Chapter 9 The use of the laser in otosclerosis was considered by Sataloff in 1967, and in 1972 by Stahle et al (1980) presented a preliminary report of argon laser stapedotomy with excellent results. DiBartolomeo and Ellis (1980) reported on the argon laser for middle ear and external ear problems, again with good results. McGee (1983) reported the use of the laser in over 100 primary stapedectomies. McGee (1989) reported shorter hospital stays, less vertigo, and excellent hearing results as the advantages of laser stapedectomies. The single biggest advantage was the use of the laser in revision stapedectomy. For revision stapedectomy with mechanical instruments, air-bone closure to within 10 dB was achieved in fewer than half of all patients. The incidence of significant sensorineural hearing loss is 3 to 30%. The results of revision stapedectomy are significantly lower than the accepted standard for primary stapedectomy. The introduction of laser revision stapedectomy, however, has greatly improved results in terms of hearing and has reduced the incidence of permanent sensorineural hearing loss. The word laser is the acronym for light amplification by stimulated emission of radiation. Laser energy is derived from the release of photons that occurs when stimulated electrons return to their resting orbit. Albert Einstein stated that when photons of the appropriate wavelengths strike excited atoms, a second additional photon is released as the electron returns to its ground state. In this stimulated emission situation, both photons emitted from the excited atom have exactly the same frequency, direction, and phase, providing a laser beam that is collimated, coherent, and monochromatic. Lasers differ from one another principally in the lasing medium used, which determines the wavelength of light. The energy source, along with the mechanism to excite the laser medium and release its energy, determines the mode of the laser, which is generally continuous wave (CW), pulsed, or Q switched. Q switching involves a mirror system designed to interrupt the photon emission in the lasing medium, allowing it to build to higher levels, then releasing the emission in short pulses. Delivery of the energy may be by free beam or by focused beam, or the energy may be delivered by fiber or by contact fiber tip. The wavelength of the laser has important characteristics for tissue interaction. Lasers whose wavelength falls in the visible spectrum (about 380 to 700 nm) and the infrared (700 nm to 1 mm) of the electromagnetic spectrum are considered thermal lasers. On contact with tissue, the laser energy is converted to thermal energy, which causes a rapid increase in tissue temperature. The laser–tissue interaction depends on the nature of the tissue (i.e., bone, cartilage, etc.) as well as on the laser being used. Visible spectrum laser (argon and KTP) energy absorption by tissue is partially dependent on tissue color. For example, for soft tissue work, chromophores of hemoglobin and melanin absorb most of the energy. Tissues having a lighter color will reflect most of the energy from the laser. Energy absorption from the invisible carbon dioxide laser is primarily by intracellular and extracellular water, which is instantly converted to steam. The magnitude of the laser tissue interaction can be regulated by the laser’s power output, the power density, and the energy fluence. Power can be defined as the time rate at which energy is emitted and is expressed in watts. The power output is directly adjusted by the control panel on the laser console. Laser energy is delivered through a focusing lens. Power density is a measure of the intensity or concentration of the laser beam spot size. Power density can be expressed as the ratio of power to the surface area of the spot size and is expressed in terms of watts per square centimeter: Power density = power (watts)/area of spot size (cm2) Area of spot size is πr2, where r equals the spot size radius in centimeters. Thus, it can be seen that power density is inversely proportional to the square of the radius of the spot size. For any specific power output, changes in the spot size can have a tremendous effect on power density. Fluence can be expressed with the formula Fluence (joules) = power (watts) × exposure time (seconds)/area of spot size (cm2) As the fluence increases, so does the volume of affected tissue. The thermal energy having an impact on tissue rises dramatically as the time of exposure increases. There are four factors that determine laser–tissue interactions: 1. The laser wavelength (frequency) 2. The absorption characteristics of the tissue 3. The temporal parameters of the laser energy delivered 4. The mechanism of the laser delivery An incident beam of laser radiation on tissue can have one of the following effects: 1. Reflection 2. Absorption 3. Scattering 4. Transmission The ideal qualities of a laser for use during a stapedectomy are the following: 1. Precise optics are required to focus the laser beam at the place required. 2. The laser should be able to vaporize either the bone or the collagen at the oval window in a predictable manner. 3. The thermal footprint of the laser should not be beyond (deeper than) the site of application. If it did penetrate beyond the site of application, it would cause thermal damage to the utricle and the saccule beneath. 4. The laser should not heat the perilymph. The short wavelength lasers (argon = 488 or 514 nm; KTP = 532 nm) have ideal optical properties for microscopic surgery. The KTP laser is produced by passing an Nd:YAG laser output through a KTP crystal, which doubles the frequency (halves the wavelength) of the laser light. The KTP laser is generally a Q switched laser. The argon laser is produced by excitation of argon gas, yielding two possible laser bands at 488 nm (blue light) and 514 nm (green light). The light can be delivered from the laser source to the microscope-mounted micromanipulator through an optical fiber and can be focused to a spot size of 0.5 mm or smaller. The light of the aiming beam and the light of the working beam are the same spot size, and their point of impact is identical; that is to say, they are parfocal and coaxial. Initially, Perkins (1980) and McGee (1983) used microscope-mounted lasers. In 1990, Gherini and Horn introduced a handheld Endo-Otoprobe (HGM Medical Lasers Systems, Salt Lake City, UT) to deliver an argon laser beam; this provided further refinement to the convenient use of lasers in stapedectomy. Currently, long-wavelength carbon dioxide (CO2) laser light (10,600 nm) can only be transmitted to the microscope via a series of carefully aligned mirrors and lenses. The infrared CO2 laser is invisible to the naked eye, so a visible laser aiming beam is needed. For this purpose, a helium-neon laser is used. The CO2 laser is delivered in a CW mode and can also be used in a superpulsed mode (a chopped variation of CW) to produce higher peak energies but less thermal dissipation in tissue. The CO2 laser has near ideal tissue absorption characteristics because the laser energy is absorbed by water. The water component of bone, for example, is approximately 60%. At the high-power density setting, the pulsed laser vaporizes bone and releases heat and tissue into the vapor plume. Because the CO2 laser energy is absorbed by water, it does not penetrate. Disadvantages of the CO2 laser include the following: 1. The laser apparatus is cumbersome. 2. Increased working distance exists between the microscope and the operative site. 3. Diminished light through the microscope is common because of the use of the beam splitter. 4. The aiming beam and the working beam do not have the same wavelength, so it becomes difficult to focus both together at the same spot. In short, the CO2 laser can be said to have optimal tissue characteristics but suboptimal optical characteristics for use in stapedectomy (Poe 2000). The advantages of visible lasers include the convenience of the handheld probe and the fact that the spot size can be chosen accurately. Visible light lasers depend on char formation to begin creation of a rosette (fenestra) on the footplate. The char absorbs laser energy and creates heat. The laser energy can pass through (either directly or by scatter) and injure the neural tissue of the utricle or saccule. Presently, there is no ideal laser. The visible lasers, especially the argon Endo-Otoprobe, have excellent optical precision, superior to the CO2 laser. The CO2 laser, however, has superior interaction of laser energy with bone, collagen, and perilymph (Fig. 9–1). Fluency threshold studies confirm that 15 to 20 times more argon or KTP energy is required to ablate bone (Lesinski and Newrock 1993). Transmission spectroscopy studies of both collagen and bone further verified the selective absorption of infrared electromagnetic energy compared with visible light (DiBartolomeo 1981). Finally, thermocouple experiments show that, should correct energy parameters and surgical care during surgery be taken, both visible and invisible (infrared) lasers can be used safely for stapedectomy (Perkins 1980). Figure 9–1 (A) Schematic representation showing how the laser beam from the micromanipulator has the potential risk of damaging structures proximal and distal to the target because the rate of power density falloff on either side is small. (B) The Endo-Otoprobe has a larger angle of divergence and a rapid power density falloff, thus reducing the risk of damage to structures both proximal and distal. (From Friedman MD. Surgical management of otosclerosis: Primary stapedectomy surgery. Operative Techniques in Otolaryngology—Head and Neck Surgery. 1998;9(1):55. Used with permission.) A pulsed CO2 laser beam is ideal for revision stapedectomy because infrared lasers are well absorbed by collagen. Because visible lasers are poorly absorbed by collagen and pass readily through perilymph, they are less preferable for revision stapedectomy. Situations that are ideal for laser stapedectomy include Revision stapedectomy (Fig. 9–2) Obliterative footplate Primary stapedectomy is also an ideal situation because in stapedectomy the first surgery is the best chance for an optimal outcome. The advantages of laser stapedectomy include the following: 1. A precise fenestra can be created in the oval window. 2. Laser stapedectomy avoids trauma to the inner ear. The incidences of vertigo, sensorineural hearing loss, and tinnitus are much less likely. 3. A floating footplate is more likely to occur with manual stapedectomy where the surgeon attempts to create a fenestra without the use of a laser. The use of a laser lowers the chance of a floating footplate occurring. 4. Laser stapedectomy results in good hemostasis. 5.
Lasers in Otosclerosis
LASER PHYSICS AND PRINCIPLES
OPTICAL PROPERTIES
Visible Lasers
Infrared Lasers
ADVANTAGES OF THE CO2 LASER
DISADVANTAGES OF THE CO2 LASER
ADVANTAGES OF VISIBLE LASERS
DISADVANTAGES OF VISIBLE LASERS
WHICH LASER SHOULD BE USED FOR STAPEDECTOMY?
IDEAL SITUATIONS FOR LASER STAPEDECTOMY
ADVANTAGES OF LASER STAPEDECTOMY
Stay updated, free articles. Join our Telegram channel

Full access? Get Clinical Tree
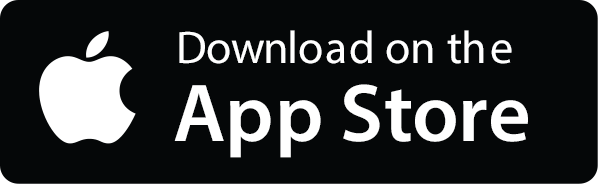
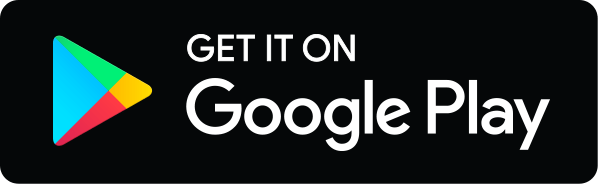