5 Christophe Baudouin The tear film is the second line of ocular surface defense after the lids. The volume of tear film is 7 to 9 μL, and the basal secretion rate is 1 to 2 μL/minute.1 The accurate thickness of the tear film, however, remains under discussion, ranging between 7 and 40 μ according to various authors.2,3 Until recently, tear film has usually been considered to be composed of three distinct layers—mucus, aqueous, and lipid—but in fact the tear film mostly resembles a gellike structure with a gradient of mucus concentration from the superficial epithelial cells, where it tightly adheres, by the glycocalyx, to the outer layers, less concentrated in soluble mucins and covered with the lipid layer that limits tear evaporation. Tears are cleared partly by evaporation but mainly by the nasolacrimal ducts. The mucus layer thickness is a matter of substantial controversy, ranging between 2 and 40 μm depending on the author, the technique used, and whether the mucin layer is considered as integrated with the aqueous layer or as a whole separated from the overlying aqueous layer.3,5 Mucins have high molecular weight (2000 to 40,000 kd) and are heavily glycosylated glycoproteins.6 Soluble and gelforming mucins are secreted by goblet cells throughout the conjunctiva. Mucins play a major role by rendering the corneal surface hydrophilic, instead of its naturally hydrophobic state; in addition, mucins lower the surface tension of tears. Above a concentration of 1%, tears form a high-viscosity gel with non-newtonian properties. Other mucins are membrane-bound and participate in the mucus layer of the tear film adhering to the epithelial surface. Adherence of tear film to the ocular surface is also increased by the microvilli and invaginations of the epithelial membranes covered with the glycocalyx, which participates in tear film stability by making the epithelial surface wettable. The glycocalyx therefore interacts with soluble and gelforming mucins, especially MUC5AC, which is secreted by goblet cells and other soluble mucins such as MUC2 and MUC7, a soluble mucin secreted by the lacrimal gland. Nineteen genes have been identified, from MUC1 to MUC17 (with two forms of MUC3 and MUC5), eight of which are known to be associated with the ocular surface or lacrimal glands. The membrane-associated mucins MUC1, MUC4, and MUC16 have been found in conjunctival and corneal epithelia.6 Some membrane-bound mucins may also be found in a soluble form in the tear film. In addition to their role in cell surface wettability and a likely role as a pathogen barrier, some membrane-associated mucins, especially MUC4, have epidermal growth factor (EGF)-like domains that may directly influence epithelial growth and regulation.7 The soluble mucin MUC7 also has a histatin-like domain that may have antifungal properties.8 The aqueous layer is the thickest layer of the tear film. It is mostly composed of water (98%) and mucins, but it also contains electrolytes, growth factors (among them EGF plays a critical role in corneal epithelium physiology and wound healing), cytokines, immunoglobulins, and a contingent of desquamated and inflammatory cells. Basal secretion is ensured by Krause and Wolfring accessory lacrimal glands, whereas reflex secretion and most proteins are provided by the main lacrimal glands. This notion has been challenged by some who think that all aqueous tear secretion is neurally driven and that both the main and accessory glands have similar functions. These proteins play a major role in defending the ocular surface against microbial agents through the secretion of lysozyme, beta-lysin, lactoferrin, IgA, IgG, and serum proteins filtered throughout the acini of the lacrimal glands.1 Tear proteins also play an important role in tear viscosity. The lipid-binding proteins lipocalins, a family of proteins secreted by lacrimal glands, together with lactoferrin and secretory IgA, have been shown at their tear physiological concentrations to form a high-viscosity non-newtonian gel.9 The low surface tension of tears would therefore be the result of tear proteins and possibly of some lipids complexed with tear lipocalins, at least as much as that of the only soluble mucins.4,5 It has thus been demonstrated that nonmeibomian lipids are present in high concentrations in the aqueous layer of the tear film and that their removal increases the surface tension of tears.10 A higher surface tension, as encountered in dry eye disease, results in tear film instability and is therefore an important parameter causing impairment of ocular surface cells. In addition, lipocalins could act as scavengers toward contaminating lipids that may make the corneal surface hydrophobic and prone to desiccation. This protective effect of lipocalins has been postulated as a continuous phenomenon regulated by lacrimal glands.5 The lipid layer is produced mainly by the meibomian glands, holocrine-type glands embedded in the tarsal plates. Its thickness varies between 1 and 100 nm, but most studies estimate it at levels close to 90 to 100 nm.4 Using the meibometry technique, it has been calculated that the total amount of meibomian secretion would reach 300 μg in the normal marginal reservoir and 9 μg in the preocular film.11,12 Meibomian oil is expressed during blinking and is spread on the tear film between each blinking. The thickness and stability of tear film depend on the expression capability of meibomian glands. Meibum is a complex mixture of different lipidic compounds that may vary greatly from one subject to another.4 It is composed mainly of wax esters (13 to 35%), sterol esters (8 to 34%), triglycerides (4 to 43%), free fatty acids (2 to 24%), polar lipids (0 to 16%), and hydrocarbons assumed to be of environmental origin. A high proportion of cholesterol esters would be a suitable substrate for commensal microorganisms and may constitute a risk for development of meibomian gland dysfunction (MGD).13 Two distinct layers, that is, polar and nonpolar lipids mainly composed of phospholipids, make up the outer layer of the tear film and play a key role in limiting evaporation between two blinks. The model proposed by Holly4 suggests that nonpolar lipids spread onto a surface of polar lipids. The lipid layer may also interact with lipids bound to lipocalins in the aqueous phase. Lipocalins secreted by the lacrimal glands also participate in the stability of tear film by preventing penetration of cutaneous lipids that disrupt tear film stability.5,9,14 The tear film responds to very delicate regulatory mechanisms, notably involving neuroregulatory phenomena and hormonal stimulation.15 Each layer of the tear film plays a major role in the physiology of the ocular surface. The aqueous layer hydrates the cornea, prevents it from keratinization, and thus from opacification, and vehicles major defense proteins. The mucus layer allows the tear film to spread on the epithelial cell surface and participates in eliminating foreign bodies and microorganisms. In addition to the barrier function of all three layers of the tear film, microorganisms may efficiently be prevented from accessing ocular surface cells by antibacterial or antifungal properties of some mucins (especially MUC7), tear proteins, and even meibomian lipids.4 Both mucus and lipid layers participate in tear film stability, as mucins play a role in tear film adherence to epithelial cells and the superficial lipid layer limits tear evaporation. The complete removal of lipids from the tear film or meibomian gland obstruction may considerably increase the evaporation rate and tear osmolarity.4 Any abnormality of either tear components will thus cause tear film instability and hyperosmolarity. In addition to protection against desiccation and penetration of microorganisms, the tear film also participates in the refractive power of the cornea, by smoothing the epithelial surface and facilitating the healing process of the cornea and the penetration of oxygen into the cornea. Tear film also contains antioxidant enzymes that may protect ocular surface cells from oxidative stress. Any defective compound may severely affect tear film stability and ocular surface homoeostasis. Although the causes of dry eye are multifactorial, they can be related to deficiencies in any one of the components of the ocular surface and tear film. Despite their wide use in clinical practice, standard tests for assessing dry eye disease and ocular surface disorders—including symptom recording, tear breakup time, meibomian gland testing, corneal staining, and Schirmer’s testing—have shown only poor repeatability16 and are thus only weakly reliable. Moreover, it is well known that subjective symptoms often do not correlate with objective signs. Many additional exploratory techniques have therefore been developed to objectively investigate the tear film and the ocular surface clinically in a noninvasive or minimally invasive way. Some of them are easily available to the clinician, whereas others remain research procedures and require further validation but could constitute in the near future a battery of reliable methods for clinical investigations of ocular surface disorders. The main compartment of tear film resides in the tear meniscus. Attempts have thus been made to measure tear meniscus height as a useful representation of tear film volume, which is deeply decreased in aqueous-deficient dry eyes. In contrast with Schirmer’s 1 test, which also measures a contingent of reflex tears, tear meniscus height relates to the steady-state volume of tears. Photographs of tear meniscus may be used for measuring its height, radius, or width, but these procedures require fluorescein instillation17 or a specular reflection device. A videomeniscometer has been developed18,19 that projects black and white stripes and records in real time the meniscus behavior over time. The meniscus radius can then be calculated from sequential images. At the center of the lower lid margin, the meniscus radius was found to be highly significantly decreased in dry eyes (0.17 ± 0.05 mm) compared with normal eyes (0.30 ± 0.1 mm) and more than restored after punctual plugging (0.57 ± 0.23 mm). A cutoff value of 0.25 mm was proposed by the authors, with a sensitivity and specificity of 88.9% and 77.8%, respectively (see review in Yokoi and Komuro19). Interestingly, this technique was also used for evaluating the tear turnover of eyedrops instilled in the cul-de-sac. The retention time of various tear substitutes could be easily compared using this procedure, which could thus provide interesting pharmacological applications in the future. Fluorophotometry of the tear film was first developed as early as 1963 by David Maurice. The technique was further improved by using a highly sensitive automated fluorophotometer (Fluorotron Master®, Coherent Radiation, Inc., CA).20 Tear volume and turnover may be calculated after applying one drop of fluorescein and collecting a tear specimen. Eter and Gobbels recently proposed a noncontact technique of tear fluorophotometry by establishing a calibration line with various concentrations of fluorescein in a test chamber.21 This made it possible to determine tear volume and turnover without taking a tear sample. The decay of tear fluorescein after instillation of 1 μL of 2% fluorescein is recorded over a period up to 30 minutes and has been shown to follow a biphasic curve that may allow calculation of the tear turnover rate.22 In most studies in the literature,22 the normal tear flow averages 1 μL/minute, with a tear turnover rate between 15 and 22%/minute, and it decreases in dry eyes at values between 0.10 and 0.55 μL/minute and 6 to 8%/minute, respectively, with higher rates in meibomian gland diseases than in aqueous-deficient eyes. Despite the great potential interest of fluorophotometry in ocular surface diseases, the concentration of fluorescein administered, the time chosen for sequential measurements after instillation, the influence of examination duration, and the lack of definitively standardized methods for fluorophotometry calibration may severely affect the results from one investigator to another, so that this technique has not yet become a standard for dry eye investigations. Meibomian gland secretions are intrinsic components of the tear film and play a major role in stabilizing it between blinks. MGD is an important cause of tear film instability and one of the principal mechanisms of evaporation in dry eye disease. Meibographic techniques have been developed to assess the morphology of meibomian glands and their content. A transillumination probe is used for this purpose and can identify partial or total loss of the visible structure of meibomian glands, also called meibomian gland dropout (Table 5-1). Meibomian gland destruction is the hallmark of MGD, but meibography may be useful in other ocular surface diseases to assess meibomian gland patterns. It was shown that significant meibomian gland dropout occurred in about 58% of eyes with Sjögren’s syndrome.23 In addition, meibometry can quantify the lipid layer volume. Different techniques have been described for collecting and analyzing lipid levels at the lid margin and lipid delivery rates. Lipid sampling is obtained using an 8-mm-or a 20-mm-wide loop of translucent plastic tape applied for 3 to 10 seconds to the central third of the lower lid margin, with the eye in upgaze. The tape is air-dried, and optical density is measured with a meibometer.19,24 Improvements have been proposed for lipid collection and analysis.24 A laser-based technique has also recently been proposed by Komuro et al.25 This technique seemed to correlate well with the amount of lipids in meibomian secretions and the degree of expressibility of the glands, the meibometric values being increased in aqueous-deficient dry eyes and decreased in MGD.24,25 Calculations made with a meibometer in healthy subjects showed that meibomian secretions along the lid margins reached approximately 300 μg and constituted a large reservoir for the preocular lipid layer of the tear film, which is expected to be 9 μm in volume.12
Nonroutine Tests for Dry Eye Disease
Key Points
♦ The Tear Film
Composition
Main Roles of the Tear Film
♦ Clinical Assessment of Ocular Surface and Tear Film
Assessment of Tear Volume and Secretion
Tear Meniscus Height
Tear Fluorophotometry
Evaluation Techniques of the Lipid Layer
Meibography and Meibometry
Grade 0 | No meibomian gland dropout |
Grade 1 | Meibomian gland dropout in less than half of the inferior tarsus |
Grade 2 | Meibomian gland dropout in more than half of the inferior tarsus |
Data from Shimazaki J, Goto E, Ono M, Shimmura S, Tsubota K. Meibomian gland dysfunction in patients with Sjogren syndrome. Ophthalmology 1998;105:1485–1488.
Interferometry and Interface Patterns
Interference patterns, generated by specular reflection of cold light (to limit warmth-induced evaporation) on the lipid layer of the preocular tear film, provide interesting information concerning lipid layer thickness, spreading, and stability. Different devices have been developed (Tearscope Plus®, Keeler Instruments, Broomall, PA; DR-1®, Kowa Co., Ltd., Torrance, CA) to investigate interference patterns.26,27 Several classifications have been proposed. Yokoi et al28 proposed five grades: grade 1 shows a grayish uniform distribution of the lipid precorneal layer; grade 2 shows a grayish color with a nonuniform distribution; grade 3 shows nonuniform distribution of color interferences; grade 4 shows many colors with a nonuniform distribution; and grade 5 shows an absence of lipid layer and areas of exposed cornea. Normal tear films are mainly grade 1 or 2, whereas dry eyes are grades 2 to 5, with a possible overlap of grade 2 tear film layers between normal and dry eyes.19 The classification proposed by Guillon et al,26,29 using the Tearscope Plus®, is slightly different and has been upgraded to 10 distinct levels (Table 5-2). High grades were found predominantly in newborns and infants, indicating a high lipid thickness, whereas adults were mainly distributed in the lower grades.29 A major limit to these techniques, however, is the wide range of theoretically normal patterns, as 85%, of normal eyes in adults were distributed between grades 2 and 7, indicating that abnormal patterns are only at the extremes of such classifications, that is, either absence of lipids or the greatest thickness with globular patterns (Fig. 5-1).
Improvements in interference analysis techniques have been proposed by using video recording of tear spreading time and patterns30,31 or by providing computer-assisted interference color charts with respect to lipid layer thickness.32 Dynamic investigations of the tear film lipid layer after blinking showed that complete stabilization followed a horizontal propagation pattern and took less than 1 second in normal eyes. In MGD or aqueous-deficient dry eyes, time to reach a stable tear film was much longer, several seconds or more, and the propagation pattern was vertical and uneven, with a thicker lipid layer at the inferior cornea and a much thinner, most likely deficient layer in the superior cornea.32
Level 0 | Lipid absent |
Level 1 | Open meshwork |
Level 2 | Detailed meshwork |
Level 3 | Closed meshwork |
Level 4 | Meshwork-wave combination |
Level 5 | Wave |
Level 6 | Wave-amorphous combination |
Level 7 | Amorphous |
Level 8 | Amorphous-color combination |
Level 9 | Color |
Data from Isenberg SJ, Del Signore M, Chen A, Wei J, Guillon JP. The lipid layer and stability of the preocular tear film in newborns and infants. Ophthalmology 2003;110:1408–1411.
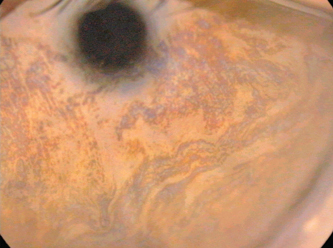
All these techniques are useful for noninvasively assessing the preocular tear film, especially the lipid component, its thickness with qualitative or semiquantitative assessments, its dynamics immediately after blinking, and its stability over time. However, a great overlap between normal patterns and those found in mild dry eyes may limit their discriminative potential and prevent interference-based methods from definitively becoming the reference techniques for practical assessment of dry eye disease.
Evaporation Rate Measurements
Evaporation of the tear film is assumed to result from the stability and composition of the lipid layer and possibly from the corneal epithelium, but it also depends on many environmental and individual factors, such as interpalpebral surface, eye position, hygrometry, light conditions, and temperature. Animal species and age also show major variations. In particular, young infants blink much less than adults, most likely because of a better antievaporative function of the lacrimal film.33 Noninvasive breakup time and lipid layer thickness assessed by the technique of interference fringe biomicroscopy were thus found to be much longer and thicker in 3- to 6-month-old infants than in adults.29 Different devices and procedures were described, but the main principle is to measure humidity over time with an evaporimeter in a chamber made up of tight-fitting goggles containing known water content and temperature. Most researchers have tried to standardize measurements, especially the evaporation from the skin, by using petroleum jelly34 or by subtracting evaporation from closed eyelids.33,35 The latter studies gave evaporation rates as a function of relative humidity, and values throughout the literature vary between 4 and 15 × 10-7 g · cm-2 · second-1 in normal eyes.33 Conflicting results were found when comparing normal evaporation rates and those found in dry eye disease, with values ranging between 7 and 59 g · cm-2 · second-1.22 Most studies thus showed increased evaporation rates in dry eye disease,36 together with hyperosmolarity and a greater temperature, decrease after blinking.37 MGD consistently caused a very high evaporation rate compared with normal eyes and tear-deficient dry eye, but values calculated from one study to another were not fully consistent and showed wide ranges of calculated evaporation rates. Nevertheless, evaporation rates correlated well with meibomian gland dropout and patients associating aqueous-deficient dry eye and MGD had the highest evaporation rates.38 Moreover, from these studies, it appears that the proportion of tear volume lost by evaporation averages 10 to 15%, although rates up to 30% were also calculated by other groups. These rates increase more than 50% and even 70% of the total tear secretion in dry eyes, irrespective of their mechanisms.22
An indirect method for evaluating evaporation rates uses thermography. Corneal temperature is warmer just after blinking and rapidly decreases in temperature when eyes are opened. These subtle changes can be recorded using new-generation high-speed infrared radiation thermography, which can collect up to 60 values per second and calculate a temperature-decrease coefficient. Using this technique, patients with dry eye showed smaller temperature changes after blinking than did patients with normal eyes.39 Thermography could thus become an interesting technique for better evaluating tear film impairment.
A relationship between temperature and inflammation of the ocular surface was also suggested, with increased temperatures in dry eyes recorded with infrared thermography.40 Examination conditions, however, have to be standardized for all these functional evaluations of tear film (constant room temperature, humidity, and brightness; fixed eye position), which may make their widespread use difficult for most clinicians.
Optical Assessment of the Ocular Surface
Videokeratography
One major role of the preocular tear film is to smooth the corneal surface and give an optimally regular air-cornea interface. It has been shown that any irregularity in the tear film—resulting either from corneal drying spots, increased tear film thickness, or irregular surface of the tear film between blinking—may severely affect optical quality of the eye and cause blurry vision in dry eye disease.41 Videokeratography techniques have shown that major indices of the corneal surface, namely the surface regularity index (SRI) and the surface asymmetry index (SAI) are deeply impaired in dry eye disease. Specific software that analyzes these indices every second for 10 seconds greatly improved sensitivity of tear film stability assessment.42 This technique required topical anesthesia so that patients could keep their eyes open for 10 seconds, but the drop was found not to influence tear stability results significantly.43 Another similar adjustment of videotopographic measurements using successive recording of SRI and SAI four times per second for 15 seconds also provided a means to follow the buildup and breakup of tear film after blinking over time.44 Optimal resurfacing of tear film took a few seconds, and indices then decreased in most, but not all, cases when progressively approaching the breakup time. Because videokeratography is a rather easily accessible technique for a large number of clinicians, available in most ophthalmic centers, this procedure could prove to be a promising simple and noninvasive technique for time-wise kinetic evaluations of the tear film.
Wavefront Analysis
The recent development of wavefront analysis using Hartmann-Shack-type aberrometers also has provided a better understanding of the nature of optical impairment resulting from a poor tear film. Tear breakup was found to cause high-order optical aberrations.45 Dry eyes also showed significant increases in total aberrations (root mean square; RMS) and high-order aberrations, especially coma-like and spherical-like aberrations, both for scotopic and photopic vision, in dry eyes compared with normal eyes.46 This technique may make it possible to better investigate and quantify the optical impairment that many patients with dry eye complain of, even in the absence of superficial keratitis, as tear film instability may also cause optical aberrations.
Morphological Changes of Ocular Surface Cells
New-generation in vivo techniques of confocal microscopy (Confoscan®, Nidek, Japan; or Corneal Module of the HRT-II®, Heidelberg Engineering) can now explore the cornea, limbus, and conjunctiva at a cell-level resolution. Superficial epithelia are easily differentiated (Fig. 5-2), and inflammatory cell infiltrates or goblet cells can also be investigated by these totally painless and almost noninvasive techniques, although they require contact between the probe and the ocular surface. Keratoconjunctivitis sicca (KCS) showed dramatic changes in corneal epithelium morphology, with enlargement of epithelial cells and increased reflectance of nuclei, which took on a conjunctival-like pattern, together with mild to intense dendritic cell infiltration of the conjunctiva and the peripheral cornea. Goblet cells have mostly disappeared in these cases, but in some cases limbal stem cell dysfunction may be recognized by the ingrowth of conjunctival epithelial cells and goblet cells within the corneal area. A spectacular correlation between in vivo confocal microscopy images and histopatho-logical features from corneal or conjunctival imprints was demonstrated. These devices will therefore become major tools in the near future for minimally invasive ocular surface investigations.
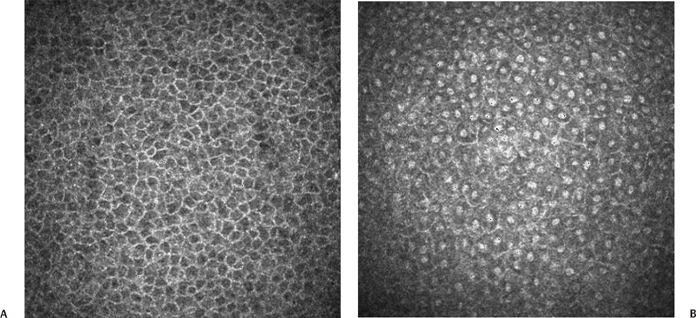
Figure 5-2 In vivo confocal microscopy using the HRT-II® corneal module, showing a normal corneal epithelium (A) and a metaplastic corneal epithelium in a case of keratoconjunctivitis sicca (B).
♦ Conjunctival Impression Cytology
Cytological Procedures
General Principles
Developed at the end of the 1970s,47,48 impression cytology is now a well-known, easily repeatable technique for collecting conjunctival epithelial cells in a noninvasive or minimally invasive, rapid, and almost painless way for biological analyses of ocular surface disorders. Using conjunctival impression, the most superficial cells of the conjunctival epithelium, that is, cells undergoing desquamation, are collected. The conjunctival epithelium renews itself from the basal layers, and the most superficial cells, which have reached their final differentiation, are progressively evacuated into the lacrimal fluid. Conjunctival impression enables collection of the cells located in this superficial layer and therefore allows analysis of the cells having reached their final differentiation.
At this level, three main populations of conjunctival cells can be found in impression cytology specimens—epithelial cells, goblet cells, and inflammatory cells. Until now, epithelial cells were considered as participating only in the outer lining of conjunctival mucosa, but it has now been widely shown that they are directly implicated in numerous biological reactions, especially in conjunctival inflammatory or apoptotic pathways.49–51 Goblet cells release soluble mucins in the lacrimal film and thus play major defense and regulatory roles in the ocular surface. Lack of goblet cells is the hallmark of dry eye disease,52 whereas the presence of goblet cells in impressions obtained from the corneal surface is pathognomonic of conjunctivalization associated with stem cell deficiency.53
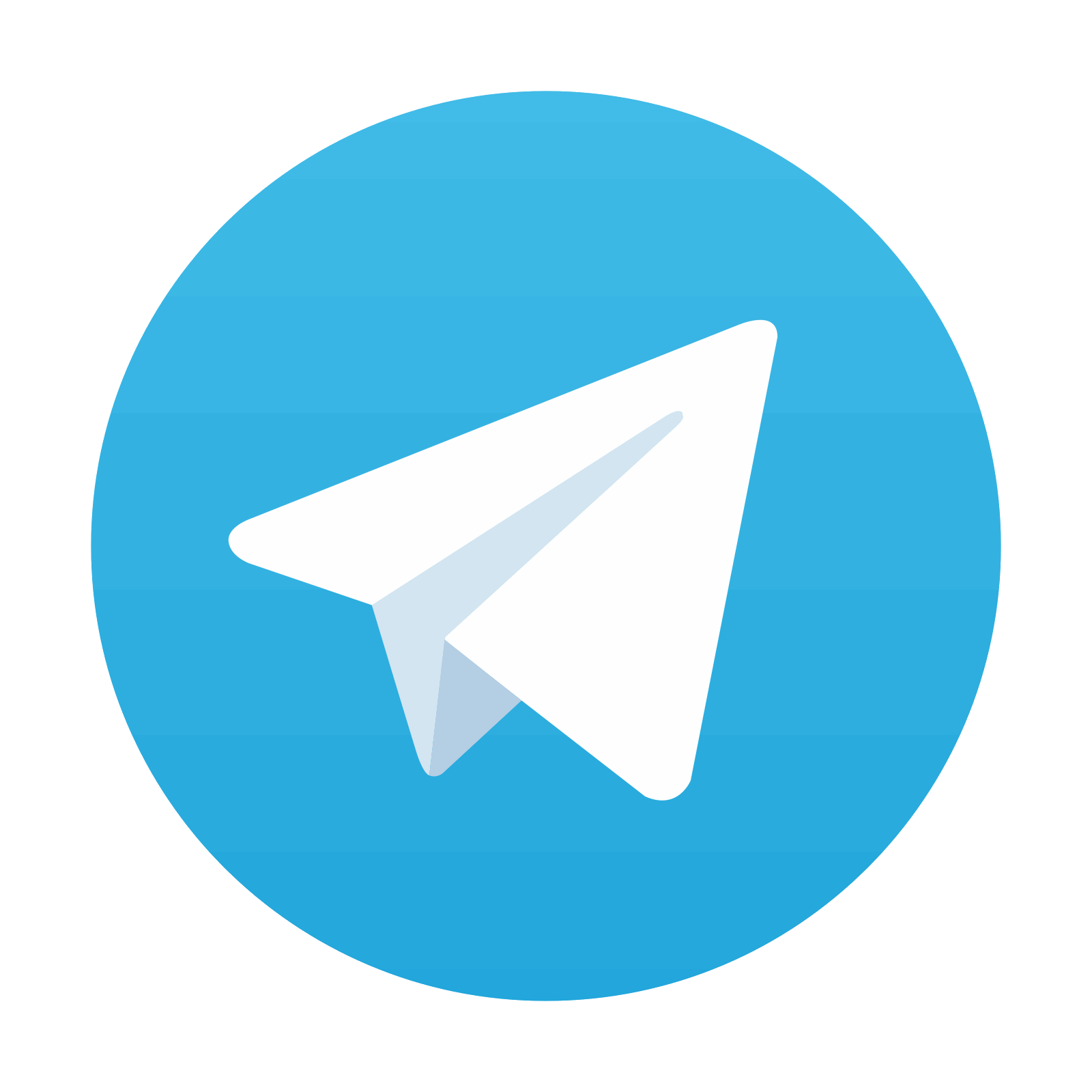
Stay updated, free articles. Join our Telegram channel

Full access? Get Clinical Tree
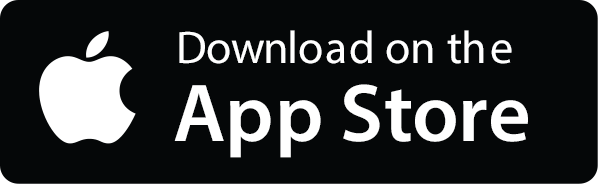
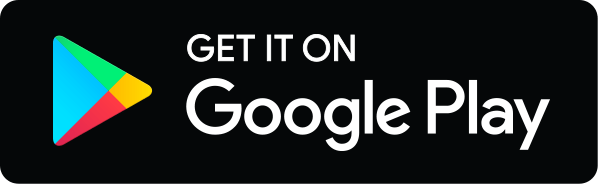