The applications of endoscopic techniques have expanded beyond the treatment of inflammatory sinus disease and toward the resection of anterior and middle skull base lesions. Image-guided surgery has emerged as an important tool that compensates for the limitations of surgical endoscopy. The disadvantage of image-guided surgery, however, is its dependence on preoperative imaging data. Intraoperative imaging provides near real-time imaging that has the potential to improve surgical outcomes and reduce operative morbidity. The role of intraoperative imaging in endoscopic sinus and skull base surgery has demonstrated great promise in recent literature. It has had an impact on surgical decision-making during functional endoscopic sinus surgery and the resection of anterior skull base neoplasia. Advances in portable MRI and volumetric CT technology have enhanced the efficiency and safety of intraoperative imaging. Although further studies are required to quantify the precise utility of this new technology, it appears that intraoperative imaging will be an important tool for rhinologic surgery.
Advances in endoscopic surgery have revolutionized the evaluation and management of sinonasal diseases over the last 2 decades. Experience with endoscopy has led to the application of endoscopic techniques to regions beyond the paranasal sinuses, including adjacent areas of the skull base. Endoscopic skull base surgical techniques, compared with traditional craniofacial approaches, may allow pathology in these regions to be treated with reduced morbidity. Technological advancements have preceded major improvements in paranasal sinus skull base surgery. The cornerstone of these technological improvements has been the rod-lens endoscope, which has enabled adequate visualization, illumination, and magnification within the paranasal sinuses as well as at the boundary of the paranasal sinuses at the anterior and middle skull base and orbit. Unfortunately, the rigid endoscopes only provide a two-dimensional (2D) representation of a complex three-dimensional (3D) space. Over the past 10 to 15 years, image-guided surgery has emerged as an important tool that compensates for at least some of the intrinsic limitations of surgical endoscopy. An important limitation of image-guided surgery is its dependence on a preoperative image data set. Obviously anatomic manipulation resulting from the surgical procedure is not represented in the preoperative imaging. Intraoperative imaging provides an opportunity to obtain near real-time information that can be used to update the navigation system data set and alter surgical decision-making. Advances in intraoperative imaging technology potentially may enhance the effectiveness and reduce the morbidity of endoscopic procedures of the paranasal sinuses and skull base.
Intraoperative imaging with or without image-guided surgery also has applications beyond standard endoscopic sinus surgery. By giving a real-time update for image-guided surgery, intraoperative imaging may permit even more extensive procedures of the skull base. Furthermore, intraoperative imaging may serve important roles in craniomaxillofacial procedures, including a role in reducing complex facial fractures. In this role, intraoperative imaging gives immediate information intraoperatively about the positioning of bony fragments and thus guides the surgeon for their optimal placement and fixation.
Terminology
Nearly 20 years ago, the International Society for Computer Aided Surgery proposed a broad definition of computer-aided surgery:
The scope of Computer-Aided Surgery encompasses all fields within surgery, as well as biomedical imaging and instrumentation, and digital technology employed as an adjunct to imaging in diagnosis, therapeutics, and surgery. Topics featured include frameless as well as conventional stereotaxic procedures, surgery guided by ultrasound, image-guided focal irradiation, robotic surgery, and other therapeutic interventions that are performed with the use of digital imaging technology .
Broadly speaking, computer-aided surgery encompasses all semiconductor-based technologies with surgical applications. Thus, computer-aided surgery includes surgical navigation, computer-aided image review, stereotactic surgery, robotic surgery, telemedicine, and electronic medical records.
Over the years, the term image-guided surgery has emerged as the preferred term for surgical navigation applications in endoscopic sinus surgery. From a practical standpoint, image-guided surgery includes both software-enabled review of imaging data as well as surgical navigation, since modern image-guided surgery systems easily support both applications during surgical procedures.
By default, diagnostic imaging, even if it is acquired using semiconductor-based technology, is not part of computer-aided surgery because, unlike computer-aided surgery, diagnostic imaging does not enable the surgeon to manipulate imaging data so as to develop a functional representation of the relative anatomy. Treating a computer like a digital light box for reviewing a CT scan is not computer-aided surgery; however, studying those images using various software tools, which may enable image reconstruction/segmentation and window level/width adjustments, is computer-aided surgery.
Intraoperative imaging refers to the acquisition of imaging data during actual surgical procedures. Because intraoperative imaging data is reviewed interactively at a computer workstation and then commonly uploaded for image-guided surgery, intraoperative imaging is part of computer-aided surgery.
Equipment for intraoperative imaging
Intraoperative imaging requires equipment for image acquisition and transfer as well as review and manipulation. In some scenarios, image-guided surgery will also be employed and, thus, the images from intraoperative imaging must be transferred to the image-guided surgery computer as well. A computer workstation is required to view and manipulate the intraoperative images; similar hardware is also a part of the image-acquisition process. Finally, software is needed to upload the newly acquired images into the navigation system.
The ideal requirements for intraoperative imaging include portability, rapid image acquisition, compatibility with commercially available image-guided surgery systems, and patient safety. Both CT and MRI may be used for intraoperative imaging, although CT is much more commonly accepted.
Cone Beam and Multidetector CT
Cone beam CT (CBCT) imaging has emerged over the last decade as a technology that fulfills the aforementioned criteria. Having gained wide acceptance within the dental and oral surgery fields, CBCT is replacing conventional radiography for in-office diagnosis of periodontal and temporomandibular joint disease. Its applications are expanding into otorhinolaryngology for intraoperative and in-office diagnosis of sinonasal and otologic disorders. Several commercially available systems are available, including the xCAT ENT (Xoran Technologies, Ann Arbor, Michigan), CereTom (Neurologica, Danvers, Massachusetts), Iluma (Imtec, Ardmore, Oklahoma), and the O-arm (Medtronic, Jacksonville, Florida).
CBCT differs from multidetector CT (MDCT) by its imaging geometry. In conventional MDCT, a narrow fan-shaped beam of radiation is passed through the patient to a detector on a revolving gantry. Data is acquired through slices in the axial plane and an image is created by stacking these images. CBCT, based on volumetric tomography, uses a single diverging cone of radiation that penetrates through the patient and is detected on a 2D area detector. CBCT permits the structure of interest to be imaged within a single rotation. This effectively reduces the time and radiation exposure of image acquisition over MDCT.
CBCT provides better image resolution than MDCT as a function of voxel size and geometry. MDCT relies on rectangular voxels oriented with its longest dimension in the axial plane. Although the surface dimension may approach 0.625 mm, the depth remains in the range of 1 to 2 mm. CBCT resolution is based on isotropic voxels that are equal in all three planes, which permits resolutions as fine as 0.125 mm. Image quality provided by CBCT is quite good. In a study by Hashimoto and colleagues, CBCT was found to produce better image resolution than MDCT for the evaluation of alveolar bone and tooth anatomy when viewed by blinded dental radiologists.
Other benefits of CBCT include easier patient positioning, lower cost, and reduced radiation exposure. MDCT requires the patient to be perpendicular to the x-ray beams within the gantry. Because CBCT creates an image of the entire structural volume, head positioning is not an important factor. The cost of CBCT technology is less than that of MDCT since a motorized mechanism for patient transport through the gantry is not required. The efficiency of the photons with CBCT reduces the heat expenditure within the x-ray tube and reduces the frequency of replacement and maintenance.
CT Devices
Recent advances have reduced the footprint for intraoperative imaging with CT. The intraoperative CT and fluoroscopy units are mobile and can be transported between operating rooms. The xCAT device has dimensions of 32 × 47 × 60 in and weighs approximately 500 lb ( Figs. 1 and 2 ). Imaging acquisition occurs at 300 slices in 60 seconds and can obtain a minimum thickness of 0.4 mm. The workstation has the capability of reconstructing triplanar and 3D images.
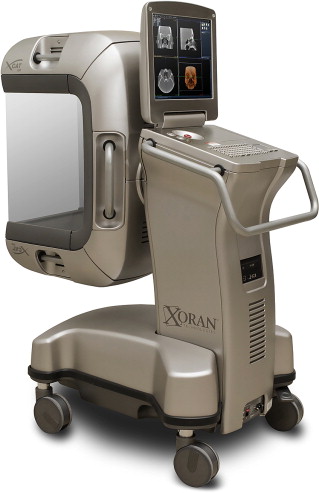
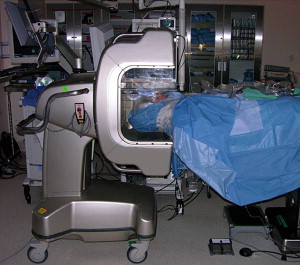
A similar device is the Iluma scanner, which also acquires images by cone beam tomography and flat panel detection ( Fig. 3 ). Ryoo and colleagues demonstrated that the Iluma and miniCAT (in-office version of the xCAT) devices produced images of equivalent resolution. The OTOscan device (Neurologica, Danvers, Massachusetts) incorporates multidetector technology, which permits direct coronal imaging and an adjustable scan distance (1–60 cm). The disadvantage is its size, which requires a 9 × 5-ft floor plan. Both the Iluma and OTOscan devices are not configured for use in the operating room. For all CBCT systems, the use of intraoperative CT and fluoroscopy requires that precautions for protecting the patient and operating room staff from ionizing radiation exposure must be in place.
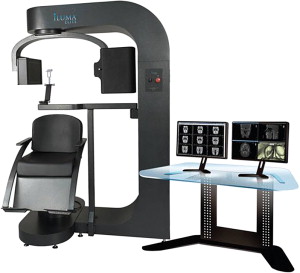
MRI
Intraoperative MRI has been used in the neurosurgery field for over 20 years. Over that time, the technology has evolved to permit the greatest resolution without limiting portability and patient access in the operating room. MRI scanners transmit radiofrequency signals, which are absorbed by protons within the target tissue, and then receives the signals emitted from those protons within the relaxed state. Image quality is proportional to the magnetic field strength. The features of an optimal intraoperative MRI system, including small size to permit portability and open structure to allow access to the patient, conflict with the ability to obtain high-resolution images ( Fig. 4 ). Intraoperative MRI devices with higher field strengths (1.5 to 3.0 T) permit advanced functionality, including MR angiography, MR venography, diffusion-weighted imaging, and functional MRI. The disadvantage of these systems is that they rule out the use standard surgical instruments near the magnet. This means the patient usually has to be transported into the MRI scanner.
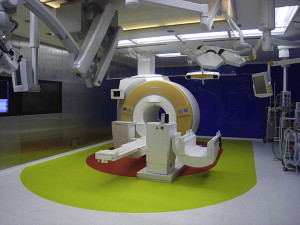
The low field strength (0.15 T) intraoperative MRI systems permit only partial imaging and therefore have limited diagnostic applications. The PoleStar device (Medtronic, Minneapolis, Minnesota) is a low field strength system. Its field of view is approximately 16 × 20 mm. Standard imaging modalities are available with this system including T1, T2, and fluid-attenuated inversion recovery (FLAIR) sequences. Scan times range from 8 seconds to 13 minutes with slice thickness between 2 and 8 mm. The PoleStar device offers the benefits of compactness and portability. It weighs approximately 650 kg, making it possible for two people to easily move the device into position. The magnetic gantry consists of two columns spaced approximately 27 cm apart. The gradient coils are mounted on the outside of these columns. The gantry is powered by both electric and hydraulic sources. It can be placed underneath the operating table when not in use during the procedure. The MRI gantry is typically stored within an iron cage when not in use.
The device still requires dedicated operating rooms and instrumentation compatible with the magnetic fields generated by the MRI coils. At this low field strength, however, ferromagnetic instruments can be as close as 25 cm to the coils without attraction. An important safety mechanism is the ability for the closer magnets to attract the instrument and divert it away from the patient’s head. Radiofrequency shielding is also required within the operating room to reduce the amount of noise generated by electrical equipment. The entire operating room can be shielded or else a cage can be constructed around the operating room table and device.
Three-dimensional Fluoroscopy
Three-dimensional fluoroscopy is another method of intraoperative imaging that has been implemented in the field of rhinologic surgery. Fluoroscopy has been extensively used in the operating room for orthopedic and vascular procedures. Its portability and maneuverability makes this technology ideal for this application. The patient is placed between the fluoroscope’s x-ray source and image intensifier. The x-rays pass through the patient, creating a shadow, which is detected by the image intensifier and video camera. The resulting radiographic image can then be viewed on a portable workstation monitor. New fluoroscopic technology and software have permitted conventional 2D data to be reconstructed into 3D CT-like images. Commercially available products include the OEC 9800 C-arm and FluoroCAT software (GE Healthcare, Lawrence, Massachusetts). The data can then be uploaded onto the InstaTrak workstation (GE Healthcare, Lawrence, Massachusetts) and used as a near real-time update for surgical navigation. A reference array or transmitter is firmly affixed to the patient’s head for registration of the device. The C-arm rotates 190° around the patient and captures approximately 200 images, which are reconstructed into triplanar CT images on the image-guided surgery workstation. Similar to CBCT, this imaging modality provides adequate depiction of bony detail with less radiation exposure than that of conventional MDCT. Manarey and Anand showed that the maximal surface radiation dose using the FluoroCAT device was one eighth that of a MDCT scanner.
Equipment for intraoperative imaging
Intraoperative imaging requires equipment for image acquisition and transfer as well as review and manipulation. In some scenarios, image-guided surgery will also be employed and, thus, the images from intraoperative imaging must be transferred to the image-guided surgery computer as well. A computer workstation is required to view and manipulate the intraoperative images; similar hardware is also a part of the image-acquisition process. Finally, software is needed to upload the newly acquired images into the navigation system.
The ideal requirements for intraoperative imaging include portability, rapid image acquisition, compatibility with commercially available image-guided surgery systems, and patient safety. Both CT and MRI may be used for intraoperative imaging, although CT is much more commonly accepted.
Cone Beam and Multidetector CT
Cone beam CT (CBCT) imaging has emerged over the last decade as a technology that fulfills the aforementioned criteria. Having gained wide acceptance within the dental and oral surgery fields, CBCT is replacing conventional radiography for in-office diagnosis of periodontal and temporomandibular joint disease. Its applications are expanding into otorhinolaryngology for intraoperative and in-office diagnosis of sinonasal and otologic disorders. Several commercially available systems are available, including the xCAT ENT (Xoran Technologies, Ann Arbor, Michigan), CereTom (Neurologica, Danvers, Massachusetts), Iluma (Imtec, Ardmore, Oklahoma), and the O-arm (Medtronic, Jacksonville, Florida).
CBCT differs from multidetector CT (MDCT) by its imaging geometry. In conventional MDCT, a narrow fan-shaped beam of radiation is passed through the patient to a detector on a revolving gantry. Data is acquired through slices in the axial plane and an image is created by stacking these images. CBCT, based on volumetric tomography, uses a single diverging cone of radiation that penetrates through the patient and is detected on a 2D area detector. CBCT permits the structure of interest to be imaged within a single rotation. This effectively reduces the time and radiation exposure of image acquisition over MDCT.
CBCT provides better image resolution than MDCT as a function of voxel size and geometry. MDCT relies on rectangular voxels oriented with its longest dimension in the axial plane. Although the surface dimension may approach 0.625 mm, the depth remains in the range of 1 to 2 mm. CBCT resolution is based on isotropic voxels that are equal in all three planes, which permits resolutions as fine as 0.125 mm. Image quality provided by CBCT is quite good. In a study by Hashimoto and colleagues, CBCT was found to produce better image resolution than MDCT for the evaluation of alveolar bone and tooth anatomy when viewed by blinded dental radiologists.
Other benefits of CBCT include easier patient positioning, lower cost, and reduced radiation exposure. MDCT requires the patient to be perpendicular to the x-ray beams within the gantry. Because CBCT creates an image of the entire structural volume, head positioning is not an important factor. The cost of CBCT technology is less than that of MDCT since a motorized mechanism for patient transport through the gantry is not required. The efficiency of the photons with CBCT reduces the heat expenditure within the x-ray tube and reduces the frequency of replacement and maintenance.
CT Devices
Recent advances have reduced the footprint for intraoperative imaging with CT. The intraoperative CT and fluoroscopy units are mobile and can be transported between operating rooms. The xCAT device has dimensions of 32 × 47 × 60 in and weighs approximately 500 lb ( Figs. 1 and 2 ). Imaging acquisition occurs at 300 slices in 60 seconds and can obtain a minimum thickness of 0.4 mm. The workstation has the capability of reconstructing triplanar and 3D images.
