan. The SSADA algorithm detects motion in the blood vessels by measuring the variation in the reflected OCT signal amplitude between consecutive scans.1,3 The decorrelation (1-correlation) of the signal amplitude between consecutive B-scans is then calculated allowing for evaluation of the contrast between tissue with motion and that without motion, that is, blood flow. Since decorrelation can also be generated by bulk eye motion, the SSADA algorithm splits the original full spectrum to 11 subspectrum OCTA images. While this lowers the resolution of the image, it also results in less susceptibility to eye motion and a wider coherence gate over which a moving blood cell can interfere with adjacent structures. Each of the split-spectrum images contains a different speckle pattern that also has independent information on flow. By combining the images from the various spectral bands, the flow signal is intensified. Increasing the number of spectral bands that are analyzed enhances the signal-to-noise ratio and increases the ability to detect flow. The use of the orthogonal OCTA volumes is necessary to apply motion correction algorithms. The postprocessing MCT increases the number of patients that can be successfully scanned compared to independent use of real-time tracking and enhances microvascular flow visualization. MCT is necessary to minimize OCTA artifacts related to microsaccades and fixation changes.4 Without motion correction, bright lines would appear in the OCTA image due to saccade motion. Since an eye takes 1 millisecond to move 300 µm, an OCT device would need to have 10 kHz tracking speed to get 30-µm accuracy. While 1-kHz laser tracking systems could perform this tracking, it would be very expensive. Most scanners use image tracking that tracks at a 15- to 30-Hz speed—in general too slow for OCTA. The AngioVue system uses a sophisticated algorithm that performs a rough axial correction in the first stage, then a full optimization in the second stage to produce the merged image. The motion-corrected data improves signal-to-noise ratio and corrects for z-motion artifacts. This requires considerable computational power. The registered and merged data output is used to apply the angiography algorithm. The flow information is then presented as an en face image with segmentation utilized to show different levels of vasculature. The AngioVue software generates four default en face imaging zones: the superficial plexus, the deep plexus, the outer retina, and the choriocapillaris.5 The software can also be manipulated to show a custom region of interest. The AngioVue software also does quantitative analysis of the OCT angiograms. The new quantification tool is called AngioAnalytics. This tool provides numerical data about flow and nonflow areas. This information is used to generate a flow density map that may be used to track the change in perfusion density over time. This data should be taken with a grain of salt, however, as the SSADA algorithm is felt to saturate at higher velocities such as those seen in larger vessels.6 The software also allows the user to draw the borders of the choroidal neovascularization and the software will calculate the drawn area and the vessel area. It also generates maps of nonflow areas that can be tracked over time. Most of the new software is still in its infancy and each iteration adds more features. The Carl Zeiss Meditec AngioPlex system is based on an upgraded Cirrus 5000 instrument. This SDOCT system has an A-scan rate of 68,000 A-scans per second with a light source centered on 840 nm with a bandwidth of 90 nm. The system has an axial resolution of 5 µm, transverse resolution of 15 µm, and an A-scan depth of 2.0 mm. The system incorporates a retinal tracking technology to track and compensate for eye movements in real time, and using a proprietary algorithm allows the device to rescan areas that may have been affected by motion. This system also allows for registration between visits so that the exact same area can be imaged on consecutive visits. Postacquisition motion correction software is not part of the device. There are three patterns available for imaging on the AngioPlex: 3 × 3, 6 × 6, and 8 × 8 mm. The OCTA analysis shows six en face slabs representing the inner capillary plexus, outer capillary plexus, the outer retina, which should normally be avascular, the choriocapillaris, the choroid, and vasculature above the vitreoretinal interface, which should also normally be avascular. The AngioPlex software also combines three of the retinal en face slabs to provide visualization of microvasculature in the entire retina and also uses color mapping to show flow in the superficial, deep, and avascular layers as red, green, and blue, respectively, in a color depth–encoded slab. As with AngioVue, the AngioPlex device also captures consecutive B-scans; the differences lie in how the data is then processed by the device to generate the final en face images. The AngioPlex device uses the optical microangiography (OMAG) algorithm to generate the final en face OCTA images instead of the SSADA algorithm.7 The OMAG algorithm examines the signal differences in successive B-scans at the same location and records these as vectors utilizing both the amplitude and the phase of the signal, which are then used to create a picture of changes in the microscopic structures encountered.8,9 The resulting image relies on the contrast in the OCT signal created by motion of scattering elements, which is primarily expected to be due to flow of erythrocytes through vessels. OMAG allows for high-sensitivity imaging of capillary flow. The high sensitivity of OMAG makes it susceptible to bulk-motion artifacts and the algorithm can only be utilized with precise-motion correction.3,10 Because the bulk-motion correction and precise-motion correction are part of the implementation in AngioPlex, the device can offer high sensitivity to microvascular flow, with no loss of axial resolution that occurs with use of narrower spectrum of wavelength. The Nidek RS-3000 Advance OCT is a SDOCT system with AngioScan OCTA software. Like the Cirrus AngioPlex, OCTA can be added to recent OCT devices via a software update. In some cases, a replacement of the PC unit is required. The AngioScan software uses the tracing high-definition (HD) function of the RS-3000 to track eye movements, which is necessary for the OCTA software to insure the sequential images are taken from the same place. The scan size ranges from 3 mm to a maximum of 9 mm. Using a composite function, a wide-angle panoramic OCT image up to 12 × 9 mm2 is also possible. The resulting image can be imaged en face and with false color representing the depths of the vascular channels. The software can remove the projection artifacts from the inner vasculature when viewing the outer vasculature. Proprietary software offers measurement of areas of ischemia, area of the foveal avascular zone, and a density map of the vasculature. A unique mode of the AngioScan software displays choroidal neovascular membrane (CNV) blood flow independent of the normal vasculature. This makes it easier to highlight areas of neovascularization. Like the other devices, this software is still in its infancy and is undergoing significant improvement as software algorithms get optimized. The Topcon DRI OCT Triton is an SSOCT system that has a number of advantages over SDOCT systems. The DRI OCT uses a 1,050-nm light source with a 100,000 A-scan per second scan rate.11 Since the system uses a longer wavelength light source compared to SDOCT devices, it has improved penetration into tissue; therefore, it should theoretically be able to image the choroid better. Additionally, the use of a 1050-nm light source should be more comfortable for the patient given it is in the IR spectrum and is not visible to the patient. Similar to the other two devices described earlier, the DRI OCT can generate en face OCTA images of either 3 × 3 or 6 × 6 mm. The DRI OCT includes motion correction to help account for patient movement. The DRI OCT uses yet another proprietary algorithm to produce the OCTA images—OCTA ratio analysis (OCTARA).12 The Topcon OCTARA algorithm does not require splitting the spectrum and thus maintains the full axial resolution. Unlike the other two devices described earlier, the faster speed of the SSOCT system allows for each B-scan position to be scanned four times. The B-scans at each location are then registered to each other and the OCTA images are generated by computing a ratio-based result between corresponding image pixels. This represents a relative measurement of OCT signal amplitude change while optimizing angiographic visualization over the retina and choroid. The system suppresses motion artifacts by averaging multiple registered B-scans. The DRI OCT software displays en face OCTA images corresponding to the superficial and deep capillary plexus as well as the choriocapillaris by default. In addition, the corresponding OCT cross-sectional image, IR fundus image, and en face OCT are also shown for comparison. There are a few other companies currently developing OCTA systems, but they were not readily commercially available at the time of completion of this chapter. These include the Spectralis OCTA (Heidelberg Engineering, Inc., Heidelberg, Germany) and the Canon Angio eXpert (Canon, Inc., Tokyo, Japan). Each of these devices uses a different software and hardware approach. Information on the specifics of each device was not available at the time of publication of this chapter. Unfortunately, there have been no published studies comparing the en face OCTA images generated by the various commercially available systems to each other in the same patient. As a point of reference, example images from some of these systems in normal patients are shown in ▶ Fig. 3.1, ▶ Fig. 3.2, and ▶ Fig. 3.3. ▶ Fig. 3.4 and ▶ Fig. 3.5 show examples of CNV on the AngioView and AngioPlex. ▶ Fig. 3.6 shows an image of geographic atrophy assessed by the OMAG algorithm, in the Zeiss AngioPlex software. Fig. 3.1 Normal patient imaged and analyzed on Optovue AngioVue software. The software shows four optical coherence tomography (OCT) angiographic views: superficial capillary plexus, deep capillary plexus, the outer retina, and the choriocapillaris as well the corresponding horizontal and vertical OCT B-scans. The red and green lines on the B-scans correlate to the area being analyzed by the OCTA algorithm in the large OCTA image displayed. These can be manually manipulated in the software to show a targeted area of interest.
3.3 Carl Zeiss Meditec AngioPlex
3.4 Nidek RS-3000 Advance Optical Coherence Tomography
3.5 Topcon DRI OCT Triton
3.6 Others
3.7 Comparisons
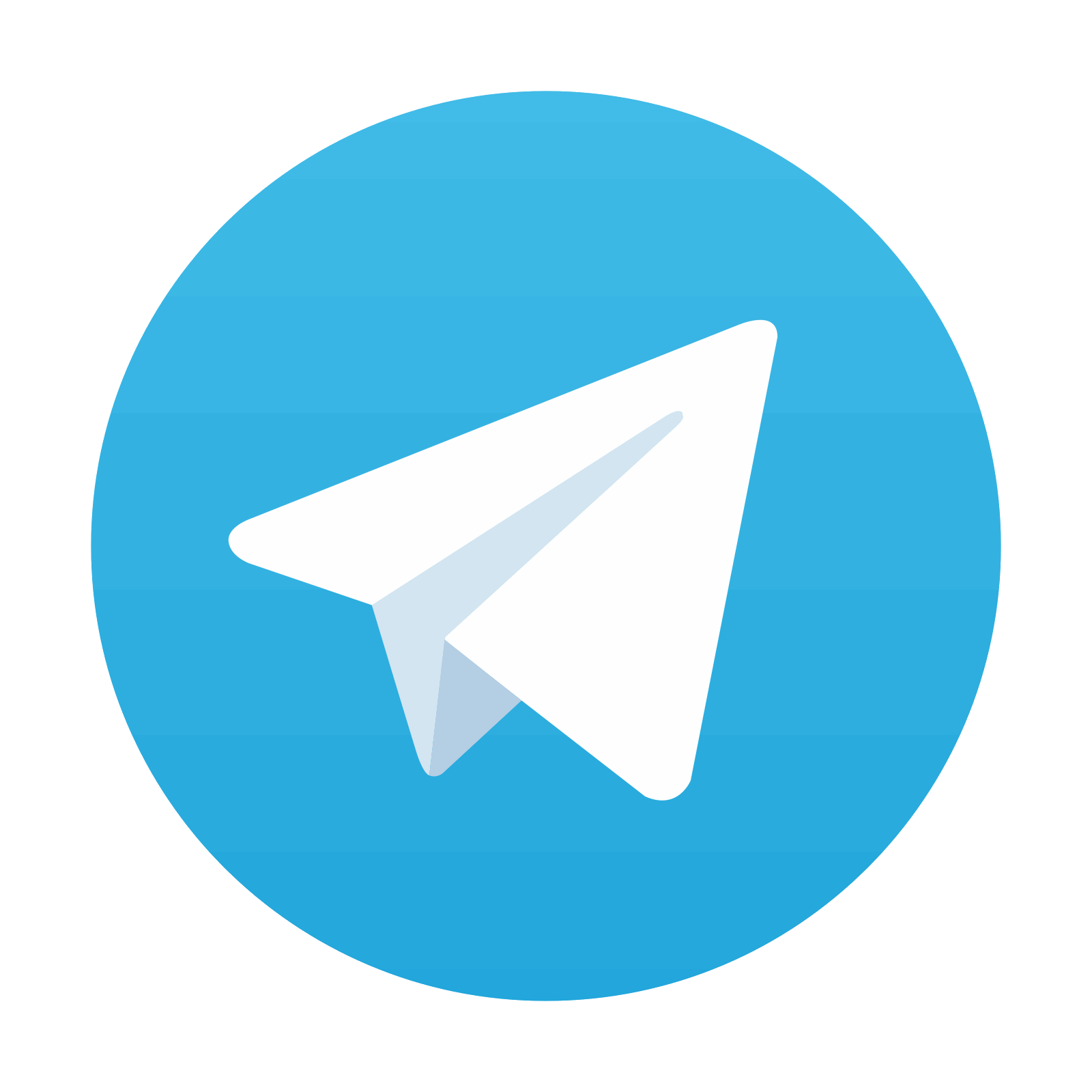
Stay updated, free articles. Join our Telegram channel

Full access? Get Clinical Tree
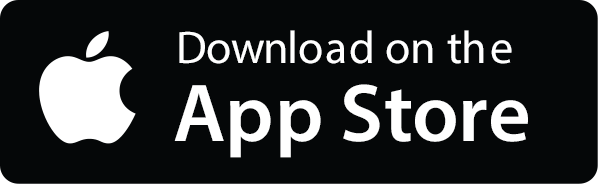
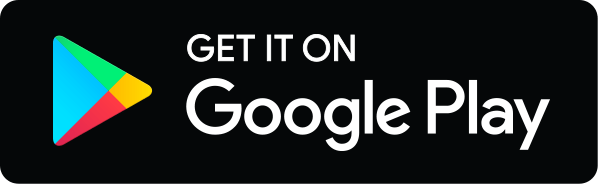