Light Toxicity in the Posterior Segment
Stephen G. Schwartz
Dan S. Gombos
Susan Schneider
It has long been known that light can cause damage to the posterior segment of the eye. What is the nature of solar damage to the eye and how has the notion of light-induced retinopathy evolved into the concepts recognized today?
Light interaction with the retina can result in various tissue effects. The three ways in which light can damage the posterior segment of the eye are photodisruption, photocoagulation, and phototoxicity.1
Retinal photodisruption is a form of mechanical damage caused by high-powered, ultrashort light exposures, usually nanoseconds to picoseconds in duration. The target tissue is disintegrated and the adjacent tissues are disrupted by shock waves.2 Photodisruption of retinal tissues usually occurs accidentally in conjunction with Q-switched laser use.3
Retinal photocoagulation is a form of thermal damage caused by high-powered, short light exposures, usually between 0.1 and 0.5 seconds in duration.4,5 The target tissue is heated, and the rise in temperature of the affected tissue causes cellular damage, including coagulative necrosis, with subsequent scarring.6 Permanent damage can occur when the temperature is raised by as little as 10°C.1 Photocoagulation of retinal tissues is used clinically, with argon and other lasers. The laser burns produced by these instruments are seen almost immediately.7
Retinal phototoxicity results from a chemical reaction.8 It is caused by low-powered, relatively long light exposures, usually >10 seconds in duration.9 Unlike photodisruption (mechanical) and photocoagulation (thermal), phototoxicity can occur after exposure to a nonlaser light source, such as sunlight.1 The light leads to cellular chemical reactions, with subsequent retinal damage.10 The energy required to cause this phototoxic reaction is related to the wavelength of the incident light.11 Phototoxicity occurs at temperatures much below that seen in photocoagulation.10,11,12,13,14 Clinically, the lesion usually is not detected until 24 to 48 hours after the insult.15
Phototoxicity can be divided into photo-oxidative (photochemical) damage and photosensitized reactions. Most toxic reactions discussed here are related to photo-oxidative interactions between incident light and various retinal tissues.1 Photosensitized reactions include photodynamic reactions, such as used therapeutically in photodynamic therapy with verteporfin (Visudyne, Novartis, Basel, Switzerland) for exudative age-related macular degeneration (AMD).16
History
Light-induced retinal damage has been recognized at least since the time of Plato.17 Galileo reportedly sustained retinal toxicity while using a telescope for solar observation.18
In 1912, Birch-Hirschfeld suggested that eclipse blindness was caused by visible light.19 Verhoeff and Bell opposed this explanation and, in 1916, proposed that solar retinopathy resulted from thermal damage rather than from the photochemical effects of light.20 In 1962, Vos, using mathematical models, estimated that the temperature rise in the retina during exposure to light in cases of eclipse blindness was only about 2 degrees higher than ambient temperature.21 Similarly, Noell et al. proposed a nonthermal phototoxic mechanism for light-induced retinal damage.22
In the mid-1970s, Cain et al. used thermocouple probes to demonstrate that solar retinopathy occurred from photochemical damage (phototoxicity) rather than from thermal damage (photocoagulation).23,24
In 1983, McDonald and Irvine reported the first cases of iatrogenic phototoxicity in patients who had undergone uncomplicated cataract surgery.8 In 1986, Robertson and Feldman established a cause-and-effect relation between the operating room microscope and retinal phototoxicity.25 Since then, numerous publications on iatrogenic phototoxicity have appeared, describing cases associated with cataract surgery,26,27,28,29,30,31,32 other anterior segment procedures,33,34 and pars plana vitrectomy (PPV).35,36,37
Protective Mechanisms
The retina is protected from light toxicity through a variety of adaptations, including physical barriers (eyebrow ridge)38 and various reflexes (squint reflex, blink reflex, and miosis).39 Retinal xanthophyll and melanin pigments may offer additional protection,40,41,42 possibly by converting light to heat, and by suppressing free radicals and photosensitized molecules.12,43 The constant turnover and renewal of rod and cone outer segment discs may also blunt the effects of light toxicity.44 Various biochemical pathways may scavenge toxic molecules, such as superoxide, hydrogen peroxide, hydroxyl radical, and singlet oxygen.45
The wavelength and intensity of light that can be transmitted through the ocular media determine the potential for light-induced retinal damage.46 The wavelength of visible light ranges from 400 to 700 nm.47 Ultraviolet (UV; 100 to 400 nm) and infrared (from 700 to >10,000 nm) light represent nonvisible light adjacent to the extremes of the visible spectrum, and these, as well as the visible spectrum, are capable of interacting with the eye.1
The cornea reflects most of the light not perpendicular to its surface and absorbs incident light in the UV-C (100 to 260 nm), UV-B (260 to 315 nm), and infrared (>700 nm) ranges. The lens absorbs most of the light in the UV-A (315 to 400 nm) range and some UV-B and near infrared light.1,27,48 Its filtering action varies with age-related changes in lens proteins.49 With aging, the lens provides increasing amounts of protection from shorter wavelengths. The aqueous humor absorbs some UV-B, UV-A, and infrared light,50 whereas the vitreous absorbs light in the range of up to 300 nm and some infrared light.51 Early poly(methylmethacrylate) (PMMA) intraocular lenses (IOL) transmitted UV and visible light.1,52 Since 1986, most IOL designs have blocked UV wavelengths.52
The net result is that the ocular media allow transmission of light with wavelengths between about 400 and 1,400 nm (Fig. 3-37.1).46,49,53,54 Of particular concern are the violet (400 to 440 nm) and blue (440 to 500 nm) wavelengths.
Risk Factors
In general, the four most important factors in determining biological tissue response to light exposure are the size of the area affected (optical zone), the wavelength of incident light, the duration of exposure, and the total energy exposure.1 With regard to the retina, two important exceptions to this general principle exist, both of which are related to the unique light-focusing properties of the anterior segment of the eye. First, the optical zone is typically limited to the macula, so in effect the most important factors are wavelength, intensity, and duration.4,55,56,57,58,59,60,61 Second, a light exposure insufficient to cause damage to any other part of the body can cause significant damage to the macula.1
Photochemical (phototoxic) damage is generally caused by shorter wavelengths of visible light. The most potentially toxic wavelengths are in the near-UV (320 to 400 nm) and short wavelength visible (400 to 500 nm) light ranges.62,63 In contrast, thermal injury (photocoagulation) is generally caused by longer wavelengths.64,65
Additional risk factors for phototoxicity, demonstrated in various experimental models, include increased core temperature66,67 elevated blood oxygen levels,12,42,68,69 and the lipofuscin constituent A2E.70 Potential clinical risk factors include diabetes mellitus28 and use of hydrochlorothiazide,28,71 St. John’s wort,74 and perhaps certain IOL designs.9
Clinical Manifestations
Posterior segment light toxicity is probably under reported, because many cases are asymptomatic and objective signs may be subtle. The macular changes may be more evident on fluorescein angiography than clinically (Fig. 3-37.2)
Phototoxicity can be divided into three phases: acute, reparative, and chronic degenerative.15
Immediately after exposure, there may be no obvious sign of disease. A temporary blood–retinal barrier dysfunction may be detected by fluorophotometry, however.75 The first clinically apparent signs are typically mild pigmentary changes, retinal edema, or both, usually within 24 to 48 hours.15 The pigmentary changes may become more visible over the first week. After the first month, the lesions generally become smaller, to a variable degree.15,76,77 Chronic decompensation of the blood–retinal barrier can occur over years.7
Light-induced retinal damage can be permanent,22 but typically improves to a variable extent.42 In general, the prognosis is fairly good.30,31,78,79 Important prognostic factors include the size and location of the lesion.7,36 Secondary choroidal neovascularization can develop.78,80
The clinical syndromes can be separated into those attributed to ambient light and those to iatrogenic causes.
Solar Retinopathy
Solar retinopathy has been recognized for centuries, under the terms eclipse blindness, photoretinitis, photomaculopathy, and foveomacular retinitis.81,82,83,84,85 Solar retinopathy has been described in military personnel, sun bathers, religious sun gazers, solar eclipse viewers, and people under the influence of psychotropic drugs.86 Solar retinopathy is typically photochemically mediated (phototoxic), but can be enhanced by thermal effects (photocoagulation).87
Typically, symptoms begin several hours after exposure and can include periorbital ache, decreased vision, metamorphopsia, chromatopsia, central or paracentral scotomata, and afterimages. Visual acuity may be reduced to 20/200, but may return to 20/40 or better within 4 to 6 months.88,89 The associated symptoms can persist indefinitely.
A small, yellowish foveal lesion may be seen in the acute phase, which corresponds to the retinal image of the sun, approximately 160 μm in diameter.87 As the lesion fades, it usually is replaced by a lamellar hole and may show a corresponding window defect on fluorescein angiography. Alternatively, the angiogram could be normal.
Welder’s Maculopathy
Welding arc exposure is typically associated with photokeratitis; however, retinal damage can occur.90 Clinically, welder’s maculopathy is similar to solar retinopathy.91,92 Macular hole formation can occur in severe cases.93
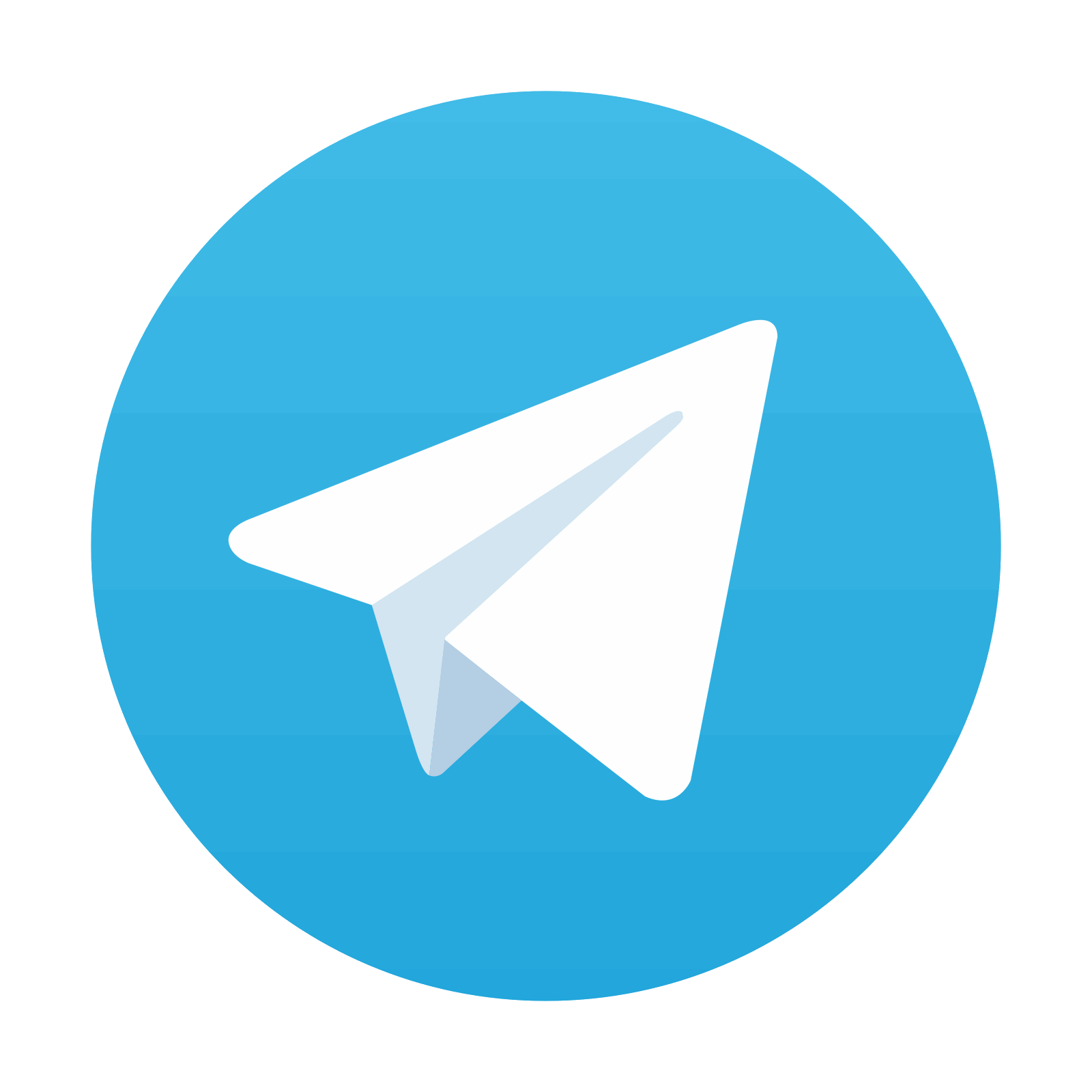
Stay updated, free articles. Join our Telegram channel

Full access? Get Clinical Tree
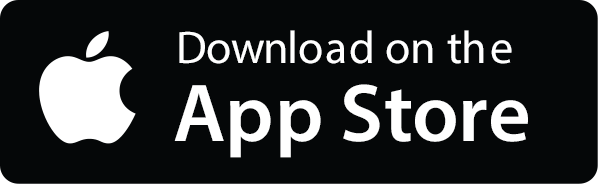
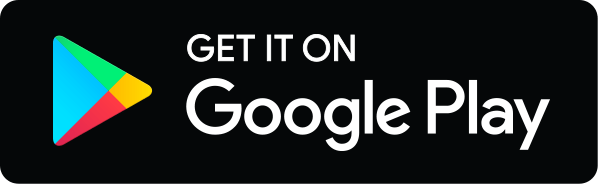