LASIK
Sumit (Sam) Garg
Ann Z. McColgin
Roger F. Steinert
The term keratomileusis comes from the Greek words for “cornea” and “to carve.” Laser in situ keratomileusis (LASIK) combines keratomileusis with the accuracy of the excimer laser and is used worldwide for correction of a broad range of refractive abnormalities. The safety and efficacy of the procedure combined with the quick visual recovery and minimal patient discomfort have made LASIK the most popular refractive procedure for the treatment of all but the highest levels of myopia and astigmatism, and for high hyperopia.1 This chapter summarizes the background, preoperative evaluation, technique, clinical outcomes, and potential complications of LASIK.
BACKGROUND
Jose I. Barraquer2 first described corneal lamellar surgery for the correction of refractive error in 1949. In the late 1950s and early 1960s Barraquer3,4 introduced two landmark contributions in keratorefractive surgery. The first innovation was a manually advancing electric microkeratome for creating a corneal cap. The microkeratomes in use today are based on many of the same general principles as Barraquer’s original design. The second was the use of a cryolathe that froze the corneal cap for precise lenticular reshaping of corneal tissue. The cryolathe was technically difficult to use. The freezing process and subsequent suturing often resulted in irregular astigmatism and loss of best corrected vision.
Swinger, Krumeich, and Cassiday5 later developed a technique for removing corneal tissue without the complex cryolathe. In their system, after the corneal cap was cut by the microkeratome, it was stabilized with suction in the inverted position on a forming die while the refractive cut was made by a second pass of the microkeratome on the stromal side of the corneal cap. The reshaped free cap was then sutured back onto the patient’s corneal stromal bed. This procedure avoided the technical difficulties encountered with the cryolathe but unpredictability and irregular astigmatism remained as major obstacles.
In the late 1980s Ruiz and Rowsey6 introduced the concept of tissue removal from the stromal bed rather than from the free corneal cap, referred to as in situ keratomileusis. In this technique, the microkeratome removed the corneal cap in a first pass, but then the suction ring was changed to excise a free lenticle of tissue with a second pass of the microkeratome. The dimensions of the ring used in the second pass determined the thickness, and thus the dioptric power, of the lenticle removed. Although this technique involved less trauma to the corneal cap, the results remained suboptimal secondary to unpredictable refractive changes and irregular astigmatism. To improve the predictability of the microkeratome cut, Ruiz7 developed an automated microkeratome in the late 1980s. Automated advancement of the microkeratome head, coupled with development of an adjustable suction ring for control of the second microkeratome cut, represented major advances in performing the refractive keratectomy. The lack of predictable tissue removal in the second pass and the small optical zone of the excised refractive lenticle often left the patient with unacceptable optical aberrations, however.
In 1990 Pallikaris8,9 performed the first LASIK procedure when he used the excimer laser instead of the second microkeratome pass for the removal of tissue to induce the refractive change. The excimer laser produced better optical results for three reasons: (a) The excimer laser ablates tissue with submicron accuracy, (b) the laser does not deform the tissue during the refractive reshaping, and (c) larger optical zones are achieved.
The final critical step in achieving consistently excellent results with lamellar refractive surgery was the modification in the microkeratome to stop the pass just short of creating a full free cap. By leaving a narrow hinge of tissue, the outer cornea becomes a flap that is reflected out of the way during the laser exposure, then returned to its original position and allowed to adhere through natural corneal dehydration to the underlying stromal bed (Fig. 49-1). Repositioning of the flap in its original position and avoiding the distortion induced by sutures are critical in reducing irregular astigmatism. Ongoing improvements in microkeratomes continue to make them safer and easier to use. Advances in femtosecond scanning lasers represent another important improvement in creation of the LASIK flap that allow for greater accuracy and safety.10
![]() Figure 49-1. Schematic representation of laser in situ keratomileusis with formation of a corneal flap followed by stromal ablation. The flap is then replaced into its original position. |
Instruments
Although many variations of the mechanical microkeratome exist, the basic principles of the microkeratome cutting head and the role of the suction ring are the same. The suction ring has two functions: to adhere to the globe, providing a stable platform for the microkeratome cutting head; and to raise the pressure in the eye to a high level, which firms the cornea so that the cornea cannot move away from the cutting blade. The dimensions of the suction ring determine the diameter of the flap and size of the stabilizing hinge. The suction ring is connected to a vacuum pump that typically is controlled by an on-off foot pedal.
The cutting head has several key components: (a) a highly sharpened disposable cutting blade, which is discarded after each patient, either after a single eye or bilateral treatment; (b) an applanation plate that flattens the cornea in advance of the cutting blade; the length of blade that extends beyond the applanation plate is a principal determinant of flap thickness; and (c) a motor, either electrical or gas-driven turbine, which oscillates the blade rapidly, typically 6,000 to 15,000 cycles per minute. The same motor or a second motor often is used to mechanically advance the cutting head, attached to the suction ring, across the cornea. In several models, the surgeon manually controls the advance of the cutting head.
The hinge initially was located nasally in the Barraquer-designed microkeratomes. A sliding microkeratome has easiest access to the cornea when approaching from the temporal side, leaving the nasal area the last to be cut. Subsequently, several popular microkeratomes were introduced that allow the microkeratome head to pivot on a post, resulting in an arcing path that leaves the superior zone the last to be cut.11 A superior hinge was touted as a more desirable location because it tended to resist any disturbance of the flap by the wiping motion of the upper eyelid. A nasal hinge did not have this advantage, and also had the drawback that many pupils are located somewhat nasal of the corneal center. As a result, the nasal hinge, even if shifted maximally to the nasal limbus, might impinge on a large treatment zone. Some surgeons have found evidence that a nasal hinge location spares some innervation of the flap from the nasal long ciliary nerves as they enter the cornea, and as a result there may be less tendency for postoperative dry eye in LASIK patients with nasal hinge flaps compared to superior hinge flaps. In contrast, however, one study found more rapid recovery of corneal sensation in patients with superior hinges compared to nasal hinges, although all patients recovered sensation to the preoperative levels within 6 to 12 months after surgery.12
An alternative newer methodology for creating flaps is now widely used in clinical practice.13 Numerous laser platforms using femtosecond Nd-YAG laser pulse technology can create a lamellar dissection within the stroma. Each laser pulse creates a discrete area of photodisruption of the collagen. Thousands of adjacent pulses are scanned across the cornea in a controlled pattern that results in a flap where the computer is programmed for flap diameter, depth, and hinge location and size. Advocates of this complex technology point to the potential for better depth control, avoiding complications such as buttonhole perforations and epithelial defects, and affording precise control of flap dimensions and location. Tanna et al14 retrospectively examined the first 1,000 eyes with femtosecond flaps versus the first 1,000 eyes with microkeratome flaps undergoing wavefront-guided LASIK and found femtosecond flaps allowed for faster visual recovery and better uncorrected visual acuity. In a randomized, controlled, paired eye study, Patel et al15 found corneal backscatter (haze) to be initially higher in the femtosecond eyes, but at 3 and 6 months haze values were equal when compared to microkeratome eyes. They also found that high-contrast visual acuity, contrast sensitivity, and forward light scatter did not differ between treatments at any examination. Another randomized, paired study showed that although femtosecond eyes and microkeratome eyes had similar visual outcomes at 1 year, there was a trend for femtosecond eyes to have fewer induced higher-order aberrations (spherical aberration, coma, and trefoil).16
PREOPERATIVE EVALUATION
The preoperative evaluation for patients prior to LASIK is very similar to the evaluation prior to photorefractive keratectomy (PRK). This chapter is limited to the differences in evaluating the LASIK patient.
As with PRK, careful attention must be given to pre-existing systemic or ocular conditions that may interfere with healing. However, because LASIK violates the corneal surface less than PRK and does not provoke a lengthy healing response of the epithelium and superficial keratocytes, a history of keloid formation is not necessarily a contraindication to LASIK. In addition, a history of quiescent herpes simplex keratitis or autoimmune disease is less of a contraindication to LASIK compared to PRK.
Many early reports indicated that postoperative dry eye difficulties were more common with LASIK than PRK. More recently, with the implementation of femtosecond laser flaps, there is a lower incidence of dry eye, which may be attributed to the creation of thinner flaps, resulting in a greater residual stromal bed and decreased corneal denervation.17
Assessment of history, the tear meniscus, rose bengal, or lissamine green staining, and Schirmer testing (if indicated) are particularly important. Patients with dry eyes preoperatively should have aggressive artificial tear supplementation, and, as needed, be considered for more aggressive treatment such as topical cyclosporine and prophylactic placement of occlusive punctal plugs placed prior to LASIK, in addition to artificial tear supplementation, to limit keratopathy that can lead to irregularity of the flap postoperatively.
When evaluating the cornea prior to LASIK, it is particularly important to look for signs of an anterior basement membrane dystrophy that could predispose to epithelial defects with the microkeratome pass. These patients usually are best served having PRK or creating the LASIK flap by a femtosecond scanning laser.
Corneal topography must be performed to quantitate corneal cylinder and rule out the presence of forme fruste keratoconus,18 the early stages of frank ectasia such as the easily missed pattern of pellucid marginal degeneration,19 or contact lens–induced corneal warpage. Keratometry is important because corneas steeper than 48 D are more likely to have thin flaps or frank buttonholes (central perforation), and corneas flatter than 40 D are likely to have a smaller diameter flap and are at increased risk for free caps because of transection of the hinge. When there is increased risk of a thin flap, the surgeon should be mindful that reuse of the microkeratome blade in the second eye typically results in creation of a second flap that is 10 to 20 μ thinner than the first flap. These issues may be reduced or eliminated by the use a femtosecond laser to create a lamellar flap. Femtosecond flaps have an exceptionally low rate of epithelial defects, epithelial sloughing, incomplete flaps, irregular flaps, and buttonhole flaps compared to microkeratome flaps. Additionally, femtosecond flaps are more uniform than meniscus-shaped microkeratome flaps.10,20 One of the most significant advantages of femtosecond laser flaps is the accuracy of flap thickness.
In a study comparing the IntraLase femtosecond laser to two popular microkeratomes, the mean achieved flap thickness was more reproducible with the femtosecond laser, reducing the comparative risk of overly thick flaps.21 Sutton and Hodge22 reviewed accuracy and precision of LASIK flaps with the IntraLase femtosecond laser in 1,000 eyes and demonstrated 87.3% of eyes were within ±20 μm of the intended result. Ninety-eight percent of caps created with the 30-KHz laser were within ±20 μm compared to 74.8% in the 15-KHz group. This compares favorably with microkeratome flaps that have been shown to be inaccurate.10
Measurement of corneal thickness using pachymetry is especially important prior to LASIK because an adequate stromal bed must remain after subtracting both the thickness of the lamellar flap and the ablated tissue in order to avoid corneal ectasia.23 Most practitioners use a guideline of a minimum residual corneal bed thickness of 250 μ and at least 50% of the original corneal thickness, although this is a clinically derived figure and no absolute determinations of this figure have been made. Even 250 μ remaining in the stromal bed after ablation does not guarantee that postoperative corneal ectasia will not develop. Most cases of unexpected ectasia despite these precautions have been associated with LASIK flaps thicker than the nominal expected thickness. As a result, an increasing number of surgeons are using intraoperative pachymetry to determine the actual flap thickness. In calculating the likely residual stromal thickness, the surgeon must use the value for the intended total correction, not the value of the nomogram-adjusted refractive error that is placed in the computer. The true tissue ablation is closer to the value needed to achieve the refractive shift. A nomogram adjustment to a lower refractive error that becomes the input to the laser does not mean that there is less tissue removed, but rather, reflects consistent overcorrection in the absence of the nomogram adjustment. The nomogram reduction of the programmed input does not mean less tissue is removed, however!
In addition, after the LASIK procedure, excess corneal flattening reduces the quality of the optical performance of the eye and increases aberrations; as a rule of thumb, postoperative central power below about 33 to 35 D should be avoided because of the high amount of spherical aberration associated with an excessively flat cornea, a difficulty exacerbated by a large pupil under mesopic and scotopic conditions.
Ideally, all patients are measured preoperatively with a wavefront error detection device and, where appropriate, the laser ablation is modified to reduce postoperative aberrations. A recent survey of the American Academy of Cataract and Refractive Surgeons (ASCRS) showed that 90% of refractive surgeons use wavefront technology, with 56% using wavefront technology on 76% to 100% of patients.1
OPERATIVE TECHNIQUE
Preparation
Prior to the LASIK procedure, the excimer laser beam is tested for proper homogeneity and fluence in an identical fashion to PRK preparation. The microkeratome and vacuum unit are assembled, carefully inspected, and tested to ensure proper function. The importance of meticulous maintenance of the microkeratome cannot be overemphasized. A new blade is placed within the microkeratome and, in models that permit different heads for different thickness flaps, the desired head size verified. If using a femtosecond laser, the unit should be warmed up and allowed to run through its self-testing procedure. Additionally, the flap parameters should be reviewed including flap diameter, flap depth, and the degree of the side cut angle. The patient may receive a mild sedative such as oral diazepam 5 to 10 mg approximately 30 minutes prior to the procedure. Topical anesthetic drops are instilled, and the skin is prepped with povidone iodine or another skin antiseptic. Some surgeons drape the skin or lashes with a plastic drape or Steri-Strips, whereas others feel this is unnecessary and a potential source of material to jam the microkeratome. A lid speculum is placed that has a configuration to accommodate the suction device and the path of the microkeratome (Fig. 49-2). The cornea may be marked with an optical zone marker to assist in proper centration of the suction ring, and one or more periradial lines usually are placed to ensure proper realignment of the flap (Fig. 49-3). If a femtosecond laser is used, a lid speculum is usually not used until after the flap has been created. The femtosecond laser uses a docking platform to couple the laser to the patient’s eye. This effectively keeps the eyelids from entering the surgical field during flap creation. Generally, periradial ink lines are not necessary when a femtosecond is used for flap creation.
![]() Figure 49-2. A lid speculum is placed that has a shape to accommodate the path of the microkeratome. Eyelids, lashes, and drape must be kept outside the path of the microkeratome as well. |
Creation of the Flap
With a microkeratome, the suction ring usually is centered over the entrance pupil, but if using a suction ring that creates a flap of less than 9.5 mm in diameter, some surgeons prefer to skew it toward the hinge to ensure that the hinge will not be located within the laser treatment zone. The ring is selected to create a diameter larger than the ablation zone; this is particularly important for hyperopic corrections and astigmatic corrections, as well as wavefront-guided treatments, all of which typically involve large areas of ablation, sometimes as much as a 10-mm diameter. Once the ring is properly positioned, suction is activated. The intraocular pressure should be raised to greater than 65 mm Hg and verified, preferably with a pneumotonometer (Fig. 49-4) rather than the less precise plastic Barraquer applanator, because low pressure can result in a poor quality, thin, or incomplete flap. Prior to making the lamellar cut, the surface of the cornea is moistened with proparacaine containing glycerin or with nonpreserved artificial tears. Balanced salt solution is avoided at this point because of the possibility of creating mineral deposits within the microkeratome that can interfere with its proper function. The microkeratome then is placed on the suction ring with the cornea moistened (Fig. 49-5), and its path is checked to ensure that it is free of obstacles such as speculum, drape, or overhanging eyelid. The microkeratome then is activated, passing over the cornea (Fig. 49-6) until halted by the hinge-creating stopper, after which the microkeratome head is reversed off the cornea. Depending on the model, epithelial defects may be reduced by lowering the vacuum or discontinuing the suction during the reversal; other models require vacuum to remain at full pressure during reversal.
![]() Figure 49-4. The suction ring is placed, the vacuum activated, and the intraocular pressure is checked (a pneumotonometer is illustrated) to ensure the IOP is greater than 65 mm Hg. |
![]() Figure 49-6. After the corneal surface is moistened, the microkeratome is placed on the suction ring, the path is checked to ensure that it is free of obstacles, and the microkeratome cut is made. |
With a femtosecond laser, the docking platform suction ring also is typically centered over the pupil. After docking the cone and applanating the cornea, the surgeon can further shift centration electronically to the desired position. For example, in the IntraLase system, the surgeon can verify that appropriate applanation pressure has been achieved by the appearance of a green light. If a red light appears, the applanation pressure is too high and the laser will not fire. Also, it is very important to make sure that there is no meniscus peripherally, thereby achieving equal applanation of the cornea and a planar cut. The laser scanning process then is activated, and the patient is verbally reassured during the process to maintain a steady upward gaze.
The Excimer Laser Ablation System
The excimer laser system then is focused and centered over the pupil, and the patient is asked to look at the fixation light. The flap is reflected (Fig. 49-7), and the patient is asked to continue to fixate. If the surgeon wishes to check bed thickness, an ultrasonic pachymeter is applied at this point (Fig. 49-8). The lights in the room and laser may need to be adjusted to allow the patient to be able to continue to visualize the fixation light through the irregular stromal surface after the flap has been lifted. If moist, the stromal bed is dried with a microsurgical debris-free sponge (Fig. 49-9). The pupil tracking and iris registration systems, if present, usually are activated at this point, followed by application of the excimer laser ablation. With or without a pupil tracking device, the surgeon must monitor the patient to ensure that the patient’s fixation is maintained (Fig. 49-10). It is important to initiate the stromal ablation promptly, before excessive stromal dehydration has taken place. However, if centration is lost the ablation should be halted and fixation regained prior to finishing the treatment. The amount of ablation is dependent on each surgeon’s individual nomogram developed based on monitoring outcomes. Major variables that some but not all surgeons find to be important include the following: specific laser, individual surgeon, amount of correction, gender, and age.
![]() Figure 49-8. Some surgeons choose to perform pachymetry on the stromal bed to determine actual flap thickness achieved and the amount of tissue available for ablation. |
Flap Replacement
After the ablation is completed, the flap is reflected (Fig. 49-11). The interface is irrigated (Fig. 49-12) until any interface debris is eliminated, best visualized with oblique rather than coaxial illumination. The surface of the flap is stroked with a smooth instrument such as the irrigation cannula from the hinge to the periphery to ensure that wrinkles are eliminated and that the flap settles into its original position, as indicated by the radial marks made earlier and illustrated by the application of prednisolone acetate 1% suspension (Fig. 49-13). The endothelial pump will begin to secure the flap in position within several minutes. If a significant epithelial defect is present, a bandage contact lens should be placed. Once the flap is adherent, the lid speculum is carefully removed, taking care not to move the flap. Most surgeons place a drop of antibiotic and steroid over the eye at the conclusion of the procedure followed by placement of a clear shield or protective goggles. Some surgeons recheck the flap at the slitlamp approximately 15 to 30 minutes later to ensure it has remained in proper alignment.
![]() Figure 49-12. The interface is irrigated under oblique illumination to ensure that all debris is removed. |
Many surgeons instruct their patients to use topical antibiotics and steroids postoperatively for approximately 5 days. In addition, it is very important to keep the surface of the flap well lubricated in the early postoperative period. Patients are examined 1 day after surgery to ensure that the flap has remained in proper alignment and that there is no evidence of infection or excessive inflammation. In the absence of complications, the next examinations are typically approximately 1 week; 1 month; and 3, 6, and 12 months postoperatively.
OUTCOMES
LASIK studies vary considerably in the techniques used, degree of refractive error treated, length that patients are followed, and variables analyzed. This section summarizes the findings of some of the larger LASIK studies using modern techniques for the treatment of different degrees of myopia, as well as for astigmatism and hyperopia. As was the case with PRK results, this section cites only a few historically significant studies and emphasizes the typical range of outcomes encountered in current clinical practice.
Conventional Versus Wavefront Treatments
One of the most significant improvements in refractive surgery has been the development and implementation of wavefront-guided and wavefront-optimized treatments. Conventional (traditional) treatments are spherocylindrical only. Wavefront error measurement technology can be used diagnostically to quantitatively define aberrations affecting the quality of a patient’s vision.24 In addition, this technology is used to create ablation profiles customized for an individual patient, in an effort to reduce pre-existing aberrations and to reduce the induction of new aberrations. In addition to addressing higher-order aberrations that cause symptoms such as loss of contrast sensitivity and night-time haloes and glare,25 wavefront-guided treatments are reported to improve the accuracy of correcting the low-order aberrations of spherical error and astigmatism.26,27
Aberrations are most commonly expressed mathematically as Zernike polynomials, whose terms can be arranged in a pyramidal fashion (Fig. 49-14). Two of the most important aberrations are spherical aberration (Fig. 49-15), which commonly causes a perception of glare and halo, particularly at night, and coma, which causes a smear in a direction like the tail from a comet (Fig. 49-16).
Currently, wavefront-guided lasers approved in the United States include the Alcon LADARVision CustomCornea, AMO VISX S4 CustomVue, and the Bausch & Lomb Technolas 217z Zyoptix.
A wavefront-optimized laser uses theoretical wavefront data to determine the best overall tissue ablation plan rather than measurements specific to the patient. Wavefront-optimized treatments are designed to treat spherocylinder errors while reducing induced spherical aberration. The Alcon Allegretto Wave Light offers both wavefront-optimized and wavefront-guided treatments.
Trials of wavefront analysis-guided and optimized treatments generally report improvements in both visual acuity and quality of vision compared to conventional excimer treatments.
Traditional wavefront analysis involves measurement of the total wavefront error from the eye.
Most wavefront aberrations come from the cornea. Most recently, topography-guided treatments are being evaluated. Topography-based treatments are calculated from an ideal reference spherical cornea using videokeratography. Topography-guided treatments evaluate many more data points compared to wavefront-guided treatments. Topography-guided treatments may be beneficial in patients with highly aberrated corneal topographies and irregular astigmatism.
Low Myopia
Because PRK already had been judged adequate for patients with low to moderate myopia and did not include the risk of creating a corneal flap, LASIK was initially conceived as a procedure for myopia greater than 6 D. However, as LASIK techniques and microkeratome designs improved, many studies reported safety and efficacy of conventional LASIK at a level at least as high as PRK for correction of low myopia. Series of eyes treated with conventional LASIK for low myopia28,29,30,31 report 45% to 83% achieved uncorrected visual acuity of 20/20 or better, 85% to 100% achieved 20/40 or better, and 73% to 100% of patients obtained a postoperative refraction within 1.0 D of the intended refraction. In most studies no eyes lose two or more lines of best corrected vision.
Several early studies directly comparing conventional LASIK to PRK for low myopia have revealed similar safety and predictability but with higher patient satisfaction after LASIK. El-Maghraby et al32 found that, in a study of patients who had conventional LASIK in one eye and PRK in the other to correct -2.5 to -8.0 D, almost twice as many were highly satisfied with their LASIK eye as compared to their PRK eye 1 year after treatment. In addition to having less postoperative pain and more rapid visual recovery, the LASIK eyes were more likely to achieve an uncorrected visual acuity of 20/20 or better and had a more regular postoperative corneal topography. As techniques and technology continue to develop, reported results also have improved.33 Nevertheless, results of PRK often are reported to be equal to or superior to LASIK for low myopia.34
In its premarket approval application to the Food and Drug Administration, Alcon Autonomous compared the effectiveness of 139 eyes with wavefront-guided Custom ablations to 47 conventionally treated eyes at 6 months after surgery. All eyes had a preoperative myopic manifest refractive error less than -7.0 D sphere and less than 0.5 D cylinder. For wavefront-treated eyes, 79.9% achieved UCVA of 20/20 or better, 91.5% 20/25 or better, and 98.6% 20/40 or better. The manifest refraction spherical equivalent (MRSE) was ± 0.5 D in 74.8% and ±1.0 D in 95.7%. Aberrations were smaller at 6 months than preoperatively for 38% of Custom eyes compared to 14% of a healed flap edge by RMS increased by 0.08 μm (20%) in the Custom group and 0.33 μm (82%) in the conventional group. Spherical aberrations increase by 0.04 μm (22%) for Custom eyes and 0.23 μm (108%) for conventional eyes. No Custom patients reported significantly worse glare or haloes postoperatively and only one patient reported significantly worse night driving difficulty. Mean contrast sensitivity for Custom eyes improved by 0.1 to 0.2 log units relative to conventional eyes. Best spectacle-corrected vision (BSCVA) and low-contrast acuity were both slightly better for Custom eyes than conventionally treated eyes. More Custom eyes showed clinically significant increases than decreases, whereas more conventional eyes showed clinically significant decreases than increases in both of these measures.
A single-site comparison of the Alcon Custom Cornea and the Bausch and Lomb Zyoptix systems, reporting results at 1 month, showed similar excellent results, such as 93% and 90% rates of 20/20 UCVA and 80% and 70% 20/16 UCVA, respectively.35
A single-site comparison of Alcon CustomCornea and VISX CustomVue for eyes less than -8 D and cylinder less than 1.5 D yielded 98% and 95%, respectively, within 0.5 D of target.36
In a contralateral eye study, after a nomogram adjustment, the Alcon CustomCornea and VISX CustomVue results were 80% and 100%, respectively, within 0.5 D of goal.37
More recently, studies evaluating wavefront-guided and wavefront-optimized LASIK continue to show safety and efficacy. Schallhorn and Venter38 reviewed initial results of 32,569 eyes with wavefront-guided LASIK in eyes with low to moderate myopia using the VISX Star S4 excimer laser and found 94% of eyes within 0.5 D of emmetropia, UCVA of 20/20 in 92%, and UCVA of 20/40 in 99%. A report by the American Academy of Ophthalmology also concluded that wavefront-guided LASIK is safe and effective in myopia and myopia with astigmatism.39 Compared to conventional LASIK, wavefront-guided LASIK improved outcomes, specifically contrast sensitivity, night vision, and visual symptoms. Another review comparing the VISX Star S4, LADARVision 4000, and WaveLight Allegretto wavefront treatments versus conventional treatments showed efficacy and safety of LASIK for myopia and myopic astigmatism. Specifically, the VISX Star S4 wavefront treatments improved results compared to VISX Star S4 conventional treatments; however, LADARVision wavefront treatments did not improve the LADARVision conventional results. The Allegretto produced the best results in terms of visual acuity in myopic astigmatism eyes, and the VISX Star S4 induced the fewest higher-order aberrations in myopic and myopic astigmatism eyes.40 A contralateral comparison study of myopic astigmatism patients showed that wavefront-guided and wavefront-optimized treatments both resulted in excellent, predictable refractive results. Wavefront-guided LASIK induced less change in 18 of 22 higher-order Zernike aberrations as compared to wavefront-optimized, which change only spherical aberration. Wavefront-guided treatments also resulted in better contrast sensitivity compared to wavefront-optimized treatments.41 In a comparison of wavefront-guided versus wavefront-optimized LASIK on the Allegretto platform in myopic astigmatism eyes, there was no statistically significant difference with regard to visual acuity or refractive outcomes. The study did note that wavefront-guided treatments could be considered for patients with preoperative root-mean-square higher-order aberrations greater than 0.35 μm. However, in the study population (374 eyes), most eyes (83%) had RMS higher-order aberrations of less than 0.3 μm.42
Moderate Myopia
In patients with moderate myopia (approximately -6.0 to -12.0 D), large published series of conventional LASIK43,44,45,46,47,48,49 report that 26% to 57% of eyes achieve an uncorrected postoperative visual acuity of 20/20 or better, 55% to 85% reach at least 20/40, and 41% to 72% are within 1.0 D of intended correction. The percentage of patients losing two or more lines of best-corrected vision ranges from 0% to 3.5%. Alió et al50 reported excellent stability and efficacy of LASIK at 10 years; 73% of eyes were within ±1.00 D and 92% within ±2.00 D with a mean myopic regression of -0.12 ±0.16 D per year. No eyes developed ectasia.
Studies directly comparing LASIK to PRK for moderate myopia generally reveal that the long-term results are remarkably similar. However, LASIK eyes have faster visual recovery with better uncorrected visual acuity at 1 month. Also, two reports from a large multicenter randomized prospective trial29,30 showed that, although average outcomes appear similar between PRK and LASIK, more PRK eyes lost best-corrected vision than LASIK-treated eyes.
Wavefront treatment of moderate myopia has also shown excellent efficacy, predictability, and safety.38,51,52,53 A study comparing night driving performance in patients with moderate myopia showed that wavefront-guided LASIK significantly improved mean night driving visual performance compared to conventional LASIK.54 The VISX Star S4 laser was evaluated in patients with moderate myopia and resulted in a mean postoperative spherical equivalent of -0.18 ±0.43 D at 3 months. In this study, 27.9% of eyes were 20/15 or better and 58% of eyes were 20/20 uncorrected. Additionally, 82% of eyes were with 0.50 D and 97.6% were within 1.00 D of emmetropia. No eyes lost more that one line of BSCVA.51 Similar results were achieved with the VISX Star S4 with postoperative spherical equivalent of -0.33 ±0.55 D at 12 months; 64.0% were within ±0.50 D and 90% within ±1.00 D of intended correction at 12 months.53
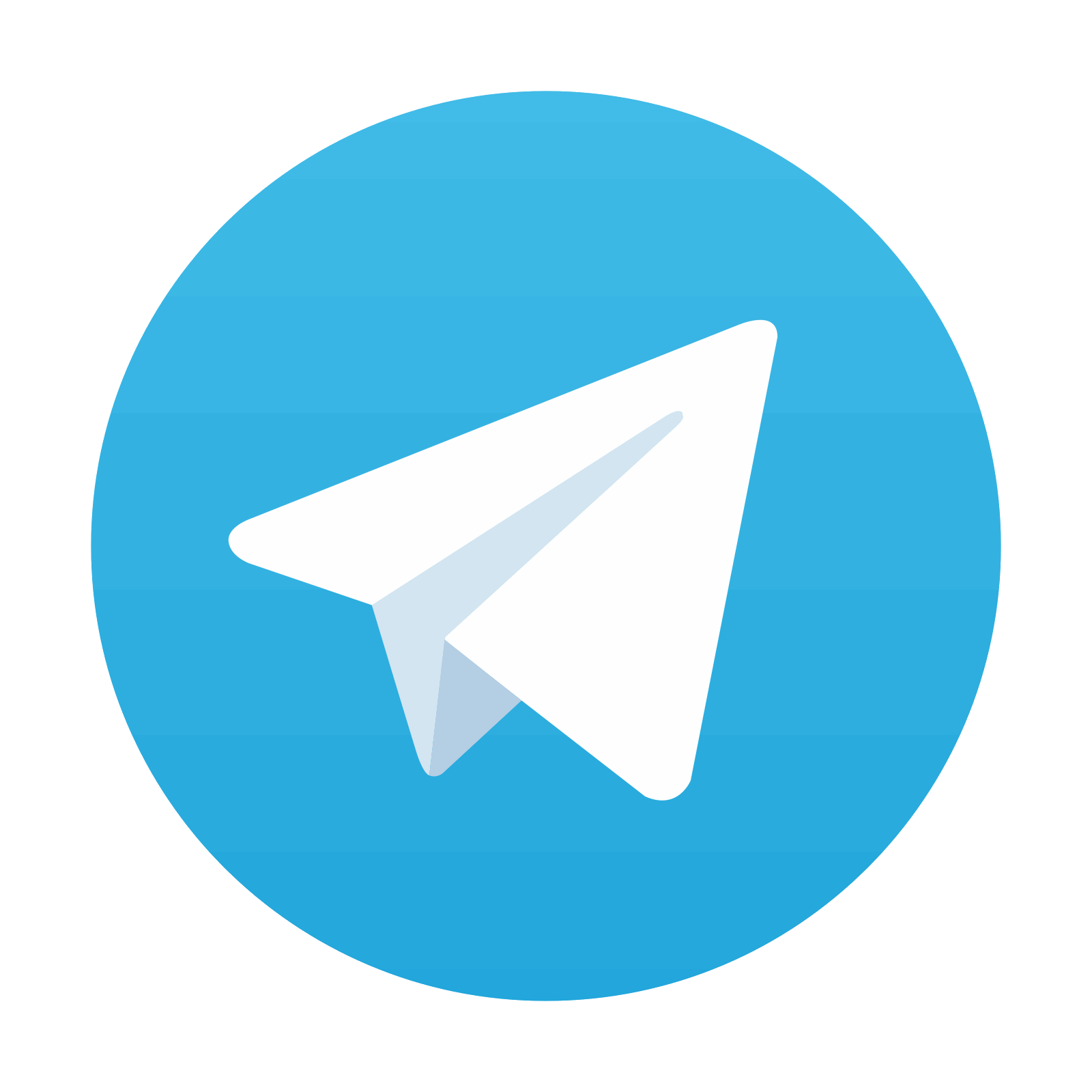
Stay updated, free articles. Join our Telegram channel

Full access? Get Clinical Tree
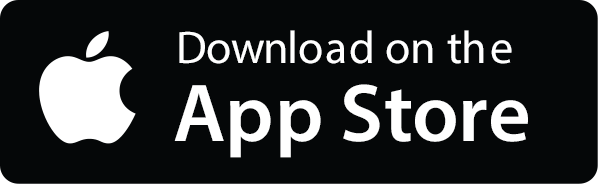
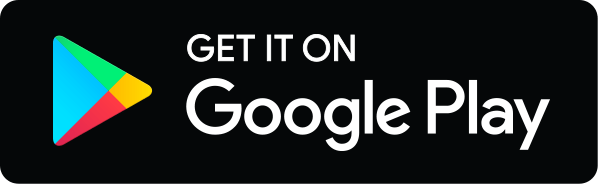