Topical drug delivery for sinonasal disorders is influenced by a variety of factors. Macroscopically (or anatomically), the ability of the drug to reach the appropriate region of the paranasal system is paramount. Delivery techniques, surgical state of the sinus cavity, delivery device, and fluid dynamics (volume, pressure, position) have a significant impact on the delivery of topical therapies to the sinus mucosa. Once topical therapeutics actually reach the desired site, factors within the local microenvironment heavily influence local drug delivery. The presence and composition of the mucus blanket, mucociliary clearance, direct mucin-drug binding, and the permeability of pharmaceutical compounds will all impact drug delivery. In addition, the general therapeutic goal of topical management may lie between the potentially competing actions of mechanical lavage and pharmaceutical intervention. Techniques for the mechanical removal of mucus, antigen, and inflammatory products may not be the most efficient approach for pharmaceutical delivery. This article reviews the evolving concepts in local drug therapy, both for the factors that influence anatomic distribution within the sinonasal system and those that affect mucosal absorption.
Respiratory epithelial damage, mucus hypersecretion, mucociliary dysfunction, and the release of proinflammatory products at the sinus mucosa all mediate the prolonged inflammation of chronic rhinosinusitis (CRS). The perceived role of local microbial flora in CRS has evolved from one of causation to disease modifer. Although there has been a shift to anti-inflammatory therapies in CRS, bacteria and fungi are still likely to be powerful mediators of inflammation. The current model of CRS pathophysiology focuses on the interaction of the inflammatory mucosal disease with microbial flora and the failure of the mechanical and innate immunity ( Fig. 1 ). Current systemic agents, such as oral or intravenous antimicrobials or anti-inflammatories, have significant side effects and are not successful in many patients. This problem has led rhinologists to examine local delivery of topical therapies. These treatment strategies are important in the management of the disordered inflammation of CRS, and are likely to be pivotal to modifying the expanding pathologic mechanisms that mediate this disease.
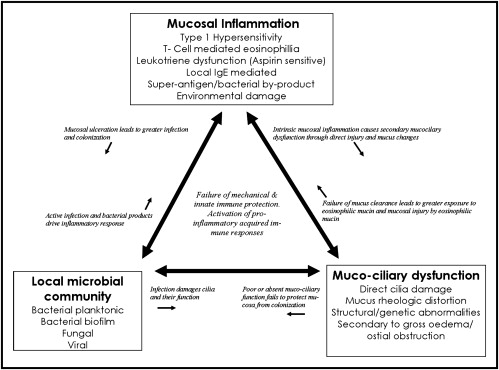
The general therapeutic goal of topical management may also lie between potentially competing actions of mechanical lavage and pharmaceutical intervention. The mechanical removal of mucus, antigen, pollutants, inflammatory products, and bacteria/biofilms is often targeted with topical approaches. These interventions rely on high-volume positive pressure solutions to provide shearing forces with additives to alter air-surface-liquid (ASL) tension. However, the same approach may not be appropriate for delivery of pharmaceutical preparations. Complete sinus distribution, prolonged mucosal contact time with local absorption, and minimal wastage are often the desired properties. There is currently a wide array of antimicrobial, anti-inflammatory and immunomodulatory agents being investigated for CRS, which are beyond the scope of this article. The discussion here focuses on modern concepts in local drug therapy, for the macroscopic factors that affect distribution within the sinonasal system and those factors within the microenvironment that influence absorption ( Table 1 ).
Local Absorption (Micro) | Sinonasal Distribution (Macro) |
---|---|
Mucus blanket | Surgical state |
Mechanical obstruction to diffusion | Device |
Cell surface charge | Position |
Mucin and protein binding | Volume |
Mucociliary clearance | Pressure |
Mucosal residence time | Anatomic dimensions of surgical cavity |
Macrodelivery
The ability of the drug to reach the appropriate anatomic region in the paranasal system will always be important, and has been the subject of much research in the past 5 years. Delivery techniques, surgical state of the sinus cavity, delivery device, and fluid dynamics (volume, pressure, position) have a significant impact on the delivery of topical therapies to the sinus mucosa.
Sinus Surgery
Distribution of topical solution to the unoperated sinuses is limited, and in the setting of CRS with mucosal edema it is probably only on the order of less than 2% of total irrigation volume. Nebulization is also ineffective, with less than 3% sinus penetration. A fundamentally held belief among those treating CRS patients is that endoscopic sinus surgery (ESS) improves the delivery of topical medications to the sinonasal mucosa, yet only recent evidence exists to support this claim. Endoscopic sinus surgery is essential to effectively allow topical distribution to the sinuses. The frontal and sphenoid sinus are essentially inaccessible before surgery ( Fig. 2 ) and an ostial size of greater than 4 mm is required to even begin seeing penetration to the maxillary sinus. For those with mucosal edema and chronic inflammation, distribution is probably worse. In medically managing CRS, the use of expensive and time-wasting topical therapies, such as increasing topical steroid options ( Fig. 3 ), are probably not supported prior to ESS.
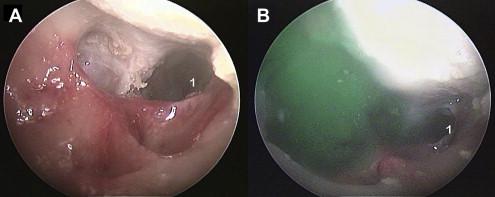
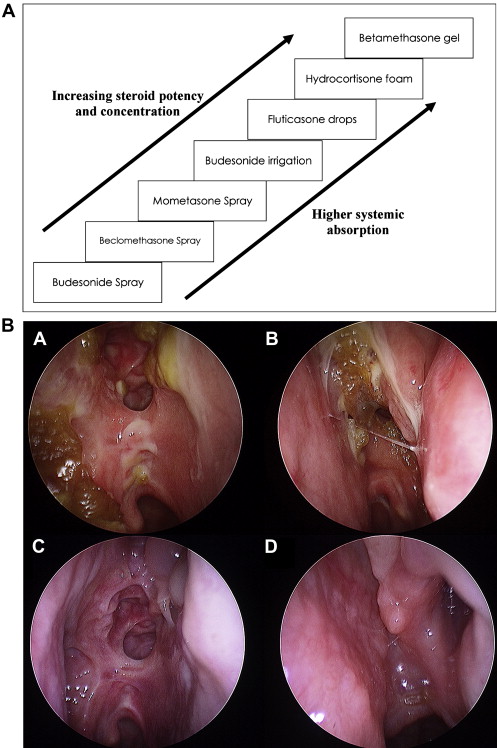
There is growing evidence that a predominant feature of CRS is a shift in mucosal immune response to a proinflammatory process, whether Th1 or Th2 dominated. This process differs from the normal mechanical and innate immunity that provides stable, healthy mucosa. Due to their anti-inflammatory action, corticosteroids have long been the drug of choice for this purpose, and are one of the most widely studied pharmacologic agents. Unfortunately, the evidence for a role of nasal steroids in the management of CRS is weak. Most studies include unoperated patients or a mix of pre- and postsurgical populations. This patient selection raises greater questions of what mucosa one is actually treating in these studies.
The potential for observing a focal effect on secondary turbinate reactivity in CRS without any actual impact on the sinus mucosa is great. Steroid sprays in unoperated patients leads to almost no sinus distribution, so it is not surprising that researchers using steroid postoperatively with direct application to the sinus mucosa have demonstrated benefit, and those with a mixed or unoperated population have found less benefit. In addition, there is a significant difference in the way in which ESS is delivered across institutions, as some post-ESS cavities are opened widely, whereas other surgeons practice techniques to simply dilate or create very conservative sinusotomies ( Fig. 4 ). The heterogeneous group of surgical comparisons makes evaluating locally delivered therapies even more difficult to assess, and minimal techniques have proliferated without consideration of many of these factors.
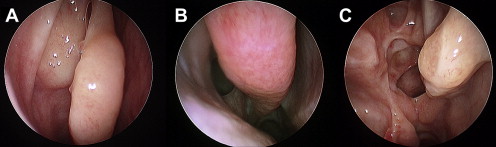
Delivery Devices
Nebulizers poorly penetrate the sinuses even after maximal ESS, and large-volume squeeze bottles or passive flow devices seem to have the best efficacy post ESS. Presurgery, the distribution to the sinuses is extremely limited regardless of device, and sprays are the least effective of all ( Fig. 5 ). Neti pots have advantage in the unoperated sinus, as head position and retrograde flow allows some penetration. Postsurgery distribution is superior with high-volume positive pressure devices. Research into topical distribution often quantifies penetration and surface area covered but not volume, shearing forces, or mucosal contact time. From intrasinus video observation during the authors’ studies, there is a very significant difference in fluid dynamics between irrigation techniques that is difficult to quantify. Simple computed tomography studies or endoscopic grading do not take into account the increased hydrostatic forces seen with large-volume positive pressure devices, such as a squeeze bottle, when compared with large-volume, low-pressure devices such as the Neti pot ( Fig. 6 ). In addition, it is unknown whether these increased irrigation pressures are beneficial. Simple low-volume sprays and drops have very poor distribution and should be considered a nasal cavity treatment only, especially before ESS. Although multiple devices and head positions have been trialed, less than 50% of most low-volume applications will reach even the middle meatus.
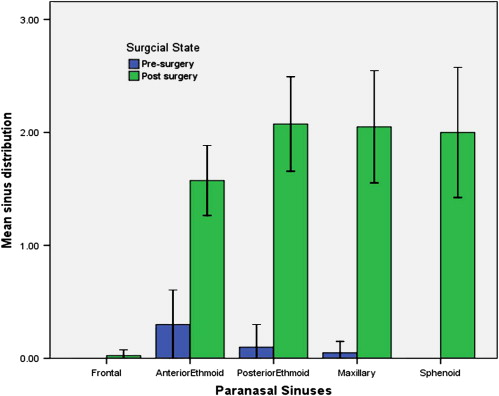
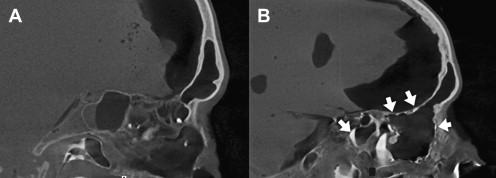
Position for Sinus Fluid Delivery
There are incomplete data on the most effective positioning for delivering fluid to the nasal cavity and paranasal sinuses. The majority of these studies involve assessment of dye “around” the middle turbinate with simple sprays and drops in presurgical patients. Many commercial products recommend a head-down, over sink, with nose to ground position for irrigation. This orientation is practical and makes runoff easy to collect. Evidence for the efficacy in delivery of drops to the middle meatus relative to head position demonstrates that the “Mygind” and “Ragan” (left lateral and supine positions) were superior to “Mecca” and “Head Back” positions in one study but inconclusive in others. The relevance of positioning with positive pressure application may be less significant. However, even with positive pressure high-volume irrigation, head-down or lateral position may lead to better frontal distribution.
Fluid Volume
There are limited data on the exact volume required to allow complete distribution. Higher volumes do seem to penetrate both maxillary and frontal sinus with good coverage starting at about 100 mL. The frontal and sphenoid sinuses are not accessed well by pressurized spray when compared with high-volume devices such as squeeze bottles or Neti pots. From current research, higher volume and positive pressure irrigation are likely to result in the best distribution. Squeeze bottles offer one option for this approach ( Fig. 7 ).
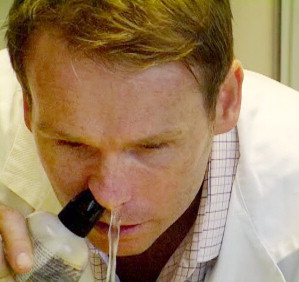
Microdelivery
Significant local mucosal factors are often overlooked in establishing the effectiveness of local drug delivery. Little is still known about the mucus blanket in the airway. Much research is extrapolated from intestinal mucosal study. The presence and composition of the mucus blanket, mucociliary clearance, direct mucin-drug binding, and the permeability of pharmaceutical compounds all contribute to altered drug delivery. For drugs to have good bioavailability and avoid rapid clearance, they must traverse the outer layers of the mucus barrier quickly, and be adherent or rapidly permeate the epithelial surface.
Mucus Blanket
Nasal mucus is a significant barrier to airway drug delivery. Nasal mucus is a powerful first line of defense for the body’s airway as it copes with more than 500 liters of air that is filtered into the system per hour. It is thought that more than 25 million particles are managed every hour by the airway epithelium. The mucus blanket is a viscoelastic gel that forms a film over respiratory epithelium. The blanket’s production is highly inducible and can increase in thickness by as much as 10 μm per second on stimulation. For many inflammatory airway conditions, there is substantial mucus hypersecretion. Hypersecretion is a similar defining feature in rhinitis and CRS.
Enhanced drug delivery without a mucus blanket
Removal of the mucus blanket may enhance drug absorption. There is evidence of a synergistic effect of hypertonic saline and topical steroid delivery. It is possible that removal of the mucus blanket alone may allow better steroid absorption. Alterations to tight junction permeability may also enhance drug absorption. There is evidence that increased tight junction permeability of respiratory mucosa occurs with hypertonic saline and is not seen with equivalent mannitol osmolarity. Whether this leads to better intranasal steroid delivery is a matter of debate. There is evidence to support a synergistic effect of saline and steroid from clinical trials in rhinitis and from nebulized therapy in asthma.
Mechanical removal of sinonasal secretions, antigens, or biofilm
The potential for sprays and irrigations to remove debris, mucus, or antigen has always been implied. However, there is little research to substantiate its effectiveness. There is only indirect evidence of reduced antigen-specific IgE levels in allergic rhinitis sufferers who use saline during the allergy season. High-volume solutions seem to be more effective in managing CRS symptoms than simple sprays, but with mixed groups of pre- and post-ESS patients the degree of benefit is still debatable. Although assumed to be effective, positive pressure irrigations may potentially seed antigens and inflammatory mucus products throughout the paranasal sinuses, as demonstrated by the positive pressure effects of nose blowing.
Diffusion and permeability through the mucus blanket
Nasal mucus consists of a layered non-Newtonian shearing fluid blanket. This blanket produces a periciliary layer with watery secretions that maintains fluidity for ciliary coupling of the outer layer, commonly referred to as the gel or viscoelastic gel layer. Of importance, each layer is relatively unstirred, with shearing forces between the 2 layers. Secreted surfactants are thought to concentrate in the transition zone and facilitate this process. The volume and thickness is difficult to determine, as techniques for imaging the layers often add to distortion. An accepted average thickness is 15 μm, consisting of at least 5 to 10 μm for the periciliary layer to allow immersion of the cilia. This layer combines with a gel layer of various thicknesses, 7 to 30 μm in healthy states and greater than 50 to 200 μm is disease states. The blanket consists of cross-linked and entangled mucin fibers secreted by goblet cells and submucosal glands. Mucins are approximately 3 to 10 μm in diameter with a length of about 15 μm. The mucins form a 3-dimensional web with an interfiber space ranging from 10 to 200 μm ( Fig. 8 ). Hydrophobic domains in mucus hinder the diffusion of many drugs. Hydrophobic compounds will often diffuse slower through mucus than water. Although physical obstruction is presented to particulate matter, the mucin fibers are highly elastic and low-affinity interactions do allow larger macromolecules, such as immunoglobulin, to diffuse through the layers. Small neutral molecules diffuse faster, as do liposome and microsphere-bearing drugs.
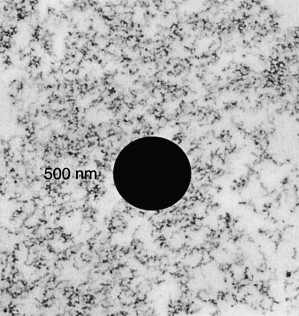
Mucin binding of delivered drugs
In a nondiseased state, the mucus comprises 2% proteins. Mucins are either membrane-bound or secreted, and consist of proline, threonine, or serine domains on linear peptide backbones. High-density mucin glycoproteins define much of the elasticity and viscosity of airway mucus. MUC5B, MUC5AC, and MUC2 are the main secreted mucins in the sinonasal tract. In addition to mucin, long chains of DNA from degraded cells, organic lipids, salts, lactoferrins, lysozymes, immunoglobulins, and other proteins add to the mucus content. Little is known about mucins and other mucus-related proteins regarding their potential drug-binding ability.
Mucociliary Clearance
Sinonasal residence, or the time a formulation is in contact with its absorbing membrane, has important consequences for the bioavailability of a delivered drug. Mucociliary function propels nasal cavity mucus at a rate of 5 to 11 mm/min, and will clear the nasal cavity in 10 minutes and the maxillary sinus in 20 minutes. Researchers have long being trying to improve the mucoadhesiveness of mucosally delivered drugs. Mucoadhesiveness is usually achieved by hydrogen bonding, polymer entanglements with mucins, hydrophobic domains, or a combination of these.
Common polymers employed for this purpose have included carbopol, carboxymethylcellulose, polyacrylic acid, and chitin. Chitosan, a cationic polymer and derivative of chitin, can improve mucoadhesion in oral and nasallly delivered drugs. Powdered delivery polymers such as carbopol can increase bioavailability of apomorphine from 45% to 98% compared with the aqueous formulation. Budesonide esterification can aid entanglement with mucins and improves nasal residency, mucoadhesiveness, and bioavaliability. When combined with polyethylene glycol, budesonide demonstrated longer drug release and absorption. Antibiotics, such as gentamicin and ciprofloxacin, can also be delivered with mucoadherent properties. Hyaluronic acid and hyaluronic acid/chitin microspheres can enhance nasal delivery of gentamicin. Ciprofloxacin, when combined with hydroxypropyl methylcellulose, can reach bioavailability similar to that achieved by the oral route ( Table 2 ). Safety concerns are not without merit, as further disruption to the mucociliary clearance or superinfection of the respiratory tract is possible.
Drugs | Mucoadhesive Agents | Dosage Forms | Bioavailability (%) | Study |
---|---|---|---|---|
Budesonide | (P(MAA-g-EG)) microspheres | Powder | 83.9 | Nakamura et al |
Gentamicin | Hyaluronan | Powder | 23.3 | Lim et al |
Gentamicin | Chitosan | Powder | 31.4 | Lim et al |
Gentamicin | Hyaluronan/chitosan | Powder | 42.9 | Lim et al |
Leuprolide | HPC/MCC | Powder | 34.9 | Suzuki 1999 |
Calcitonin | HPC/MCC | Powder | 16.4 | Suzuki 1999 |
Calcitonin | Chitosan free amine | Liquid | 2.5 | Sinswat 2003 |
Ciprofloxacin | HPMC | Gel | 40.2 | Ozsoy et al |
Ciprofloxacin | HEC | Gel | 19.5 | Ozsoy et al |
Ciprofloxacin | MC | Gel | 18.2 | Ozsoy et al |
Ciprofloxacin | HEC + Tween 80 | Gel | 25.4 | Ozsoy et al |
Ciprofloxacin | MC + Tween 80 | Gel | 22.3 | Ozsoy et al |
Gravity-Affected Areas
Refractory inflammatory sinus disease is often reported in the mucosa of the ethmoid roof and frontal recess. Is the ethmoid roof and frontal sinus an immunologically unique place, or are they simply poorly penetrated and have very short contact time or residency? Combined with poor distribution to these areas, mucosal residence of any therapeutic agent is likely to be further reduced by gravity-dependent flow from the ethmoid roof and frontal sinus, thus making these regions poorly managed with topical therapy.
Novel Agents
Mucoactive agents: surfactants
Amphipathic molecules possess the ability to be soluble in both water and organic solutions; they form the basis of surfactants, which affect both the solution and remaining molecular load behavior at air-surface interfaces. Pulmonary surfactant is the best known clinical example of these important amphipathic molecules. Pulmonary surfactant greatly improves the efficiency of mucociliary clearance by reducing adhesiveness of mucus to the respiratory epithelium. The case of acute respiratory distress of the newborn is an example of when such agents are required in normal respiratory function. Surfactants can have mucoactive and antimicrobial properties. Chemical surfactants can interfere with microbial cell membrane permeability and cause membrane disruption. These agents are often classified as cationic, anionic, or zwitterionic (possessing nonadjacent positive and negative charges) based on the charge of the hydrophilic domain present in these molecules. Cationic surfactants possess the most antimicrobial properties but are also the most irritating.
There are many commercially produced surfactants. Synthetically produced detergents, soil wetting agents, paints, antifogging solutions, and ski wax are all examples. The combination of PEG-80 sorbitan laurate, cocamidopropyl betaine, and sodium trideceth sulfate (commonly known as Johnson & Johnson Baby Shampoo) has been shown to have antibiofilm-forming properties at 1% solution and clinical efficacy in managing refractory CRS patients. It is currently unclear which subsets of CRS patients would benefit from such therapies.
Citric acid zwitterionic surfactant (CAZS) is currently under study for potential antimicrobial activity. This agent combines the calcium bridge disrupting citric acid with the surfactant, caprylyl sulfobetaine. CAZS was effective in reducing bacterial-forming units within a sheep CRS model but not as effective as topical mupirocin. There are concerns regarding possible ciliary dysfunction from any synthetic additive, and future combination solutions are likely to lead to effective agents.
Mucolytic agents may also have a role in enhancing local drug delivery. Recombinant human DNase, also known as Dornase alfa, has been commonly used in the treatment of cystic fibrosis. Dornase alfa hydrolyses the long chains of DNA that forms further entanglements in mucins. Although this has helped improve the viscoelastic properties of mucus, drug diffusion rates do not seem to be affected. Other mucolytics may have a role in improving absorption. N -Acetylcysteine breaks the mucin cross-linking by cleaving disulfide bonds. Mucolytics have been shown to improve the mucus diffusion for nonviral gene vectors.
Second-generation mucoadhesives
Newer mucoadhesives have drug-polymer interactions that allow sustained drug release to the mucosa with less toxicity to normal sinonasal physiology. Polymers that alter their viscoelastic properties based on pH or thermal changes may provide one approach. Budesonide-linked copolymers of polymethacrylic acid and polyethylene glycol in powder form swell in response to pH changes, creating a gel, and provide a sustained release in nasal mucosa. pH-sensitive chitosan and polycarbophil also have similar potential for nasal delivery. Thermoresponsive polymers have produced up to 11-fold increases in DNA absorption, and can be manipulated for “gelation” to occur at sinonasal temperatures. Other bioadhesive activity, such as cytoadhesion, may also improve delivery. Lectin, a naturally occurring glycoprotein, can be linked to therapeutic molecules for epithelial adhesion and internalization. Toxicity of these newer bioadhesives will need to be demonstrated, as they are not simply modifications of compounds already in use.
Mucopenetration
Mucoadhesion strategies that attempt to enhance local drug delivery by overcoming natural respiratory defense mechanisms will always have limitations. Mucociliary clearance, gravity-dependent drainage, mucin binding, and the perpetual turnover of mucus are normal physiologic actions that will lead to diffusion and removal of any active topical agents before these agents actually reach the respiratory epithelium or other cellular targets, thus hindering this approach. Mucus-penetrating strategies, such as nanoparticles smaller than 100 μm, will enable novel drugs to be delivered via a transmucosal route ( Fig. 9 ). Alterations to surface chemistry to incorporate uncharged and hydrophilic polymers, such as polyethylene glycol (PEG), may provide a relatively mucoinert compound able to traverse the mucus blanket and be rapidly absorbed. This approach improved absorption 3-fold for 100- to 500-mm particles and to a greater degree for larger molecules. Mucopenetrating, noncharged surface nanoparticles with immunogenicity similar to viral vectors may allow for an effective drug delivery system to mucosal surfaces.
