Medical Treatment of Cataract
Peter F. Kador
J. Samuel Zigler Jr.
John I. Clark
Manuel B. Datiles
Current estimates1 show that in the United States the direct government medical cost of cataract among those 65 years or older accounts for a 56% share of the total cost of major visual disorders. Among adults 40 years or older, cataract accounts for 42% of the overall costs of major visual disorders. These figures show that cataract is an economic and social burden; moreover, this burden is worse in less developed countries. As the overall population ages, the medical cost for cataract will continue to rise, taking away resources from other important government programs, such as education, housing, security, and transportation. Because the surgical treatment of cataract accounts for the majority of this medical cost, it is clear that the development of a nonsurgical medical treatment to delay or prevent cataracts would have a significant economic impact.
Socio-Economic Issues as Regards Medical Treatment of Cataracts
Will the development of a nonsurgical treatment of cataract benefit patients? Most patients would immediately and unequivocally answer “Yes.” As a rule, in medicine, one tries noninvasive treatments first and resorts to invasive surgical procedures only when medical treatment fails. It is common practice for the surgeon to weigh the risks versus the benefits of surgery with the patient and then to perform the surgery only when the benefits outweigh the risks. For cataract surgery, these risks include poor visual results, the loss of vision, the loss of the eye, and disfigurement. In comparison, the risks associated with medical, nonsurgical treatments are primarily side effects associated with the systemic absorption of the drug. These potential risks are reduced by topical application of the drug, because of the low concentration and small volume of the drug minimizes systemic absorption. Because cataracts usually progress slowly, it has been estimated that a 10-year delay in cataract onset could reduce the need for cataract surgery by 50%.2 Therefore, a drug that delays the onset and/or progression of cataract formation could be expected to have a significant impact not only on reducing cataract-related blindness, but also on decreasing medical expenditures associated with the surgical removal of cataracts.
In medically advanced countries, small incision phaco-emulsification cataract extraction followed by foldable intraocular lens implantation is currently the preferred and most common form of cataract surgery. Because it is safely and efficiently performed, the major impact of a medical treatment for cataracts in developed countries would be in reducing surgical costs for our aging population, in addition to providing an alternative treatment for patients at high risk for surgery.
In third world countries, adequate surgical facilities and well-trained eye surgeons are scant with patients often having to travel long distances to undergo cataract surgery. Nd:Yag laser posterior capsulotomy, often required for the postoperative clouding of the posterior capsule, may also not be readily available. This also can contribute to increased morbidity. To address the need for adequate surgical facilities, laudable efforts have been made in the creation of surgical centers funded by WHO and nongovernmental organizations in places, such as India and China. Medical missions undertaken by private individuals and groups are also performing cataract surgery in remote areas. However, because of the worldwide increase in the aging population, these efforts are not enough. Therefore, a medical treatment for cataract would play an important role in these countries.
It is unlikely that anticataract medications will totally replace cataract surgery.3 Because the cause of cataracts is multifactorial (Fig. 1), it is anticipated that anticataract drugs will only slow down cataract formation, but not completely stop or reverse the process. Therefore, although the number of surgical procedures may be reduced, surgery will continue to be a major component in the management of cataracts. This is the case in other specialties, such as in urology, where the availability of medical treatment for prostatic hypertrophy has reduced, but not eliminated the need for prostatectomies. Similarly, in glaucoma the recent development of new antiglaucoma drugs has reduced but not eliminated the need for glaucoma surgery. It is also possible that while the drug-treated lens remains optically clear, refractive changes may continue to increase visual distortion to a point where it can only be corrected by lens removal and replacement. With the older population remaining more healthy and active, patients may also elect cataract surgery to counteract the onset of presbyopia and continued refractive changes, which may hamper important activities requiring sharp vision, such as sports activities or the creation of artwork and handicrafts. Finally, as cataract detection methods (Table 1; see Chapter 72A) including assessment of alpha crystallin protein loss in the lens using dynamic light scattering techniques improve, it is anticipated that ophthalmologists will have the ability to predict the onset of uncontrolled and inevitable decline of the lens caused by increased lens protein aggregation and lens opacification.
TABLE 1. Clinical Methods to Test Anticataract Drugs | ||
---|---|---|
|
Anticataract Drug Development
A number of risk factors are associated with cataract formation (Fig. 1 and Table 2; see also Chapter 73A), and age-related cataracts are believed to result from multifactorial insults. The limiting factors in the development of a “general anticataract” drug include not only the identification of a potential lead structure that can modify cataract formation associated with a number of diverse cataractogenic insults, but also the cumulative nature of these insults, their biochemical complexity, and the lack of specific and adequate animal models that mirror human age-related cataract. Nevertheless, common biochemical and biophysical pathways have been identified in the progression of cataracts induced by osmotic changes and oxidative insult,4,5,6,7 which have lead to specific agents to reduce oxidative or osmotic stress. In addition to these drugs, it has been experimentally observed that compounds that are capable of blocking common biophysical protein changes (phase separation inhibitors) can reduce lens modifications that result in opacity formation.
TABLE 2. Causes of Cataract/Risk Factors. | ||
---|---|---|
|
Antioxidants: Oxidation-Linked Cataracts
Antioxidants as potential agents to prevent or retard cataractogenesis have been the subject of speculation and experimentation for many years. Most of these studies, which have been conducted by laboratories worldwide, can directly or indirectly be traced to the seminal work of Antoinette Pirie and Ruth van Heyningen, working at the Nuffield Laboratory of Ophthalmology, Oxford University in the 1960s and 1970s. Their work, and that of those who have followed, clearly established that oxidative damage occurs to molecules and structures in the lens, that it increases and accumulates with increasing age, and that at least some aspects of it are peculiar to, or accentuated in, cataracts relative to clear lenses of comparable age. This includes loss of reduced glutathione, the central molecule in the lens’ defenses against oxidation and the compound most responsible for the redox set point of lens’ cells. Lens proteins also become oxidized as indicated by oxidation of sulfur containing amino acids, disulfide crosslink formation, carbonyl formation, and the production of protein mixed disulfides with glutathione and cysteine. In addition, lens lipids in cell membranes become oxidized.
The lens is a unique organ that is at special risk to oxidation. Chronic oxidative stress comes from at least two sources: (a) light mediated reactions from exposure to sunlight and (b) oxidants generated internally within the eye, either in the lens itself or in the surrounding fluids. The potential role of sunlight, in particular its ultraviolet component, in cataract development has been studied extensively. This relationship was first suggested by Duke-Elder,8 and subsequently confirmed by Pirie,9 who demonstrated that lens proteins exposed to sunlight develop yellow and brown pigmentation similar to that developing in the human lens nucleus with aging and cataractogenesis and that these proteins subsequently cross-link and aggregate similar to proteins found in cataracts. Near ultraviolet radiation can damage lens proteins through direct absorption by aromatic amino acids, particularly tryptophan, or through the mediation of photosensitizers, which can generate various damaging free radicals or activated species. The presence of free radicals has been demonstrated in human lenses,10,11 and it has also been shown that the pigmented proteins present in human cataracts contain near UV photosensitizers capable of generating activated species of oxygen including singlet oxygen.12,13 Some epidemiological studies support this association between sunlight and cataract. Heavily pigmented (brunescent) cataracts have been reported to be much more common at lower latitudes where sunlight is more intense and daylight hours are longer and brunescent cataracts are reported to be more common in individuals with outdoor occupations relative to those working indoors.14 This study is consistent with earlier, poorly controlled studies and anecdotal reports that cataracts are more prevalent and occur at younger ages in geographical areas of more intense solar UV radiation. A major weakness in these studies has been the lack of individual exposure information; furthermore, many of these early studies have failed to separately consider the different types of cataracts (nuclear, cortical, posterior subcapsular). Taylor et al.15 have calculated individual ocular exposures for a group of 838 water men working on the Chesapeake Bay and observed a statistically significant relationship between high cumulative levels of UV-B and cortical cataracts. This association between cortical cataracts and lifetime exposure to UV-B has subsequently been corroborated by a number of other epidemiological studies.16
Although epidemiological evidence tying sunlight exposure to nuclear cataract is lacking, there are excellent reasons to believe that oxidation is a major factor underlying such cataracts. The recent review by Truscott17 presents a comprehensive picture of the biochemistry and physiology of the lens as it relates to human age-related nuclear cataract. It points to the generation of activated species of oxygen within the lens, probably through transition metal catalyzed reactions (Fenton chemistry), as critical effectors of the oxidative damage present in such cataracts. Oxygen radical damage is controlled in the normal lens by the very low levels of oxygen present there18 and by the intrinsic antioxidant defenses keyed by the presence of adequate levels of reduced glutathione. With increases in oxygen levels in the lens nucleus, for example in association with hyperbaric oxygen (HBO) therapy or vitrectomy surgery, the probability of nuclear cataract formation increases dramatically. This strong association between clinical HBO treatment and the subsequent development of nuclear cataract has been reported by Palmquist.19 Subsequently, Giblin20 found that HBO exposure to animals resulted in lenticular loss of glutathione and protein sulfhydryls, protein in solubilization, increased nuclear light scattering, and membrane damage. All these are features of human nuclear cataracts. Older patients (>50 years) who undergo vitrectomy surgery have a 60% or greater likelihood of developing nuclear cataract within 2 years. Holecamp et al. have demonstrated that marked increases in oxygen tension occur within the human eye during vitrectomy surgery and that after the surgery, oxygen tension is increased for at least 10 months, because the normal oxygen gradients within the posterior compartment of the eye are destroyed by the loss of the vitreous gel.21 Therefore, it seems highly likely that increased exposure of the lens to oxygen is the cause of postvitrectomy nuclear cataract.
Antioxidant Vitamin Therapy
The clear association between oxidative damage and cataract formation, as outlined previously, constitutes the basic rationale underlying the studies on antioxidants as possible anticataract agents. A strong focus has been on the potential of antioxidant vitamins as modulators of cataractogenesis. The results of over 70 epidemiological studies aimed at determining whether intake of such vitamins, in particular vitamin C, vitamin E, and carotenoids, reduce the risk of developing age-related cataract has recently been summarized.22 Most of the studies have been observational studies rather than randomized controlled clinical trials. Although the observational studies have varied widely in the specifics of their findings, the overall impression is that antioxidant nutrients provide some protection from cataract development. However, the limitations inherent in observational studies make such a conclusion far from definitive. Furthermore, most of the randomized trials testing the effects of vitamin supplementation have not found a protective effect. This lack of protection may possibly be due to the populations in these randomized trials already having fully adequate levels of antioxidant nutrients, because one of the randomized trials where a protective effect was observed was conducted in rural China where nutrient levels were likely less than optimal.23 In the most recent U.S. study, the Age Related Eye Disease Study (AREDS), no relationship was found between vitamin supplementation and development or progression of cataract. The interested reader is referred to recent reviews22,24 for further information on the epidemiological aspects of antioxidant nutrients and cataract.
Experimental Antioxidant Studies
The ideal method for preclinical testing of potential anticataract agents is an animal model where lens opacification closely resembles human aging-related cataract. Unfortunately, no such animal model has been identified. Several animal models that share certain features with human cataract have been used, but it is generally accepted that none of these models adequately reflect the complexity of human age-related cataract. In addition to the inherent difficulties in developing a model that mimics the multifactorial nature and the slow and variable progression characteristic of human cataract, there are also concerns regarding the use of laboratory rodents in such studies, because there are fundamental biological differences between rodent lenses and the human lens.17
Much of the experimental work on antioxidants has been done using either lens epithelial cell cultures or lens organ culture systems. Lens epithelial cells from various species, including human, can be successfully cultured. In addition, immortalized cell lines have been produced from such cultures. Although much of our knowledge of basic lens biology has been derived from work on lens epithelial cell cultures, there are significant limitations inherent in the use of such systems to study cataract mechanisms. Primary cultures provide a limited number of cells such that many types of studies are precluded; transformed cell lines overcome this problem, but such cells quickly lose important characteristics of lens cells, for example, the synthesis of crystallins and other lens specific marker proteins.25,26 The specific relevance of findings generated using such cultures to the lens and cataract has been questioned. In spite of these concerns, it is clear that important information relating to the effects of oxidative stress on lens epithelial cells and on the responses of the cells to various forms of oxidative stress have been derived from studies on cell cultures.27,28,29,30 Because the lens epithelium is the most metabolically active portion of the lens and it is thought to be the initial site of damage in at least some forms of human age-related cataracts,31 studies using lens epithelial cell cultures will continue to be an important source of information on the process of cataractogenesis.
A second model system used to study mechanisms of cataract and to test putative anticataract agents has been lens organ culture. Because of its unique biology, lacking both vascular and neural connections, the lens is especially suited to organ culture. In vivo the lens obtains all nutrients and eliminates all waste products via diffusion with the surrounding fluids. Thus, dissecting the lens from the eye and placing it in a culture medium approximating the aqueous humor produces minimal changes in the conditions where the lens exists. It has been demonstrated by various investigators that cultured lenses can remain viable and transparent for periods of days to weeks or even months depending upon species, conditions of culture, and biochemical parameters assessed. Using the intact lens in culture allows the direct study of cataract formation (lens opacification) without requiring experimentation on live animals. Cataract can be induced in cultured lenses by a variety of stresses including oxidation and the effects of antioxidants or other potential protectants can be assessed. In addition to transparency, a variety of physiological and biochemical parameters can be used to measure effects on the lens, including membrane transport, enzyme activities, ion fluxes, protein or DNA damage, and metabolite levels.31,32,33,34,35 The most important systems of oxidative stress that have been applied to cultured lenses have been designed to produce activated species of oxygen either through photo-oxidative mechanisms or by addition of hydrogen peroxide to the culture medium with subsequent generation of radical species via Fenton chemistry. Much has been learned from these studies and, in addition, protective effects of various antioxidants have been assessed. Among the antioxidants tested have been agents designed to increase glutathione levels in the lens,36 low-molecular-weight mimics of glutathione peroxidase,34 vitamin C, chelating agents, pyruvate, and various sulfhydryl compounds.
A number of animal models of cataract have been used to test antioxidant compounds as possible drugs to prevent or retard cataract. The models have, in general, used rabbits or rodents as the experimental species and, thus, one must recognize the potentially important biological differences between their lenses and the human lens.17 Probably the two animal models most used for this purpose have been sugar cataract and the selenite cataract, both of which utilize rats. Sugar cataracts (diabetic- and galactose-induced) are discussed at length elsewhere in this chapter; we note here only that while not the initiating insult, oxidation is involved in sugar cataract formation. The selenite model produces nuclear cataract in rat pups following a single subcutaneous injection of sodium selenite. The system is attractive because of its high reliability and short timeframe in producing nuclear cataract. Although the mechanism responsible for cataract formation is poorly understood, experimental reports confirm the importance of calcium, proteolysis, and oxidative stress as central factors. Several compounds purported to have antioxidant properties, including vitamin C,37 extracts of green and black tea,38 N-acetyl-carnosine,39 and α-ketoglutarate40 have been reported to reduce the cataractogenic effects in the selenite model.
A clear example of an in vivo cataract with an oxidative etiology is radiation cataract produced in rabbits or rodents by ionizing radiation (x-ray or gamma radiation). A number of antioxidants with active sulfhydryl groups have been shown to inhibit such cataracts.41,42,43 Similarly, Soderberg et al. have extensively studied the cataractogenic effects of UV-B on rats and have reported that vitamin E reduces the severity of these cataracts.44 Other animal models of interest include the Emory mouse,45,46 which develops late onset cataract. Although antioxidants have been used infrequently with this model, caloric restriction that reduces endogenous oxidant production from metabolic reactions inhibits cataract formation in the Emory mouse.47 In addition, Wolf et al.48 have studied age-related cataract development in five genetically engineered mouse models with altered levels of the antioxidant enzymes GSH peroxidase 1, superoxide dismutase, and catalase. Their findings demonstrate that these enzymes are important in protecting the lens from cataract formation.
Clinical Trails (Clinical Studies) (Antioxidants and Human Studies)
Many laboratories working over the last several decades have developed a convincing body of data demonstrating that oxidation is a major, if not the major, cause of human aging-related cataract. Furthermore, many studies have demonstrated that antioxidants are efficacious in inhibiting cataract in a variety of in vitro and animal models. It is surprising, therefore, that not a single FDA-approved drug for the prevention or inhibition of cataract has been developed. This may be attributable to several factors including the complexity of chronic, age-related diseases, such as cataract, the lack of a good animal model for the disease, an incomplete understanding of the biology of the lens especially during aging, and the fact that the antioxidants that have been tested in humans have been vitamins and other nutrients. There is a need for development of novel antioxidants that could effectively augment the endogenous antioxidant defenses of the lens. Currently, there is at least one compound in a clinical trial in the United States with that potential. Tempol-H is the reduced (hydroxylamine) form of the nitroxide Tempol (4-hydroxy-2,2,6,6 tetramethylpiperidine-N-oxyl) (Fig. 2). Nitroxides are stable free radicals with antioxidant properties; Tempol is a radioprotective agent, which effectively breaks radical chain reactions by reacting with other free radicals, exhibits superoxide dismutase-like activity, and can inhibit Fenton chemistry by keeping transition metal ions in the oxidized state.49 Tempol was one of numerous compounds that were tested for anticataract activity using a lens organ culture screening system.35 Lenses exposed to Tempol in this system were found to become damaged, however, no toxic effects were observed with the reduced form, Tempol-H, and the lenses were found to be markedly protected from cataract formation. This was true both for young rat lenses exposed to various damaging levels of hydrogen peroxide and also for rhesus monkey lenses cultured in medium containing 30 mM xylose. In addition to the prevention of opacification, the protective effects of Tempol-H were also seen in the maintenance of membrane transport parameters, protection of glutathione levels in the lens, and prevention of protein leakage from the lens. The compound also exhibited good bioavailability, freely crossing the lens capsule and cell membranes to enter the lens cells. Difficulties arose when Tempol-H was tested as an anticataract agent in vivo by topical application to the eye. Although no toxicity was observed, there was also no effect on cataractogenesis, and it was subsequently determined that Tempol-H failed to penetrate the cornea. This problem was overcome when Othera Pharmaceuticals, Inc. (Exton, PA) licensed the patent for the use of the compound as an anticataract agent and produced a pro-drug form of Tempol-H. This preparation (OT-551) readily crosses the cornea and is then hydrolyzed by endogenous esterases to release Tempol-H within the eye. Because Tempol and Tempol-H redox cycle freely within cells, it is believed that the antioxidant activity is catalytic-like in nature. OT-551 is currently in a Phase II clinical trial in a postvitrectomy patient population. Preliminary results may be available by the end of 2007. It should be noted that Othera is also testing the compound as a potential drug for age-related macular degeneration and, in that regard, a recent paper by Tanito et al.50 demonstrated protective effects in a light damage model in rats.
Diabetic Cataracts: Aldose Reductase Inhibitors
Therapy for diabetic cataracts has focused on biochemical changes observed during sugar cataract formation. These include oxidative stress, glycation, and excess sorbitol pathway activity, which can result in osmotic stress.
Aldose Reductase and Sorbitol Pathway Activity
Extensive experimental evidence supports the observation that diabetic and galactosemic cataracts progress through a common mechanism that is initiated by aldose reductase, AR, the first enzyme in the sorbitol pathway. This concept was developed from observations that (a) diabetic and galactosemic rats demonstrate similar histopathological lens changes that include the similar, initial appearance of hydropic lens fiber cells that rupture with liquefaction to form vacuoles and subsequent lens opacities51 and (b) that the enzyme AR is present in the lens and reduces glucose to sorbitol in diabetic rats and galactose to galactitol in galactosemic rats.52 Combining these observations, Kinoshita demonstrated that the intracellular accumulation of sorbitol or galactitol leads to osmotic changes that result in hydropic lens fibers that degenerate to form sugar cataracts.53,54,55 In the lenses of diabetic animals, physiologically significant levels of sorbitol accumulate, because sorbitol is produced faster than it is converted to fructose by sorbitol dehydrogenase, the second enzyme of the sorbitol pathway. Because the polar character of sorbitol hinders its intracellular removal through diffusion, the accumulating sorbitol creates a hyperosmotic effect that results in an infusion of fluid to counteract the osmotic gradient produced. This swelling initiates the process of sugar cataract formation. Moreover, more rapid and greater hyperosmotic effects result from galactose than glucose, because increased levels of galactose are more rapidly reduced to galactitol than glucose to sorbitol.54 The intracellular galactitol levels remain elevated, because galactitol is not further metabolized by sorbitol dehydrogenase. These observations led to the Osmotic Hypothesis of sugar cataract formation, which states that the intracellular increase of fluid in response to the AR-initiated accumulation of polyols results in lens swelling that is associated with increased membrane permeability and a series of complex biochemical changes that lead to cataract formation.53,55,56
A number of experimental studies support the role of AR in sugar cataract formation. These range from in vitro lens culture studies that demonstrate that lens opacification can be prevented by inhibiting lens sugar alcohol formation55,57,58,59 or preventing lens swelling resulting from sugar alcohol accumulation60,61,62,63 to animal models, such as the sand rat,64,65 Octodon degus,66,67,68 hyperglycemic, galactosemic, and transgenic mice,69,70,71] dogs,72,73,74,75,76 and cats.76 However, the most convincing evidence for the role of AR in cataracts comes from the use of aldose reductase inhibitors (ARIs).
ARIs encompass a broad range of structurally diverse compounds ranging from extracts from plants and animal tissues to specific small molecules. Cataracts have been delayed in diabetic and galactosemic rats with plant flavonoids, such as quercitrin or the isoflavone genistein.67,77,78,79,80 Natural products containing AR inhibitory activity range from ginger (Zingiber officinale Roscoe) that is used worldwide as a cooking spice and herbal medicine81 to diabetic herbal drugs, such as the Korean traditional medicine Aralia elata (Aralia extract),82 the Indian herbal Diabecon,83 and aqueous extracts of Ocimum sanctum, Withania somnifera, Curcuma longa, and Azadirachta indica.84 Lens polyol levels in rats have also been reduced by injection of intrinsic ARI containing extracts obtained from human kidney and bovine lenses.85 Select nonsteroidal anti-inflammatory agents (NSAIDs), such as sulindac,86,87 naproxen,88 or aspirin89,90 also demonstrate weak AR inhibitory activity and delay cataract in diabetic rats. However, cataract formation cannot only be delayed but even prevented through the administration of specific ARIs (Fig. 3). These include in approximate historical order Alrestatin,91 Sorbinil,20,66,92,93 Imirestat (AL1576),94,95 Ponalrestat,96 Tolrestat,57 Epalrestat,97 Methylsorbinil (M79175),20,74,98 Fidarestat (SNK-860),99 Zopolrestat,93 Zenarestat (FK366),100,101 M-16209,102 Minalrestat (WAY-121,509),103 GP-1447,104 Ranirestat (AS-3201),105 ARI-809,106 and Lidorestat.107 Because the timeframe of human diabetic cataract formation has not been clinically established, none of the ARIs have been specifically evaluated for the prevention of cataracts in diabetics. Instead, these inhibitors have targeted the diabetic complications of neuropathy, retinopathy, nephropathy, and keratopathy. In dogs, where the progression of sugar cataracts is more rapid and predictable, the topical ARI formulation Kinostat has been shown to reverse early sugar cataract formation.75 It is currently undergoing a preliminary phase I trial in diabetic dogs for the prevention of cataracts.
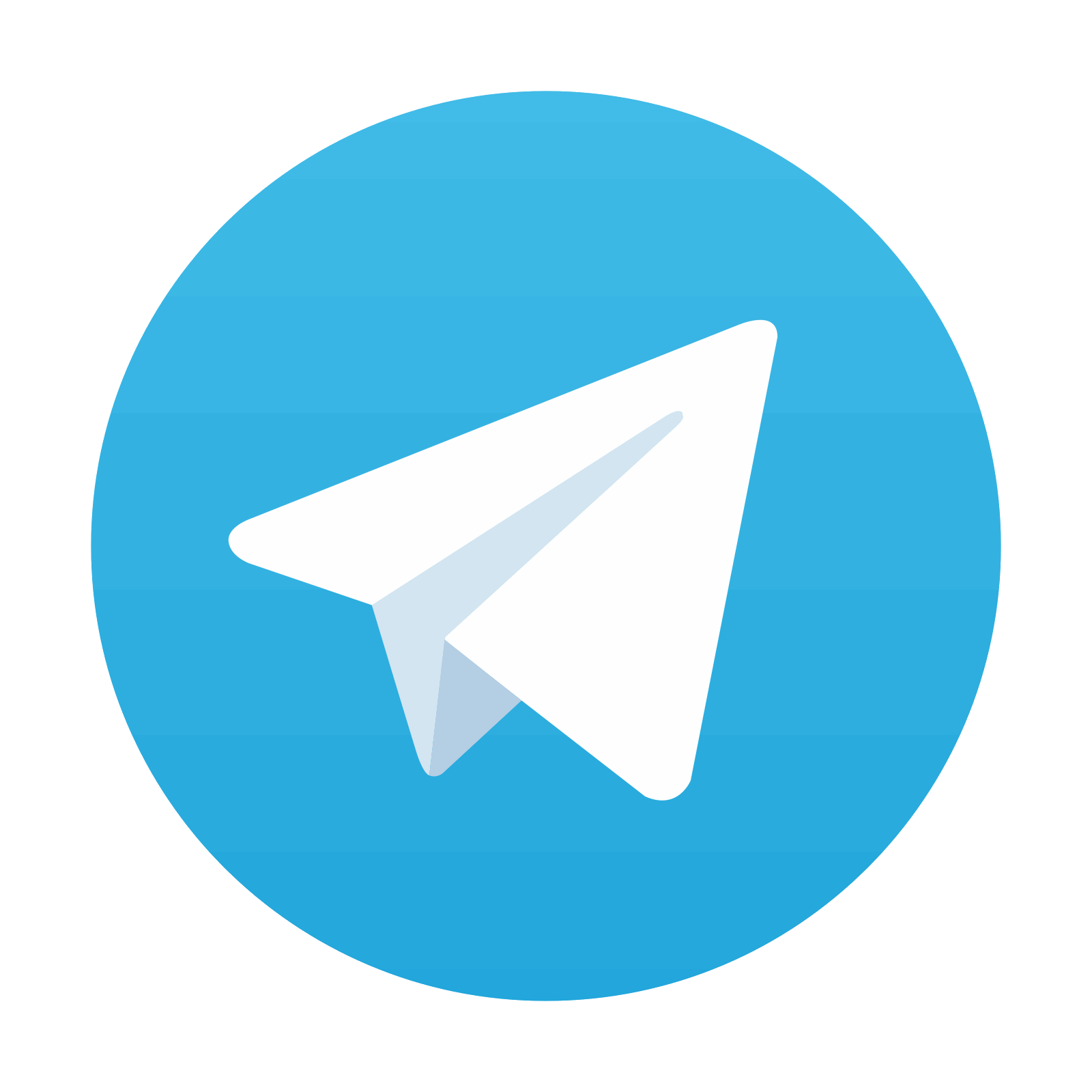
Stay updated, free articles. Join our Telegram channel

Full access? Get Clinical Tree
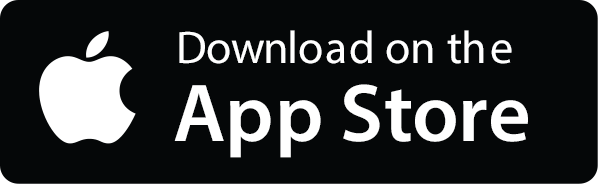
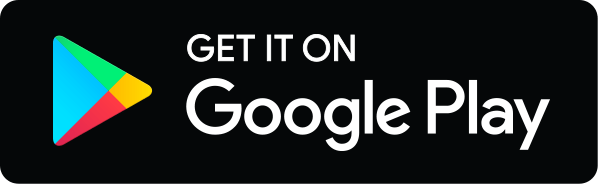