Nystagmus and Saccadic Intrusions and Oscillations
Louis F. Dell’Osso
Robert B. Daroff
Every truth passes through three stages before it is recognized. In the first it is ridiculed, in the second it is opposed, in the third it is regarded as self-evident.
Schopenauer
NYSTAGMUS
Nystagmus (derived from the Greek word, νμσταγμνσ, meaning drowsiness, is derived from νμσταζειν, meaning “to nod in one’s sleep”), the rhythmic to-and-fro oscillation of the eyes, has been regarded as enigmatic. In fact, the distinguished neuro-ophthalmologist Wilbrand once advised “never write on nystagmus, it will lead you nowhere.”1
Although technologic advances have permitted quantitative insights into nystagmus analysis, the clinician should not be daunted. Many useful, often diagnostic, observations can be made by physical examination alone. Figures 1 and 2 are examples of one convenient method of diagramming nystagmus. Also, nystagmus can be further described when the globes are inspected under slit-lamp magnification or when the fundus is viewed. However, due to the complexity of nystagmus waveforms and the possibility of combinations of different types of nystagmus, only ocular motility recordings can guarantee diagnosis that is both accurate and repeatable.
This chapter is a coalescence of the traditional neuro-ophthalmologic approach to nystagmus diagnosis and the impact of the newer capabilities of electronic eye movement recording and mathematical “biomodeling,” specifically, top-down, behavioral models capable of simulating human ocular motor responses to known target inputs in the presence of nystagmus and saccadic disorders.
Eye movement recordings have allowed definition of 49 types of nystagmus (Table 1) and new insights into their pathophysiology. For precise analysis, special recording techniques are necessary, such as infrared, magnetic search-coil, or high-speed digital video recording systems, which can faithfully reproduce the eye-movement trajectories and provide accurate information on eye position without drift or noise. For quantitative purposes, all systems should record by way of direct current, with a bandwidth of 100 Hz. The eyes should be recorded separately in horizontal, vertical, and (if possible) torsional directions, with the tracing analogs written on rectilinear graph paper. Recording should be performed during fixation of visible targets and sometimes in the dark with eyes open (see Chapter 9). For detailed quantitative analysis, the data should be digitized at 200 Hz or higher.
TABLE 1. Forty-nine Types of Nystagmus* | ||||||||||||||||||||||||||||||||||||||||||||||||||||||||||||||||||||||||||||||||||||||||||||||||||||||||||||||||||||||||||||||||||||
---|---|---|---|---|---|---|---|---|---|---|---|---|---|---|---|---|---|---|---|---|---|---|---|---|---|---|---|---|---|---|---|---|---|---|---|---|---|---|---|---|---|---|---|---|---|---|---|---|---|---|---|---|---|---|---|---|---|---|---|---|---|---|---|---|---|---|---|---|---|---|---|---|---|---|---|---|---|---|---|---|---|---|---|---|---|---|---|---|---|---|---|---|---|---|---|---|---|---|---|---|---|---|---|---|---|---|---|---|---|---|---|---|---|---|---|---|---|---|---|---|---|---|---|---|---|---|---|---|---|---|---|---|
|
Nystagmus has traditionally been divided into two types on the basis of the clinical impression of the waveform. Thus, if the eyes appeared to oscillate with equal speed in either direction, it was called “pendular” nystagmus; if movement in one direction was faster than in the other, it was called “jerk” nystagmus. True pendular nystagmus is sinusoidal, whereas jerk nystagmus has a slow phase away from the object of regard, followed by a fast (saccadic) phase toward the target. The direction of the fast component, by convention, defines the nystagmus direction. Only accurate recordings can often assess these criteria. Nystagmus should be described not only by its waveform and direction but also by its amplitude and frequency, the product of which is intensity. The examiner should also note the positions of gaze in which the nystagmus occurs and whether the intensity changes with gaze direction. Jerk nystagmus is usually accentuated in amplitude on gaze in the direction of the fast component, a characteristic referred to as Alexander’s law.2
The field of gaze in which nystagmus intensity is minimal is termed the null zone. The neutral zone is that eye position in which a reversal of direction of jerk nystagmus occurs and in which no nystagmus, any of several bidirectional waveforms, or pendular nystagmus is present. The null and neutral zones usually, but not always, overlap. Gaze-angle nulls usually result in head postures that allow use of the null to fixate targets that are directly in front of the patient.3
Based on quantitative eye-movement recordings, we identified three underlying mechanistic defects in the slow eye movement (SEM) subsystem (see Chapter 9) that produce nystagmus.
High gain instability. In some persons, because of abnormally high gain in the SEM subsystem, a runaway (increasing velocity) movement or a pendular oscillation is evoked. In this chapter, the term high gain can also imply excessive delay for the gain present (i.e., the control loop may have a normal gain, but an increased delay). Control theory suggests how particular changes in gain can result in either a pendular or a jerk nystagmus. Pendular nystagmus can be “congenital” (see section on Infantile Nystagmus Syndrome) or acquired, whereas horizontal jerk nystagmus with slow phases of increasing velocity usually is associated with congenital nystagmus; however, the latter may result from an Arnold-Chiari malformation.4 Vertical nystagmus with an exponential slow phase of increasing velocity may be secondary to acquired cerebellar disease.5
Visual-vestibular tone imbalance. The nystagmus of tone imbalance of the visual-vestibular subsystem results from the imposition of asymmetric input on an inherently normal horizontal gaze generator. This asymmetric input occurs if one vestibular apparatus (labyrinths, nerve, and brain stem nuclei) functions abnormally, if both sides are asymmetrically defective, or if there is a central imbalance of the optokinetic subsystem. The nystagmus recording always shows a linear (straight line) slow phase, reflecting a persistent tone to drive the eyes toward the side of the relatively damaged vestibular apparatus. The slow-phase amplitude is reduced by fixation and enhanced by darkness, Frenzel (high-plus) lenses, or closing the eyes. Fixation inhibition may be related to an opposing smooth-pursuit force and requires the integrity of the cerebellar flocculus.
Integrator leak. Nystagmus caused by a “leaky integrator” occurs only in an eccentric gaze position; thus, it is gaze evoked. The eyes are unable to maintain the eccentric position and drift back to the primary position with a decreasing velocity, reflecting a passive movement resisted by the viscous forces of orbital soft tissues. The defect may reside in the brain stem “neural integrator” or its connections, (such as in the cerebellum), which mediate eye deviation. This form of gaze-evoked nystagmus is called “gaze-paretic” nystagmus (see Chapter 9, Fig. 8 for an illustration of the gaze-paretic waveform).
One means of classification of nystagmus is based on whether it is a gaze-evoked or gaze-modulated type; the former category requires that there be no primary-position nystagmus. The nystagmus exhibited in two benign syndromes (infantile nystagmus and binocular maldevelopment nystagmus), physiologic types (vestibular), and symptomatic types (vestibular) fall in the gaze-modulated category. Some physiologic types (end-point) and symptomatic types (gaze-paretic) are gaze evoked. Although these concepts of a control mechanism represent useful approaches toward a more meaningful classification of nystagmus, they are far from inclusive. For practical reasons, an empirical nystagmus classification is presented that will aid the clinician in bedside and office evaluation, without the use of sophisticated recording instrumentation. This classification continues to change as our understanding of nystagmus advances. It must be emphasized, however, that such clinical diagnoses should be considered speculative and that definitive diagnosis is often only possible by means of accurate ocular motor recordings.
The localizing significance of nystagmus is often a mere indication of dysfunction somewhere in the posterior fossa (i.e., vestibular end-organ, brain stem, or cerebellum). However, certain nystagmus patterns are quite specific and permit reasonably accurate neuroanatomic diagnosis. When possible, the specific and nonspecific forms are separated on the basis of clinical appearance and associated signs and symptoms.
NYSTAGMUS IN INFANCY
There are several types of benign nystagmus usually seen in infancy. The characteristic types of nystagmus in the Infantile Nystagmus Syndrome (INS, fka “congenital” nystagmus—CN) are the most common. Others are the nystagmus of the Fusion Maldevelopment Nystagmus Syndrome (FMNS, fka latent/manifest latent nystagmus—LMLN) and the pendular nystagmus of the Spasmus Nutans Syndrome (SNS).6 We have adopted the nomenclature recommended by the Classification of Eye Movement Abnormalities and Strabismus (CEMAS) Working Group in an attempt to eliminate the confusing and misleading terminology of some of the classical names found in the literature. The new terminology differentiates between a syndrome that includes nystagmus (often several different types of nystagmus) and a specific type of nystagmus. For example, the nystagmus seen in the INS may be any combination of two or three mechanistically different types of nystagmus (see previous) resulting in 12 to 14 specific waveforms. Using this terminology facilitates more accurate descriptions of each type of nystagmus when required (e.g., pendular, pursuit-system nystagmus) while still allowing for the inclusion of several types found in each syndrome by simply appending the word nystagmus to the syndrome (e.g., INS nystagmus). Similarly, if the shorthand, “IN” is used, it too must be understood to be a general description encompassing all of the specific types of nystagmus possible in the INS; the same applies to “FMN.”
Infantile Nystagmus Syndrome
The nystagmus of the INS is usually present at birth or noted in early infancy at the time of development of visual fixation, and it persists throughout life. The syndrome consists of one or more types of nystagmus with characteristic waveforms, head turns, tilts, or oscillations. Rarely, the nystagmus becomes manifest later in life,7 so the term congenital should be thought of as a congenital predisposition for this particular type of ocular motor instability, rather than taken literally. This syndrome may accompany primary visual defects, which led to the assumption that the nystagmus is secondary to poor vision and that both “sensory defect” and “motor defect” types existed. In fact, eye-movement recordings demonstrated that the specific types of nystagmus found in the INS had the same waveforms and underlying mechanism, regardless of the coincidental, perhaps facilitating, existence of a sensory deficit. The nystagmus itself is the direct result of an ocular motor control instability that may develop with or without an accompanying sensory deficit. Thus, for those cases in which a sensory deficit exists, it can only be a subordinate factor in the development of the nystagmus, perhaps interfering with the normal calibration of one or more of the ocular motor subsystems, thereby precipitating instability. The common association of “pendular” nystagmus with a sensory defect and “jerk” nystagmus with a primary motor abnormality was both simplistic and erroneous. Ocular motor studies of infants with INS showed no difference in waveforms associated with the presence or absence of sensory deficits; the infants exhibited the same waveforms that have been recorded in children and adults.8,9,10 Specifically, the development of foveation periods in INS waveforms begins early in infancy as acuity and fixation develop. This is clearly seen in infrared recordings of infants when they are attending to a visual task. INS may appear spontaneously or be familial. Hereditary INS may be sex linked, recessive, or dominant; the dominant form has been linked with chromosome 6p12.11
The relationship of the visual defect to the nystagmus possibly represents simple genetic association. Although the visual problem is not causal, it may contribute to the intensity of the nystagmus. At least one form of INS nystagmus represents a high-gain instability in a SEM subsystem,12 and fixation attempt (the effort to see) is its main driving force. Poor vision will increase fixation effort and increase the intensity of the nystagmus. Moreover, a subclinical motor instability may become manifest by this exaggerated visual effort. Although the exact anatomical location of the source of the instability present in INS nystagmus is unknown, we hypothesize that the various pendular waveforms (and some jerk waveforms) are due to a gain/delay problem in an internal (brain stem) feedback loop in the pursuit subsystem.12 That is, the pendular nystagmus waveforms of INS stem from “pursuit-system” nystagmus, modified by the saccadic system’s attempts to foveate the target and the fixation subsystem’s attempt to extend foveation; this hypothesis is embodied in an ocular motor system model (see Chapter 9).13,14,15,16 The much greater frequency of horizontal–torsional nystagmus, compared with vertical or diagonal nystagmus, probably reflects inherent differences in the stability of the respective pursuit subsystems (i.e., the horizontal is more unstable than the vertical). Although there is no torsional smooth pursuit system per se, the torsional component of the nystagmus reflects instability in torsional control.17 Another factor in support of the hypothesis of pursuit-system nystagmus is that no oscillopsia is perceived from oscillations in pursuit velocity, not in normals and not in those with INS. Thus, no additional adaptation mechanism need be proposed to account for the absence of oscillopsia in INS; it is suppressed by the same mechanism by which normals suppress it during pursuit. Initially, we proposed that excessive positive feedback around the common neural integrator might be responsible for the accelerating slow phases of INS nystagmus.18 We subsequently demonstrated that the common neural integrator is not the site of the INS instability.19 However, several models have been proposed that attempt to explain the genesis of some INS waveforms, based on that premise.20,21,22 Although each can generate limited, specific INS waveforms, the models exhibit behaviors inconsistent with data from individuals with INS and do not simulate the known broad range of human ocular motor responses (both normal and during nystagmus) to common stimuli (i.e., they are demonstrations of putative mechanisms to generate waveforms rather than models capable of simulating ocular motor system behavior). Because IN appears to be activated and intensified by fixation attempt, the deficit may also be linked to the fixation subsystem (see Chapter 9). The coexistence of a high-frequency pendular oscillation with a low-frequency jerk nystagmus (resulting in a dual-jerk waveform) in some INS subjects and also in FMNS, suggests that the high-frequency pendular oscillation is due to an instability at a different site. Recent evidence points to the nucleus of the optic tract (NOT) as the site of this oscillation shared by patients with either the INS or FMNS.23 Goldstein suggested that IN is caused by oscillations at two frequencies whose interactions may approximate some of the known INS waveforms.24 However, such interactions do not produce the absolutely motionless (i.e., “flat”) periods of extended foveation (300–400 msec) recorded in many patients.
Distinguishing the lower frequency pendular nystagmus from jerk nystagmus may be difficult clinically, particularly in the INS. Certain forms of jerk nystagmus are invariably mislabeled as pendular, or the direction is misidentified. Even with oculographic recordings, the direction of the fast phase may be misinterpreted unless velocity tracings are obtained.25 In the absence of oculography, clinicians should describe the nystagmus carefully or use diagrammatic methods (see Figs. 1, 2, and 3). Monocular visual deprivation induced, in some monkeys, a diagonal nystagmus whose horizontal component initially looked like FMNS slow phases (see later discussion) and then developed to resemble INS slow phases. This deprivation took place from birth to 25 days and was followed by monocular deprivation of the other eye.26 The role of the NOT in FMN in monkeys has been more clearly defined recently.27,28
The INS nystagmus usually damps significantly with convergence. Although the exact mechanism responsible for this damping in unknown, there was speculation that it might result from co-contraction of antagonist muscles of each eye during convergence. However, recent work by Miller found no co-contraction.29 We hypothesized that damping during convergence might result from an effective increase in the stiffness of the ocular motor plant brought about by the increased innervation to the two medial recti. (i.e., co-contraction of antagonist muscles of the two eyes, rather than of each eye). The Orbit 1.8 simulation (J. M. Miller, personal communication), predicted that the 8 g primary-position tension in the medial rectus increased to 13 g at 20° adduction (40° of convergence) and to 18 g at 30° adduction (60° of convergence), 75% and 125% increases, respectively. Because convergence results in a change in the muscle pulley system,30,31 the latter may be the mechanism by which the stiffness is increased. The observations of convergence-induced damping of other types of nystagmus support this peripheral mechanism in preference to one relying on an inherent property of the nystagmus. As previously mentioned, the intensity of INS nystagmus is related to the fixation attempt, which probably explains why it sometimes persists with eyes open in darkness (when the subject will probably attempt to “see”) and damps behind closed lids (when the subject will, unless instructed to the contrary, reduce any attempt to “see”).25 The defining criterion is fixation attempt, not retinal illumination or lid position. Therefore, reports of the presence or absence of nystagmus with lid closure or darkness that lack a description of the instructions to the subject, provide little useful information.
The recognition of INS is of extreme importance, particularly in the adult patient, and may obviate unnecessary neurodiagnostic procedures; its characteristics are listed in Table 2. INS is almost always binocular and never shows more than minor amplitude dissociation between the two eyes. Clinically, the nystagmus usually appears uniplanar. Like vestibular end-organ nystagmus, horizontal nystagmus remains horizontal when the eyes are deviated vertically and does not convert to vertical nystagmus. Using new, sensitive techniques for recording torsional eye movements, we found small but significant torsional components in the nystagmus of subjects previously thought to have purely horizontal INS.17 Because the prominent horizontal movement masks the usually smaller torsional component, the latter appears to be a common characteristic of “horizontal” INS. In most patients, rightward movements were accompanied by clockwise torsion and leftward movements by counterclockwise torsion.32 We discuss later the superimposition of a latent component on an ongoing IN.
TABLE 2. Characteristics of Infantile Nystagmus | |||||||||||||
---|---|---|---|---|---|---|---|---|---|---|---|---|---|
|
Eye-movement recordings of INS occasionally show a pure pendular waveform (sinusoidal) or a saw-toothed waveform (equiamplitude linear slow phase with foveating saccade) (see Fig. 8) typically seen in vestibular nystagmus. These pure forms are neither frequent nor pathognomonic for INS. More often, INS manifests distinctive waveforms that are not present in acquired nystagmus. These waveforms are an expression of the attempts by the ocular motor control system to increase foveation time, imposed on inherently unstable slow control. The INS waveforms shown in Figs. 4, 5, 6 and 7 (other than pure pendular or jerk) have never been recorded in acquired horizontal nystagmus.8,33 The target position is indicated by a dashed line; target position is problematic for pure and asymmetric pendular waveforms. We have regrouped the original waveforms based on hypothesized mechanisms to emphasize the different types of nystagmus that may be recorded in an individual with INS. This resulted in differentiating linear slow phases from the more common accelerating slow phases of jerk nystagmus, based on presumed mechanisms (visual-vestibular and pursuit-system, respectively). The presence of dynamic overshoots in the fast phases (saccades) of IN waveforms is both idiosyncratic and variable; for simplicity, we have not included them in Figs. 4, 5, 6 and 7. Because dynamic overshoots are not an abnormality, their presence does not represent a “different” waveform. Using our computer model,14,16 we demonstrated how the pendular waveforms in Figure 4 could be generated from a pursuit-system instability, and we are investigating two possible sources for the unidirectional jerk waveforms in Figure 5. We hypothesize that the linear-slow-phase jerk waveforms in Figure 6 arise from a tonic imbalance in the visual-vestibular subsystem. When the low-amplitude, high-frequency pendular oscillations, hypothesized to arise in the NOT, are present, they are superimposed on the basic IN waveforms as shown in Figure 7. Of the 15 waveforms shown in Figs. 4, 5, 6 and 7, we hypothesize that nine are due to pursuit-system oscillation (Figs. 4, 5), three to visual-vestibular imbalance (Fig. 6), and the three in Figure 7 to NOT nystagmus added to either type. We have also recorded the high-frequency NOT nystagmus superimposed on a low-frequency pendular IN. Twelve of these waveforms are pathognomonic of IN; P, AP, and JL are not. One important feature of IN waveforms is the presence of “braking saccades” that act to stop runaway slow phases.34 They are triggered by extraretinal eye-velocity information and, when properly measured, have the same velocity and duration characteristics as other types of saccades.35,36 For pendular waveforms, the target is foveated at the peaks that are more flattened, indicating extended foveation. Extended foveation in an adult with lifelong nystagmus secondary to a congenital brain stem hamartoma and in an adult given gabapentin for treatment of nystagmus secondary to an arteriovenous malformation37 supports the hypothesis that extended foveation periods in INS waveforms represent the action of a normal fixation system on the underlying INS oscillation. Figures 8 and 9 demonstrate how these waveforms serve to increase the time of foveal imaging.
![]() Fig. 4 Variations of pendular nystagmus due to pursuit-system instability: pure (P), asymmetric (AP), pendular with foveating saccades (PFS), pseudopendular (PP), and pseudopendular with foveating saccades (PPFS). Note that although foveating saccades vary in amplitude, all achieve foveation (i.e., they all return the eyes to same point, the target). Foveation takes place at the peaks that are more flattened; here shown as the leftmost peaks. In this and Figures 5, 6, 7 and Figure 16, dashed lines indicate target position (see text). In all figures, upward deflections indicate rightward, upward, or clockwise eye movement (all eye movements are from the patient’s perspective). |
![]() Fig. 7 Dual jerk nystagmus hypothesized to be comprised of NOT nystagmus superimposed on IN waveforms shown in Figures 5 and 6. The resulting waveforms show sinusoidal modulation of the underlying slow eye movement off target. Depicted are dual jerk right with accelerating slow phases (DJRA), dual jerk right with extended foveation (DJREF), and dual jerk right with linear slow phases (DJRL). |
![]() Fig. 8 Foveation “strategy” employed during pendular (P) and jerk left (JL) nystagmus. The target is only briefly foveated at points 0, 2, and so forth. t, time scale. |
The pure pendular (P) and jerk (J) waveforms in Figure 8 are not conducive to good acuity because of the extremely short foveation time (instants 0 and 2 on the time axis). Although these are common acquired waveforms, when afflicted with INS, the developing nervous system modifies pendular and jerk waveforms; therefore, foveation time (and thus acuity) is increased. Examples of some resultant waveforms are shown in Figure 9. In the pendular nystagmus with foveating saccades waveform (PFS), there is usually a substantial period of time when the target is imaged on the fovea and the eye is motionless (instant 3 on the time axis). In jerk-right nystagmus with extended foveation (JREF), the position from time 0 to 1 is when foveation takes place, and in the bidirectional jerk-left (BDJL) waveform, the position from instants 4 to 5 is conducive to good acuity. Waveform, gaze-angle nulls, and convergence nulls are affected by heredity.38 Members of the same family show more specific combinations of waveforms or of either waveform, having only a convergence null or no convergence null (i.e., having only a gaze-angle null), than do members of the general INS population. We found greater damping of INS nystagmus with convergence than with gaze angle, in patients who exhibited both types of null, and this translated into acuity increases.39 Comparison of the results of the Anderson-Kestenbaum and artificial divergence procedures also favored the latter.40
Increased foveation time is the most effective determinant of increased acuity.41,42,43,44 In most INS subjects, the best waveform (i.e., most foveation time per cycle) is in the null region associated with a particular gaze or convergence angle, but in other subjects it is not; these latter subjects prefer the gaze or convergence angle that yields the best waveform, even if it is not the waveform with the least amplitude. Decreased amplitude is, however, the major determining factor in cosmetic improvement and is appreciated by a young patient’s parents. Despite a nulling of the nystagmus, an individual with INS may not show an increase in acuity with convergence if the resulting waveform has little foveation time per cycle or if acuity is primarily limited by a visual deficit. The fixation system of someone with INS is able to repeatedly foveate a target within minutes of arc, almost as accurately as a normal person.39,42,45 The use of phase-plane analysis allows definition of a “foveation window” (±0.5° by ±4.0°/second) for the study of fixation, smooth pursuit and the vestibulo-ocular reflex (VOR).39,46,47 These studies demonstrate the extremely accurate fixation, pursuit, and VOR possible in individuals with INS. In Figure 10, the tight overlap of foveation periods within the foveation window in the phase plane demonstrates how accurate the cycle-to-cycle target foveation in IN can be. The foveation window defines the time when the eye is within ±0.5° of the target and moving with less than ±4°/sec. Thus, despite an ocular oscillation whose amplitude is well outside the foveal extent and whose velocity exceeds ±30°/sec, the ocular motor system can foveate the target with extreme accuracy and allow normal visual acuity.
The nystagmus acuity function (NAF) provides an objective determination of potential visual acuity from measurements of the key characteristics of the INS waveform: foveation time and the standard deviations of foveation position and velocity means (for NAF) or position mean alone (NAFP).48 For those subjects whose foveation ability is not well developed (i.e., the target image always falls within the default foveation window), the window used for its calculation can be enlarged and the expanded NAF (NAFX) plotted versus gaze or convergence angle. Software is now available that calculates the NAFX from eye-movement data and provides a quantitative method for evaluating different therapies for their affect on potential visual acuity.49 Plots of the NAF or NAFX versus visual acuity reveal the linear relationship that allows intersubject prediction of potential visual acuity. The NAFX can also be used to compare potential acuity across subjects with different types of nystagmus (INS or FMNS) or to predict the acuity increase possible after therapeutic intervention in a given subject. The latter is accomplished by plotting the NAFX versus gaze or convergence angle. Figure 11A shows the NAFX outputs during near and far fixation from a subject with achiasma, recorded in Laboratory of H. Collewijn in Rotterdam (see Albinism and Achiasma section following).50 As the NAFX clearly shows, conditions for highest visual acuity occurred during near fixation where (as the bottom panels show) a smaller foveation window could be used to calculate the NAFX. Figure 11B shows the pre- and post-tenotomy NAFX outputs from an achiasmatic Belgian sheepdog, demonstrating the effectiveness of the treatment. As the bottom panels show, the post-tenotomy nystagmus is subclinical and well within the boundary of the area centralis; the nystagmus during the whole interval qualified as a foveation period. Figure 11C shows the NAFX versus potential (solid and dashed lines) and measured (symbols for the human data) visual acuity for two humans (S1 and S2) and a canine (S3). The NAFX (and, potential visual acuity) is higher at near than at far (S1 and S2) or while using base-out prisms at far (S1). For the canine, the post-tenotomy NAFX is higher than preoperatively, and the dashed line is inferred from veterinary data. Because of its direct relationship to potential visual acuity, the NAFX is the best available outcome measure of therapies designed to affect the IN waveform. Although amplitude is related to cosmetic appearance, neither it nor frequency is closely correlated with acuity.
![]() Fig. 11 (A) The expanded nystagmus acuity function (NAFX) output for a subject with achiasma during far and near fixation, demonstrating improvement at near by the smaller foveation window and lower variation of foveation position. (B) The NAFX for an achiasmatic Belgian sheepdog demonstrating dramatic postoperative improvement by the relative sizes of the nystagmus and the ±3° centralis window. (C) NAFX versus visual acuity for both humans and canines, demonstrating improvement at near fixation (humans S1 and S2), at far with base-out prisms (S1), and post-tenotomy (canine S3). In this and Figures 12, 13, and 18B, the dot-dashed lines indicate the extent of the foveal window (humans) or area centralis (canines). In this and Figure 12, the thickened areas identify foveation (human) or centralis (canine) periods. |
We hypothesized a new type of surgery to damp the IN of subjects that do not have gaze-angle, convergence or static nulls (i.e., they have asymmetric (a)periodic alternating nystagmus—APAN) or do have a primary-position null.51 The surgery consists of a simple tenotomy, dissection, and suture of the involved extraocular muscles in place, with neither recession nor resection. Results of a masked clinical trial of this surgery were positive in a phase 1 study of adults.52,53 The putative mechanism responsible for the damping effects of tenotomy is alteration of a proprioceptive tension-control loop.51 This is supported by the discoveries of the neural substrate for such a loop in both the musculotendon54,55,56,57 and enthesial end of the tendon (where the surgery takes place).58 Before any surgery is contemplated, IN should be definitively diagnosed using ocular motility recordings.59 Figure 12 shows the improvement in NAFX output of a patient after four-muscle tenotomy. As the increased NAFX values indicate, tenotomy damped the IN and improved the waveform. Both the foveation time per cycle and the mean foveation position improved and the post-tenotomy foveation window was smaller in both position and velocity. The various therapies available for INS, based on the presence or absence of gaze and convergence nulls, is summarized in Table 3. Note that for patients with both convergence and gaze-angle nulls, exploitation of the former (surgically or with vergence prisms) usually damps the nystagmus and increases acuity most; it is necessary to add -1.00 S (OU) to vergence prisms for prepresbyopic patients. Preliminary studies (at all gaze angles) of the broad damping effects of convergence on IN (see Fig. 14 and discussion of convergence following) suggest that composite prisms (unequal base-out) are not necessary in these cases; vergence prisms (equal base-out) will achieve the same damping.60 As indicated in Table 3, regardless of the presence of nulls, afferent stimulation can be used in all patients who exhibit nystagmus damping with active stimulation (see later discussion).
TABLE 3. Therapies for Infantile Nystagmus | |||||||||||||||||
---|---|---|---|---|---|---|---|---|---|---|---|---|---|---|---|---|---|
|
The so-called inversion of the optokinetic reflex seems to occur only with INS.61 When optokinetic stimuli are presented to a patient with INS, a peculiar phenomenon may occur: the resulting nystagmus may be opposite in direction from what would be anticipated if the evoked optokinetic nystagmus (OKN) simply summated with the ongoing nystagmus. For example, in the presence of left-beating INS nystagmus, the response to right-going optokinetic targets (a leftward fast phase) should add to the left-beating INS nystagmus to produce enhancement of the nystagmus intensity. In “inversion,” the nystagmus may either damp or be converted to right-beating nystagmus. If right-going targets are presented at a gaze angle at which the nystagmus is either absent or pendular, a right-beating nystagmus may result. Inversion of the optokinetic reflex is present in 67% of INS patients. The observation of optokinetic inversion establishes the nystagmus as IN. The phenomenon is, in reality, merely a reversal of the INS nystagmus direction due to a null shift; it is not a true inversion of the optokinetic response (see discussion of reversed pursuit later). The basic function of the optokinetic system is to stabilize slowly moving retinal images, but the rapidly moving retina of an INS patient may interfere with this function. The optokinetic response appears suppressed in some patients; however, the perceived circularvection is in the proper direction, and OKN dynamics appear to be normal in individuals with INS.
The head oscillations that often accompany INS increase with visual intent and have traditionally been regarded as compensatory. For compensation to be achieved, head movements would have to be equal in amplitude and opposite in direction to the eye movements. For such a mechanism to work, the VOR would have to be totally inhibited (gain reduced to 0). Accurate objective observations of the head movements in patients with INS do not support that hypothesis.62 Rather, the head oscillation is merely an extension of the motor instability, and the VOR functions normally to cancel the effects of head oscillation during the periods of target foveation normally present in the INS waveform.47 The head tremor in INS can be distinguished from that in acquired disease; it is easily suppressed voluntarily in the former but not in the latter.
Point out the head tremor to the patient. If it stops, the nystagmus is IN; if it persists, both are acquired.
Individuals with INS usually do not experience an illusory oscillatory movement of their environment (oscillopsia).63,64 This lack of oscillopsia in INS, and also in FMNS, suggests that both oscillations occur within an efference copy feedback loop that serves to nullify the effects of retinal-image oscillation induced by either of these instabilities.65 Like most ocular oscillations (myoclonus being the exception), INS nystagmus disappears in sleep. In two patients with INS plus an acquired nystagmus, their acquired oscillopsia seemed to be related to an inability to maintain repeatable periods of good foveation in a particular plane.66,67 However, that inability was an epiphenomenon caused by the addition of a transitory acquired nystagmus to the ever-present INS nystagmus.65 Oscillopsia suppression in INS and other types of nystagmus appears to be accomplished by efference copy of the nystagmus signal.65,68,69,70,71,72 Oscillopsia may occur in some patients with very poor foveation stability73 or may occur in later life secondary to afferent deficits.74 The thresholds for motion detection in INS differ from normal and may also play a role in oscillopsia suppression.75,76 We reported oscillopsia of a migraine aura in an individual with INS,77 as well as vertical oscillopsia secondary to a decompensated phoria; the latter event led to the discovery of subclinical see-saw nystagmus in the horizontal–torsional waveforms of IN.78 As Figure 13 shows, the subclinical see-saw nystagmus is phase locked with the horizontal and torsional components allowing foveation periods to occur in all planes simultaneously. Torsional motion does not seem to impair orientation perception thresholds, perhaps due to extraretinal information.79 We hypothesize that subclinical see-saw nystagmus results from slight mismatches between the forces exerted by the vertical recti and oblique muscles and does not represent a true vertical-system instability; this contrasts with the see-saw nystagmus accompanying achiasma (discussed later).
![]() Fig. 13 Tri-planar recording of a typical IN with a PPFS waveform showing the relative size and phase relationship of the subclinical see-saw component of IN to the more prominent horizontal and torsional components. Note the correspondence of the foveation periods in all planes. In this and Figures 18 and 19, heavier lines indicate right-eye motion. |
During fixation of stationary targets, many individuals with INS have a permanent null region representing the gaze angle at which the intensity of the nystagmus is the lowest and the waveform most conducive to highest NAFX and acuity. They often turn their heads to permit straight-ahead viewing with the eyes in the null region. Such patients benefit from appropriate version prism spectacles that alleviate the necessity for the head turn and the resulting increased fixation attempt.25,41 However, as Figure 14 shows, if the IN damps with convergence, the higher NAFX values will persist over a broader range of gaze angles than during fixation on a far target. This allows higher acuity over most useful gaze angles and demonstrates the advantage of either base-out prisms or the bimedial rectus recession procedure over therapies aimed at moving a gaze-angle null to primary position.
Some INS patients may exhibit a superimposed latent component that induces null shifts toward an eye that is covered (Fig. 15).80 Demonstration of such a shift and maintenance of any of the INS waveforms establish the nystagmus as belonging to INS rather than the FMNS (see later discussion). Rarely, a null shift is toward the viewing eye.25
Some studies of INS and smooth pursuit have led to confusion between the reversal of INS direction that may occur during pursuit and reversed pursuit. This confusion is similar to that discussed earlier for the optokinetic response. Accurate eye-movement recordings show that neither the optokinetic nor the pursuit subsystem responds in a reversed manner, as should be obvious both by the absence of any symptoms of such a grave deficit and the normal abilities of individuals with INS in sports. Also, their perceptions of both the direction and magnitude of movements in the periphery and on the fovea are normal. Just as the INS waveform is distorted by SEM (creating periods of extended foveation) during fixation of a stationary target, the pursuit system is able to generate pursuit movements with a direction and velocity that match those of a moving target during these same periods of the INS waveform.46,68,81 This ensures extended foveation of the moving target and results in accurate smooth pursuit during the periods when the target image is on the fovea. Pursuit during foveation is all that is necessary for good acuity; the same conditions are met during smooth pursuit as are met during fixation of a stationary target. During smooth pursuit (or during optokinetic or VOR stimuli) the gaze angle at which the INS null region occurs shifts in the direction opposite to the pursuit (optokinetic grating or VOR-induced eye motion).46,47 The amount of null shift is a function of the pursuit or VOR velocity. This measurable shift in the INS null angle causes the INS nystagmus reversal that has been mistakenly equated with “reversed” responses of both the optokinetic and pursuit subsystems.
In many individuals with INS, afferent stimulation of the ophthalmic division of the trigeminal nerve or of the neck may damp the nystagmus and improve the waveform, allowing increased visual acuity.48,82 Neck or forehead vibration prolonged foveation periods, yielding higher values of the NAF and improved visual acuity in 9 of 13 patients with INS.48 This noninvasive and benign therapy (active afferent stimulation) may prove useful in both INS and acquired nystagmus. The use of soft contact lenses to improve the acuity of individuals with INS takes advantage of the damping effect on nystagmus of (passive) afferent stimulation.83,84,85,86
Soft contact lenses are not contraindicated in INS and can provide better acuity than spectacles in patients whose nystagmus damps with afferent stimulation. Plano soft contact lenses can be used if no refractive correction is required.
Fusion Maldevelopment Nystagmus Syndrome
The FMNS consists of: a jerk nystagmus with either a linear or decreasing-velocity exponential slow phase identical to that of gaze-paretic nystagmus; strabismus; alternating hyperphoria/dissociated vertical deviation; and pendular torsional nystagmus in primary position. Occasionally, when both eyes are closed, a jerk nystagmus with a linear slow phase is present. Classically, the nystagmus evoked by the “pure” or “true” latent condition (LN) occurs only with uniocular viewing. There is no nystagmus with both eyes viewing, but when one eye is occluded, nystagmus develops in both eyes, with the fast phase toward the uncovered eye (Fig. 16). The fast phases of FMN may contain dynamic overshoots,90 whose characteristics in both FMN and IN are normal.91 Dynamic overshoots are integral parts of the saccadic fast phases and not the beginnings of the slow phases. The occurrence of square-wave jerks (SWJ, see following) is also normal, and their presence during binocular viewing in FMN is unpredictable and variable. Thus, the presence of either does not represent a different “type” of FMN;92 it is merely the same FMN including these common, normal saccadic dynamics or intrusions. This also applies to the occurrence of other types of nystagmus in addition to FMN (e.g., an undefined torsional nystagmus or the high-frequency pendular nystagmus thought to arise from the NOT, resulting in a dual-jerk waveform). In an individual patient, both FMN and IN may coexist with another type of nystagmus or saccadic intrusion/oscillation. The clearest way to delineate a group of patients with two mechanistically independent conditions is to describe them as having a syndrome consisting of two conditions (e.g., FMN with dynamic overshoots, FMN plus SWJ, or FMN plus torsional nystagmus). For simplicity, the basic FMN waveforms shown in Figure 16 do not contain dynamic overshoots in their fast phases nor the confounding addition of other types of nystagmus. When the additional pendular oscillation of NOT nystagmus is present, the resulting waveform would be the same as shown in Figure 7 for linear slow phases or consist of a pendular oscillation superimposed on decelerating slow phases.38 FMN is always congenital. However, several cases have been recorded of the manifest form of FMN (MLN occurring with both eyes open), associated with retrolental fibroplasia.90 FMN is common in Down syndrome, where it may coexist with IN.93
Early theories postulated that a unilateral retinal stimulus was the necessary condition for FMN, but this concept was discounted by observations of FMN in monocular fixation with a blind eye or with an acoustic stimulus in complete darkness. Similarly, the hypothesis that the FMNS is caused by nasal-temporal asymmetries in the optokinetic reflex is not supported by evidence that subjects with FMNS are able to use retinal slip information to adapt motion-detection sensitivities94 and are able to pursue symmetrically.95 Also, because nasal-temporal asymmetries exist in individuals with strabismus but not FMN,94 this cannot be the primary causal factor in the genesis of the nystagmus. Asymmetries in the monocular optokinetic response of monkeys deprived of binocular input early in life may result from, rather than cause, their nystagmus. In normal monkeys, each nucleus of the optic tract (NOT) is driven binocularly; in these monkeys, they are driven by the contralateral eye.96 Although the resulting imbalance may provide the tonic signal that produces the FMNS slow phases (inactivation of the NOT with muscimol abolishes the nystagmus), the cause of the imbalance appears to lie in higher centers. The spontaneous reversal of FMN in the dark has led to the speculation that eye dominance is predetermined.97,98 Shallo-Hoffmann et al. identified an alternating vertical component to FMN,99 and Brodsky linked the genesis of FMN and dissociated vertical divergence to the dorsal light reflex present in many animals.100
We relate the FMNS to the cortical switching that must occur in the calculation of egocentric direction when going from binocular to monocular viewing.90 Under binocular conditions, the gaze angle of each eye is summed with the other and divided by two to obtain the egocentric direction, referenced to the “cyclopean eye.” However, with monocular viewing, egocentric direction depends only on the viewing eye, and the cortical operation of summing and dividing by two must be altered to process unchanged information from the viewing eye. The shift in egocentric direction toward the nonviewing eye causes the slow drift of the eyes in that direction. Both eyes are then corrected by a saccade in the direction of the viewing eye, which brings the eyes to the target (or, in darkness, to the intended gaze angle). This contention is supported by unilateral strabismus surgery causing central effects on egocentric localization.101 Thus, FMNS nystagmus may be generated by this inability to properly alter the cortical mathematical operation normally used to define egocentric direction (i.e., this deficit in higher centers may result in a tonic imbalance in the visual-vestibular subsystem, producing the linear slow phases of FMN).
The shift to monocular egocentric localization can also produce a mode whereby the saccadic system generates defoveating saccades that momentarily carry the fixating eye past the target in a temporal direction, followed by a decelerating-velocity nasal drift back toward the target.102 This results from generating a pulse, but not a step, of innervation to drive the fast phases of the FMNS nystagmus. Presumably, the common neural integrator is kept from integrating these defoveating pulses by the signal representing the correct eye position vis-à-vis the target. These hypotheses have been combined in an ocular motor system model (see Chapter 9) capable of simulating responses of an individual with FMNS.15,103,104
FMNS nystagmus occurs in patients with strabismus who, although viewing with both eyes open, are fixing monocularly. The slow phases are either linear or decelerating, and the fast phases are always in the direction of the viewing eye.90 The nystagmus of patients with strabismus, alternating fixation, and FMNS nystagmus with both eyes open has fast phases always in the direction of the fixating eye. Such patients may be easily misdiagnosed as having INS, because the nystagmus is present with both eyes open. Recordings are required to document the decelerating or linear slow-phase waveforms characteristic of FMNS from the accelerating slow phases predominant in the INS.
Strabismus is a necessary (but not sufficient) condition for FMN.105 That is, all individuals with FMN have strabismus, consisting of a phoria under cover and a tropia with both eyes open, if nystagmus is present under these respective conditions. Conversely, FMNS is not significantly associated with early-onset strabismus.106 Rarely, on occlusion of a preferred eye, during which fixation with an amblyopic eye is forced, both eyes drift in the direction of the covered eye without corrections by fast phases; this is called latent deviation. Early surgical correction of infantile strabismus may convert the nystagmus of FMNS present with both eyes open (the manifest condition) to nystagmus present only upon occlusion of one eye (the latent condition),107 thereby supporting a previous hypothesis.105
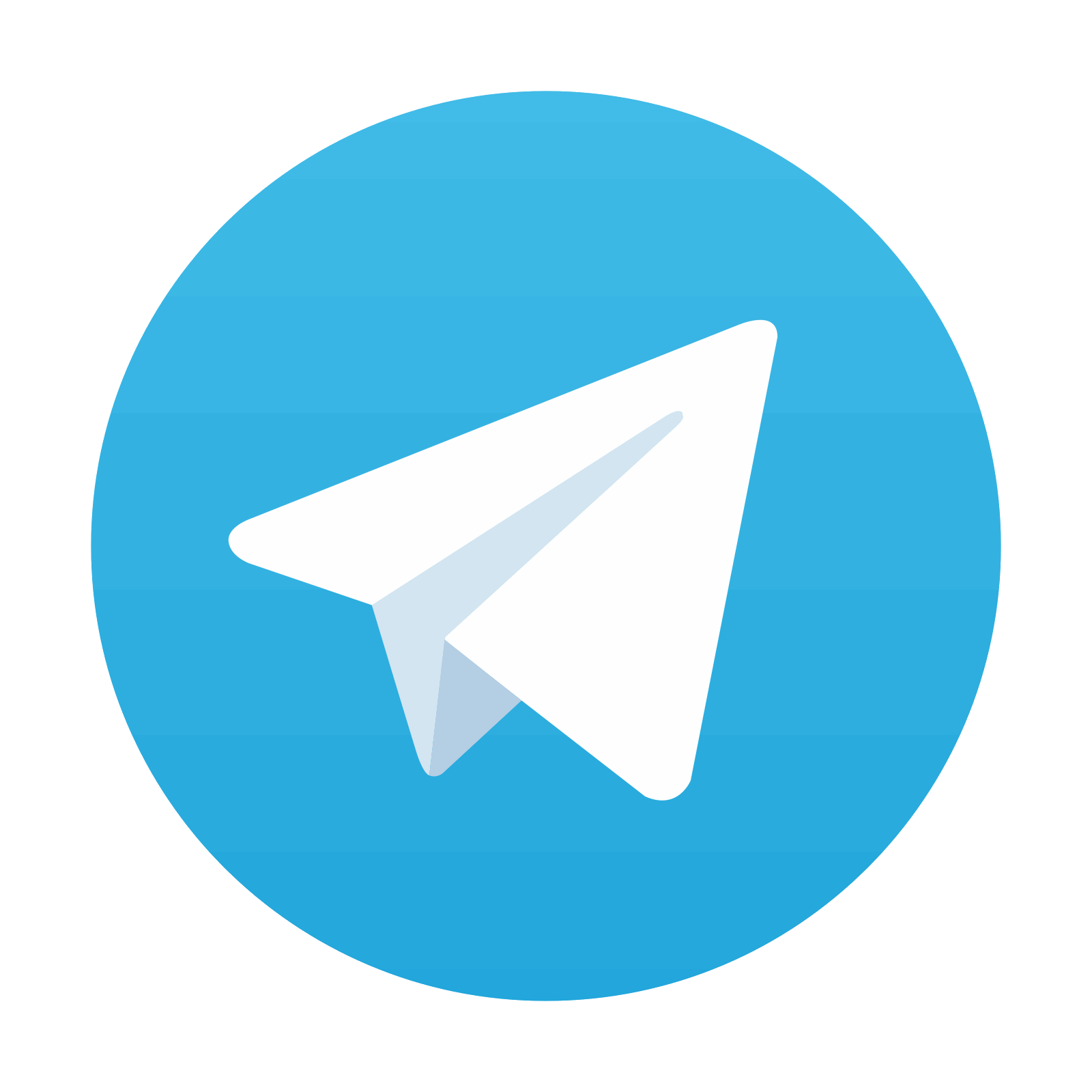
Stay updated, free articles. Join our Telegram channel

Full access? Get Clinical Tree
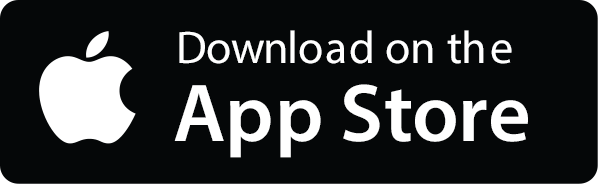
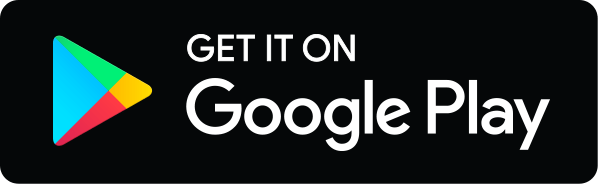
