Optical Coherence Tomography
Caio V. Regatieri
Robin A. Vora
Jay S. Duker
INTRODUCTION
Age-related macular degeneration (AMD) is the leading cause of severe visual loss in adults older than 60 years (1,2). It is estimated that approximately 30% of adults older than 75 years have some sign of AMD and around 10% develop advanced stages of the disease. More than 1.6 million people in the United States currently have one or both eyes affected by an advanced stage of AMD, and it is estimated that there are another 7 million individuals “at risk” (1). Due to rapid aging of the population in many developed countries, this number is expected to double by the year of 2020 (1,3). Although neovascular AMD only accounts for about 10% to 20% of the overall AMD incidence, this subtype is responsible for 90% of cases of severe vision loss (20/200 or worse) (4,5).
Until recently, fluorescein angiography (FA) was the primary imaging modality used to detect choroidal neovascularization (CNV) presence and activity. Although FA is useful for determining presence of leakage in neovascular AMD, this technique does not provide any three-dimensional anatomic information about retinal layers, the retinal pigment epithelium (RPE), or the choroid. The development of optical coherence tomography (OCT) makes it possible to have cross-sectional images of the macula or optic nerve head analogous to ultrasonography.
Instead of sound, OCT uses light waves to obtain a reflectivity profile of the tissue under investigation (6). Light from a broadband light source is divided into a reference beam traveling a delay path and a sample beam that is directed onto the subject’s retina. Light backscattered by retinal structures interferes with light from the reference beam. The interference of echoes is detected to create a measurement of light echoes versus depth. The first commercially available OCT systems such as the Stratus OCT (Carl Zeiss Meditec, Inc., Dublin, CA) used time-domain (TD) detection, in which the reference mirror position and delay are mechanically scanned to produce axial scans (A-scans) of light echoes versus depth. Scan rates of 400 A-scans per second with an axial resolution of 8 to 10 µm in the eye are achieved with the Stratus OCT (7). In cases with active neovascular AMD, OCT can be used to detect signs of activity and to establish a baseline retinal thickness, volume, and fluid involvement. Additionally, OCT is particularly helpful in identifying the location and level of CNV (intraretinal, subretinal, sub-RPE) and other lesion components (blood, fluid, pigment, and fibrosis) (8). In cases suspicious for exudative changes, OCT can be extremely helpful in detecting suspected intraretinal, subretinal, or sub-RPE fluid, especially when angiographic interpretation is equivocal, inconvenient, or not readily accessible (9,10).
Spectral domain (SD), a type of Fourier domain detection, uses a high-speed spectrometer to measure light echoes from all time delays simultaneously enhancing OCT capabilities. The reference mirror does not require mechanical scanning. Improved sensitivity enables dramatic improvements in sampling speed and signal-to-noise ratio (11,12). SD detection, coupled with improvements in light sources, achieves axial scanning speeds of greater
than 20,000 A-scans per second with an axial resolution of 3 to 7 µm in the eye (Fig. 13.1). At present, at least nine commercial spectral/Fourier domain OCT systems are available worldwide. SD-OCT has emerged as the ancillary examination of choice to assist the diagnosis and management of neovascular AMD. SD-OCT has the advantage of detecting small changes in the morphology of the retinal layers and subretinal space, allowing for precise anatomic detection of structural changes that may correspond to progression or regression of the neovascular lesions (13,14).
than 20,000 A-scans per second with an axial resolution of 3 to 7 µm in the eye (Fig. 13.1). At present, at least nine commercial spectral/Fourier domain OCT systems are available worldwide. SD-OCT has emerged as the ancillary examination of choice to assist the diagnosis and management of neovascular AMD. SD-OCT has the advantage of detecting small changes in the morphology of the retinal layers and subretinal space, allowing for precise anatomic detection of structural changes that may correspond to progression or regression of the neovascular lesions (13,14).
Several types of treatment directed at CNV have been developed to prevent the severe visual loss in neovascular AMD patients. These include laser photocoagulation (15), photodynamic therapy (PDT) (16), and the combination of PDT with intraocular injections of triamcinolone acetonide. Despite anatomical success in some cases, there is a low chance for visual improvement when these treatments are used. In recent years, research has provided new insights into the pathogenesis of macular disease. Today, less destructive treatments directly targeting the leakage produced by CNV and its pathogenic cascade have become available (17,18). Antibodies against vascular endothelial growth factor (VEGF) uniquely offer a significant chance to improve visual acuity in patients affected by neovascular AMD. Anti-VEGF therapy can provide long-term vision maintenance in over 90% and substantial visual improvement in 25% to 40% of patients (19,20).
Monitoring response to therapy is one of the most important clinical uses of OCT. OCT can be used to quantify changes in central retinal thickness, macular volume, and subretinal fluid. These parameters, in combination with visual acuity, are used to analyze the response to therapy in several diseases including neovascular AMD. Fung et al. (21) used OCT to guide the treatment of neovascular AMD and demonstrated that OCT could detect the earliest signs of recurrent fluid in the macula after the ranibizumab injections were stopped.
This chapter discusses the OCT findings in neovascular and dry AMD, the importance of the OCT on AMD management, and the choroidal changes observed with OCT.
OCT FINDINGS IN NEOVASCULAR AMD
FA remains the gold standard for initial diagnosis of CNV, the defining characteristic of neovascular AMD. Correlation between OCT findings and leakage and staining on FA has been demonstrated using both TD-OCT and SD-OCT systems (10,14). Small changes in the structure of the retinal layers and subretinal space can readily be noted allowing the detection of morphologic changes that may signal progression or regression of the lesions. As such, SD-OCT is a useful tool in diagnosing and monitoring anatomical changes associated with neovascular AMD.
Classic CNV on OCT can be seen as a highly reflective fibrovascular tissue with irregular yet defined borders between the RPE and Bruch’s membrane or above the RPE (22). Likewise, CNV may also appear as a localized fusiform serous or fibrovascular pigmented epithelial detachment (PED) (23). CNV not well defined by FA, or occult CNV, may present as an RPE elevation from the underlying choroidal plane and may be composed of either serous or fibrovascular tissue (23,24).
CNV, when active, is associated with presence of fluid, which appears on OCT as well-circumscribed hyporeflective spaces that distort the surrounding retinal architecture. The fluid can accumulate in the subretinal space, sub-RPE space, or between all layers of the inner retina, and it can be quantitatively evaluated with OCT (Fig. 13.2) (24,25). Recent studies show that SD-OCT is superior to TD-OCT in detecting subretinal, sub-RPE, and intraretinal fluid making it better for evaluation of CNV activity (26).
Freund et al. (27) proposed a more refined classification of CNV that is based on information from a variety of imaging techniques, such as combined FA/SD-OCT, high-speed SD-OCT, and indocyanine green (ICG) angiography, when necessary. This new classification system divides the neovascularization into three types with the goal of honing treatment strategies and providing better prognostic information.
Type 1 neovascularization is characterized by vessels proliferating under the RPE. With FA, it appears as occult or poorly defined CNV. With ICG angiography, it
presents as a low-intensity hyperfluorescence. On OCT, it appears as localized fusiform serous or fibrovascular PED (Fig. 13.2A). Type 1 neovascularization usually presents as a fairly mature neovascular tissue that may incompletely respond to anti-VEGF therapy (27). Polypoidal choroidal vasculopathy (PCV) is included in this classification as a variant of the type 1 neovascularization.
presents as a low-intensity hyperfluorescence. On OCT, it appears as localized fusiform serous or fibrovascular PED (Fig. 13.2A). Type 1 neovascularization usually presents as a fairly mature neovascular tissue that may incompletely respond to anti-VEGF therapy (27). Polypoidal choroidal vasculopathy (PCV) is included in this classification as a variant of the type 1 neovascularization.
Type 2 neovascularization is characterized by proliferation of neovascular tissue above the RPE, in the subretinal space. With FA, such CNV is described as classic. Using ICG angiography, it is usually more difficult to identify type 2 neovascularization due to the hyperfluorescence of the background choroidal circulation. SD-OCT localizes the vessels in between the RPE and the photoreceptor outer segments (Fig. 13.2B). Disruption of the inner/outer photoreceptor junction and intraretinal cystic spaces is often observed. Type 2 vessels may show a complete anatomic response to anti-VEGF therapy (27).
Type 3 neovascularization, commonly referred to as retinal angiomatous proliferation (RAP), is characterized by intraretinal neovascularization and has a distinct appearance on OCT. Imaging characteristics include sub-RPE CNV with intraretinal angiomatous change along with sub-retinal neovascularization and cystic change within the retina. These changes correlate with clinical and angiographic findings and are hypothesized to be caused by aberrant retinal-choroidal anastomosis (Fig. 13.2C and D) (28). Type 3 neovascularization appears to be sensitive to anti-VEGF therapy, if detected very early in its evolution (27).
Additional features of neovascular AMD are RPE tears or rips. They are known to occur in the setting of an RPE detachment both spontaneously as a feature of the disease and as the result of therapy. On OCT, a tear in the RPE is characterized by a focal defect in the RPE with scrolling at the borders along with pleating of the adjacent continuous RPE (Fig. 13.3). RPE rips are associated with the presence of a dome-shaped PED (29).
Additionally, end-stage AMD can be visualized as a disciform scar made up of hyperreflective tissue in the same location as the inciting CNV accompanied by retinal atrophy (24).
ANATOMIC ASSESSMENT OF THERAPEUTIC RESPONSE IN NEOVASCULAR AMD
Currently, inhibition of VEGF-A is the first-choice therapy for neovascular AMD. Such therapy often stabilizes the vision but can result in significant visual acuity improvement in about 1/3 of treated eyes. Two of the most effective preparations, bevacizumab (Avastin, Genentech Inc. South San Francisco, CA) and ranibizumab (Lucentis, Genentech Inc.), are recombinant monoclonal antibodies (Fab) that neutralize all biologically active forms of VEGF (18
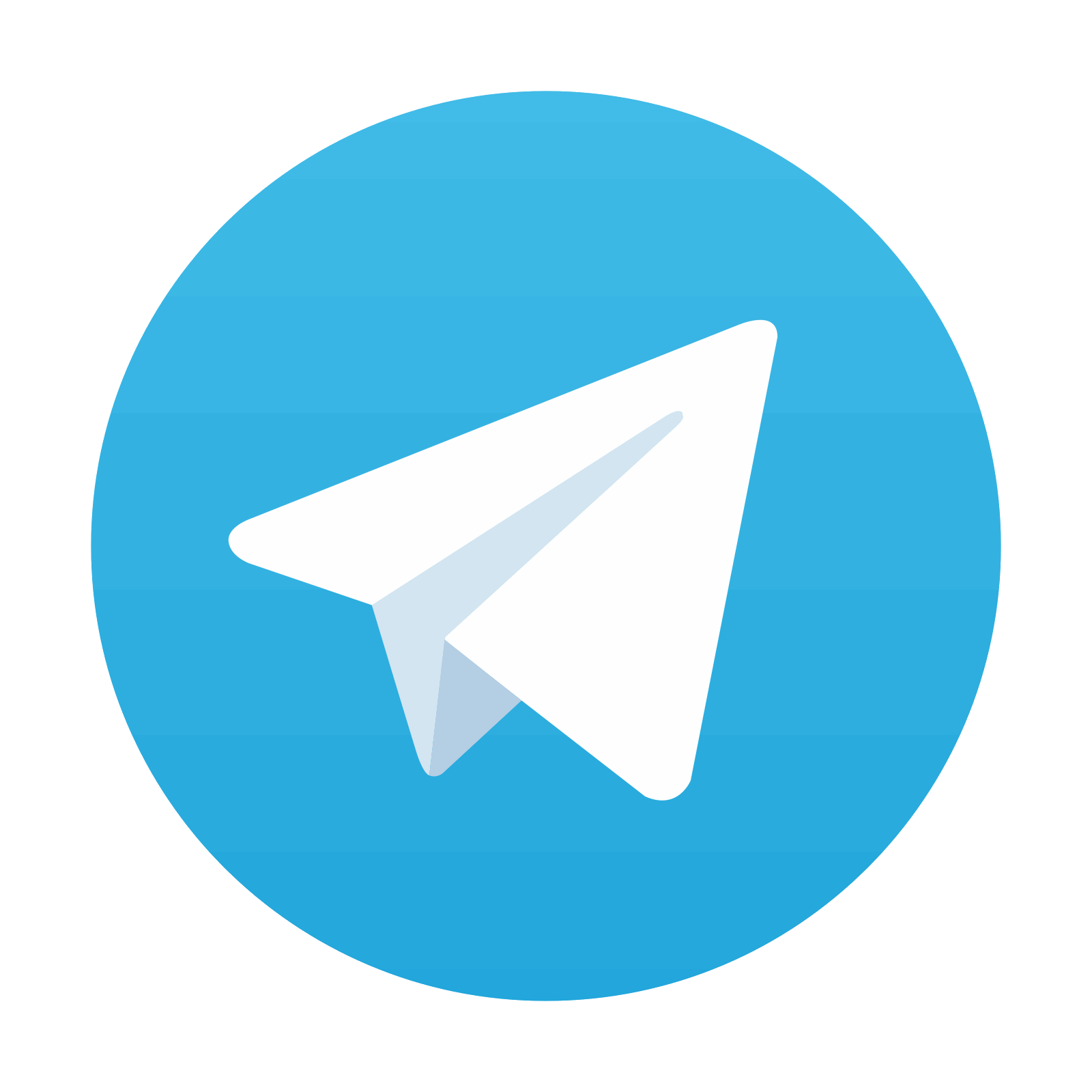
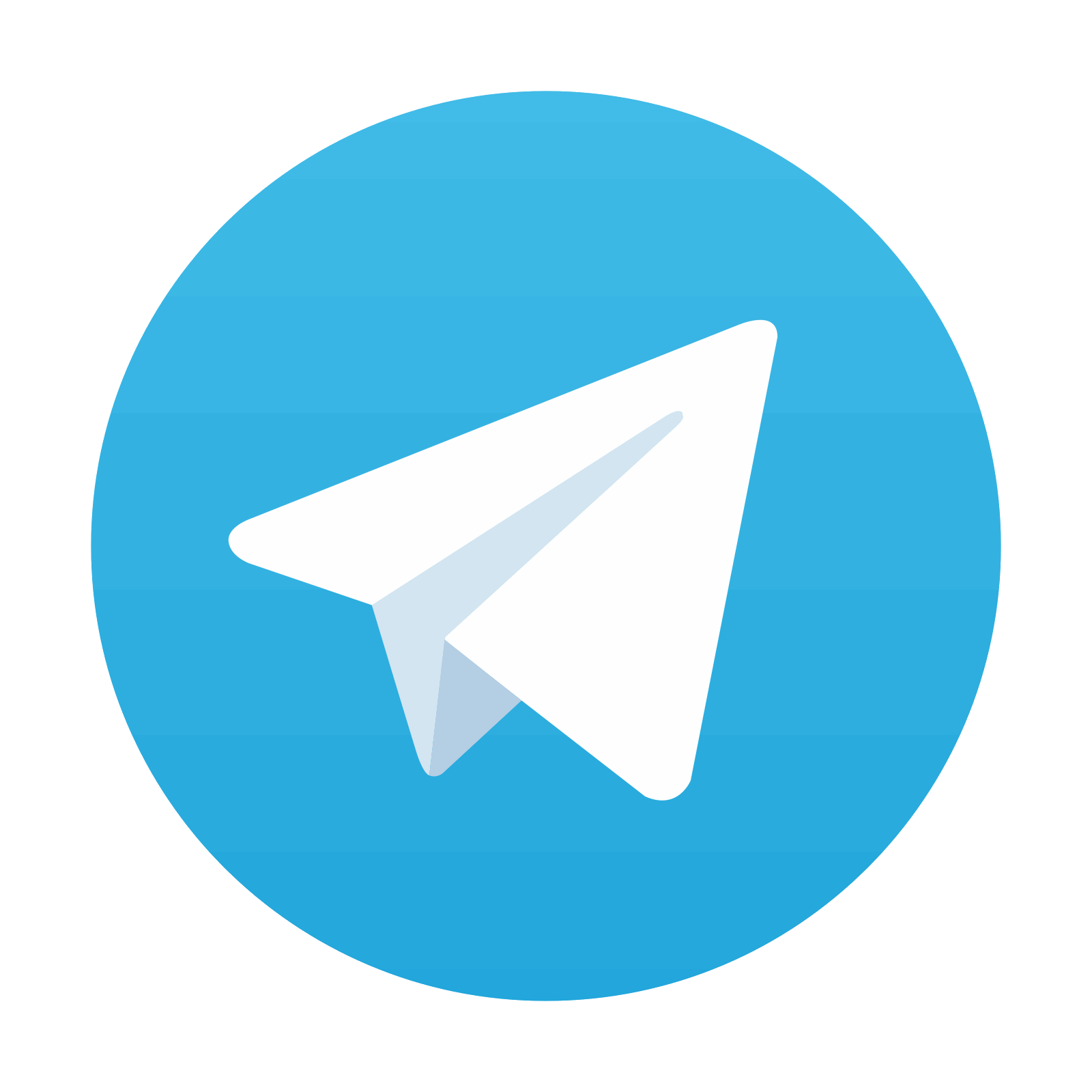
Stay updated, free articles. Join our Telegram channel

Full access? Get Clinical Tree
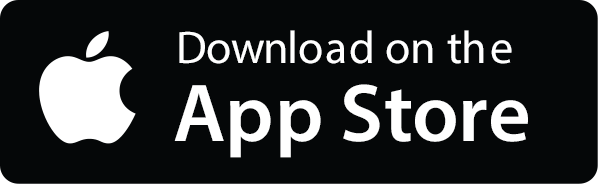
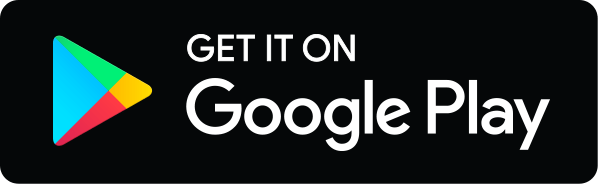