Definitions
The word “laser” is an acronym for light amplification by stimulated emission of radiation. A laser is a device that produces an intense beam by amplifying light.
The radiation produced for surgical lasers is in the electromagnetic spectrum with a wavelength that ranges from 200 to 400 nm (near-UV radiation), 400 to 700 nm (visible radiation), 700 to 1000 nm (near-infrared radiation), and more than 1000 nm (infrared radiation). The most prominent physical feature of the radiation is its wavelength, which determines its visibility. The three most commonly used types of surgical lasers are (1) the argon laser, which is within the visible portion of the electromagnetic spectrum; (2) the neodymium:yttrium–aluminum–garnet (Nd:YAG) laser; and (3) the carbon dioxide (CO2) laser.
Stimulated emission is the main source of laser energy. However, the energy of stimulated emission needs to be amplified to produce an intense beam. When the laser pump activates the active medium, the active medium starts having more atoms in an excited state. As atoms in the excited state release photons, this induces the emission of the photons from other atoms through a chain reaction.
One of the distinctive features of the light is its highly concentrated energy per unit area. Beams forming the light synchronously occur parallel with each other, which makes it possible for the laser to travel a certain distance without divergence. It is monochromatic. The wavelength of the light is one of the factors determining the physical characteristics of the laser and its interaction with tissue.
The current model of stimulated emission is described by quantum physics, which defines different energy levels of electrons while revolving around the nucleus in different levels of orbit. In this model, a stable electron in a normal state makes a transition to a higher but unstable energy level by absorbing a photon (absorption). This unstable electron with high energy ultimately may return to the original stable level spontaneously (spontaneous emission). Alternately, this emission can be induced by a forced interaction between one photon and the unstable electron to release a new photon (stimulated emission), which is the basis of laser energy.
Laser Components
A laser primarily consists of three main components: (1) an active medium; (2) a stimulation (excitation) mechanism, which is the power source or a laser pump; and (3) an optical chamber (feedback mechanism) (Figure 6–1). The active medium is the component where the laser radiation is generated. The function of the active medium is to supply a source of stimulated atoms, molecules, and ions. It may be in a solid, gaseous, or liquid state. Different types of lasers are named based on what is used as an active medium. Lasers with a solid state of active medium are the Nd:YAG, ruby, and diode lasers. Lasers using a gaseous active medium are the CO2, argon, and helium-neon lasers. The helium-neon laser is used as an aiming beam in lasers with an invisible beam (as in the CO2 laser) in order to create a visible beam. A laser with a liquid active medium uses organic dye.
The activation status of the laser medium is operated by the operation mode of the laser device. Three operational modes are available currently. In the continuous mode, the active medium is kept in a stimulated mode, which provides constant and stable energy. In the pulsed mode, the active medium is intermittently activated for a very short time, which allows tissue to cool off between pulses, thereby decreasing thermal damage. However, a much higher maximum of instantaneous energy is delivered with pulses compared with that of the continuous mode in which average power output is greater. In Q-switched mode, very short pulses of the laser are produced in a controlled manner. The second component of the laser is the power source that is used to activate the medium. The optical chamber is used to direct the output and also to provide feedback from amplification and collimation. The optical chamber contains the active medium.
Besides these major components of the laser, it must contain a cooling system, a delivery system, a control unit, and a remote control. Delivery systems are important in the selection of a laser. They can be an articulated arm (for the CO2 laser), optical fibers (for near-infrared and visible lasers), or a connection between the laser and the operation microscope (for the CO2 laser).
Commonly Used Lasers
The wavelength of the CO2 laser is 10,600 nm, which is not visible. Its power is between 0.1 and 100 W. A cooling system is required to couple to the main system because of the high heat energy produced by the laser. Also, the helium-neon laser beam is used as an aiming beam to make it visible. Its delivery system may be a handpiece at the end of an articulated arm consisting of reflective mirrors, a wave guide, or a micromanipulator to be coupled to an operating microscope. Practically, its energy is absorbed by the tissue within a 0.2-mm depth. Any tissue with a high water content selectively absorbs the CO2 laser. For an incision, a small spot size with a high power density is preferred. New CO2 laser systems have a spot size as small as 160 μm. For vaporization purpose, a low power density is applied with a large spot size. This also creates a heat energy that coagulates blood and lymph vessels. However, its hemostasis capability is limited to vessels <0.5 mm. In skin resurfacing, the CO2 laser ablates 20–60 μm of tissue and up to 150 μm of residual thermal damage per pass. Generally speaking, the CO2 laser is used for excision of laryngeal lesions and deep skin resurfacing for rhytids and acne scarring.
The argon laser is typically used for the coagulation of hemangiomas. Its beam emits a green-blue light, visible in the range of the electromagnetic spectrum (458–515 nm), and has a penetration depth of 1 mm. Because of its wavelength, it is almost completely absorbed by hemoglobin, melanin, and myoglobin.
The Nd:YAG laser is a solid-state laser that delivers a 1060-nm beam (near infrared), thereby requiring an aiming beam. The penetration depth is 3–5 mm because of its low absorption by water and tissue pigments. This low absorption also causes scattering and reflection. Therefore, its use for coagulation purposes requires high power, making the thermal coagulation of vessels and hemangiomas possible. Its delivery system is a fiberoptic carrier, which provides a hemostatic effect at contact. However, it can be used for ablation in a noncontact mode. The Nd:YAG laser is used for tracheobronchial lesions, particularly for its excellent hemostatic qualities; nonablative skin resurfacing; and hair removal in ethnic patient populations.
The KTP-532 (potassium titanyl phosphate) laser works by passing an Nd:YAG laser through a KTP crystal, resulting in the emission of half its wavelength (532 nm), which becomes visible. The delivery system is a fiberoptic carrier (for vaporizing and coagulation effects) or a contact quartz tip (for cutting). Since this laser is primarily absorbed by oxyhemoglobin, it is mainly used in the treatment of vascular lesions (including superficial skin lesions and telangiectasias) and the surgical reduction of turbinate tissue.
The erbium:YAG laser emits a 29–40 W, which is highly absorbed by water (12–18 times more efficiently than CO2 laser). It has the advantage of precise tissue ablation, 5–20 μm per pass, a small zone of residual thermal damage compared with the CO2 laser. The erbium:YAG laser has the disadvantage of poor hemostatic qualities and limited collagen tightening compared with the CO2 laser. It is primarily used for superficial skin resurfacing for fine wrinkles, brown spots, and acne scars.
Laser–Tissue Interaction
The effects of laser on tissue rely on one of the following interactions: absorption, scattering, transmission, or reflection (Figure 6–2). The type of interaction between a laser beam and any tissue is determined by the wavelength of the laser beam, the operation mode of the laser, the amount of energy applied, and tissue characteristics. The interaction of the laser on tissue can be summarized with the general statement that “the shorter the wavelength, the greater the effect on the tissue.” Table 6–1 shows lasers with their electromagnetic spectrum and penetration depth. Lasers whose wavelengths are within 0.1–0.8 μm (UV and visible region of the spectrum) cause minimal water absorption but considerable hemoglobin-melanin absorption. Lasers with a wavelength >3 μm absorb water.
Electromagnetic Spectrum | Laser Type | Wavelength | Penetration Depth |
---|---|---|---|
Visible lasers | Argon | 514 nm | 0.8 mm |
KTP-532 | 532 nm | 0.9 mm | |
Flashlamp-excited dye | 577 nm | 0.9 mm | |
Near-infrared lasers | Nd:YAG | 1060 nm | 4.0 mm |
Infrared lasers | Ho:YAG | 2100 nm | 0.4 mm |
Er:YAG | 2940 nm | 3.0 μm | |
CO2 | 10,600 nm | 30.0 μm |
The visible lasers penetrate into tissue at approximately 1 mm. However, the Nd:YAG laser goes into tissue at 4 mm and absorbs minimal water. In contrast, the penetration depth of the CO2 laser is only 30 μm, which makes it superb as a cutting tool.
Changes in tissue exposed to a laser relate to the temperature created by the laser. In temperatures higher than 50°C, enzymatic activity decreases. Protein denaturation occurs at temperatures over 60°C, the point at which physical changes are seen. However, tissue can still recover with the healing process. Over 80°C, the collagen degrades; 100°C is the temperature at which the water vaporizes, which results in expansion of the steam and finally tissue ablation. Although providing perfect hemostasis, a laser incision causes a delay in wound healing. Collateral thermal damage, although inevitable, can be minimized by using infrared lasers. Lasers can be used for incision, vaporization, or coagulation. Laser beams can be focused to spot sizes <1 mm in diameter or defocused. A focused beam is used for cutting and a defocused beam for ablation and coagulation.
Laser Safety Rules
Currently, there are two main federal regulations regarding the safe use of lasers in the United States. These are the American National Standard for Safe Use of Lasers (ANSI Z 136.1), which regulates the laser industry, and Safe Use of Lasers in Health Care Facilities (ANSI Z 136.3), which regulates the installation, operation, and maintenance of lasers in health care units. There is another standard, Safe Use of Lasers in Educational Institutions, which regulates safe use during educational activities (ANSI Z 136.5).
Lasers can harm not only the patient but also the surgeon and other personnel in the operating room. Laser system hazards can be related either directly to the effect of the beam on tissue, such as the retina, the corneas, or skin, or secondary conditions such as fire, electrocution, toxic waste, and plume radiation.
A primary concern of unsafe laser use is eye injury. It can occur as a result of direct exposure to either a laser beam or a reflected beam. The best protection is for all personnel to wear approved laser safety glasses. If possible, the patient also needs to wear the same eyewear. If it interferes with the operation field or the procedure, moistened sterile cotton eye pads with moistened towels or metallic eye protectors are needed to cover the eyelids. All operating room windows must be covered with an opaque material at the wavelength of the laser used. An ANSI-approved warning sign at the entrance to all operating rooms should be placed with protective eyewear for those who enter the operating room.
Plume hazards relate to plume radiation and plume content. Plume radiation occurs when the laser beam contacts the smoke plume, which is a by-product of laser beam use. The smoke plume results from heat effect of the laser beam. Some of the energy may shift in wavelength, resulting in secondary emission and often in the visible portion of the spectrum. This secondary emission can cause temporary blindness. Continuous or frequent smoke evacuation is the only solution. Plume content biohazard relates to the direct toxic effect of the plume. In addition to laser smoke elimination, a surgical mask can minimize this risk.
Laser systems may cause airway burns from an endotracheal tube fire when the laser beam strikes an endotracheal tube, which is made of PVC. Therefore, tubes wrapped with reflective tape or made of reflective metals are preferred. Likewise, surgical drapes made of flame-retardant material are advised. The immediate surgical field should be covered with saline-soaked towels.
These precautions for anesthetic procedures are important when the operation is performed on the larynx or trachea. A closed ventilatory system that is provided with a small-cuff endotracheal tube is preferred unless the tube obstructs the surgical view. This type of system reduces the possibility of an anesthetic gas leak into the operative field, where the laser beam is present. The cuff may be filled with methylene blue. Ventilation with a high concentration of O2 and nitrous oxide should be avoided. Jet ventilation is often necessary for glottic and subglottic lesions.
Web Sites
Lasers in Otology–Neurotology
Ear canal lesions that are treatable with lasers include chronic infections, tumors, atresia, scar tissue, and webs. Chronic skin infections of the ear canal may require surgical intervention if medical treatment fails. If the full removal of canal skin is necessary, the argon or KTP-532 laser with low power is used to spot weld the edges or corners of the skin graft, which holds them in position. If whole-skin removal is not required, weeping areas of canal skin can be cauterized under local anesthesia with the argon or KTP-532 laser. Either laser is set at 1.5–2.0 W with a 1-mm spot size.
Polyps or other suspicious soft tissue in the ear canal can be biopsied with the same laser systems. Currently, there is no benefit to using lasers in canal skin carcinoma. Subcutaneous fibrous tissue in acquired ear canal atresia or stenosis can be dissected and vaporized with good hemostasis. This helps to keep the skin of the ear canal intact. In transcanal tympanoplasty, skin incision of the ear canal can be made with the laser without compromising visibility.
Fenestration of the tympanic membrane can be performed with a laser (laser-assisted myringotomy) under local anesthesia as an alternative to cold-knife myringotomy under general anesthesia. A CO2 laser is set at 3–18 W with a single 100-ms pulse through a handheld otoscope or 200-mm objective of the microscope. A spot size of 2.0–2.6 mm is preferred. Diode laser was reported to be effective in this application as well. Myringotomy opening made by either laser may be used for endoscopic examination of the middle ear. Patients with a single attack of otitis media with effusion may be candidates for laser-assisted myringotomy instead of ventilation tube placement. However, a short duration (average 15 days) of myringotomy patency and recurrence should be kept in mind for chronic cases.
A minimally invasive treatment option called “laser contraction myringoplasty” was described for tympanic membrane atelectasis. In this technique, CO2 laser set at 0.1–1 W with a spot size of 0.2 mm is applied to the perimeter of the atelectasis. It has been reported that the laser causes tightening of tympanic membrane tissues, which reduces or eliminates atelectasis.
Repairing a tympanic membrane perforation with a laser by spot welding is still far from a routine clinical practice. Nonetheless, in recurrent perforations with unresponsiveness to tympanoplasty, welding the temporalis fascia into the anterior canal skin may be effective.
The KTP-532, argon, and CO2 lasers are useful in middle ear surgery. The CO2 laser has a small spot size (0.1–0.2 mm), which provides precision and safe handling.
Controversy exists as to which laser system is optimal; each has advantages and disadvantages. Visible lasers ensure the accurate aiming of the laser light. A handpiece eases the manipulation. The light of these lasers can pass through the overlying fluid environment. The spot size and power can vary by changing the distance between the tissue and the probe. The disadvantages of these lasers include the fact that their absorbency depends on pigment, which necessitates cautious work on white bones and tendons because of the possible inner ear damage. Therefore, in revision stapedotomy, visible lasers should not be used. In contrast, there is no inner ear damage risk in using the CO2 laser, given its depth of penetration. However, with the CO2 laser, the aiming and treatment beams need to be accurately aligned. The lack of penetration through liquid may cause the beam to be weakened if fluid is overlying the target tissue. Visible lasers have better optical precision but less ideal tissue characteristics, and the CO2 laser has better tissue characteristics but less ideal optical precision.
Recently, the Er:YAG laser was found safe in middle ear procedures, given its high absorption rate by water, weak penetration through the otic bone, and weak transmission through the perilymph. Laser applications in middle ear surgery are not without complications. Facial nerve injury, severe vertigo, chorda tympani burn, and hearing loss can occur.
Small glomus tympanicum tumors can be vaporized with good hemostasis. Visible lasers are advantageous in this application. Although not commonly used, granulation tissue in the mastoid cavity can be removed with the argon laser at 4–6 W on continuous mode.
Laser stapedotomy was first introduced in 1979. Since then, it has gained growing acceptance. Although there are studies showing no difference in the hearing gain between classic and laser stapedotomy, laser stapedotomy appears to be advantageous over classic stapedotomy. Laser stapedectomy eliminates mechanical trauma to the inner ear as well as minimizing the prerequisite fine-hand skills. It provides precision in surgery. Postoperative vertigo is also diminished. Its benefits become more obvious in revision surgery and obliterative otosclerosis. Laser stapedotomy can be performed under local or general anesthesia. The laser is first used on the stapes tendon, and then the posterior crus of the stapes is vaporized. The latter should be done as close to the footplate as possible. Either a rosette pattern of spots with a series of laser shots or 0.6-mm fenestra with a single shot is used in the center of the footplate. After every shot, the char is wiped away. If a series of shots is to be used, the firings of the laser beam should be a few seconds apart. Recommended laser settings are as follows: (1) the spot size is 0.15 mm for the CO2 laser and 0.20 mm for the KTP-532 and argon lasers; (2) the power is 1.5 W for the CO2 laser and 1.6 W for the KTP-532 and argon lasers; and (3) the pulse duration is 0.1 seconds for each. With laser stapedotomy, closure of the air–bone gap within 10 dB is obtained in 90–95% of cases. No superiority of any laser system over another is mentioned.
For cases with otosclerosis confined to the fissula ante fenestram only, a novel technique has been described, laser stapedotomy minus prosthesis (STAMP). The technique simply includes vaporization of anterior crus first and later anterior one-third of the footplate with a handheld probe of argon laser. Use of a prosthesis is not needed. If the otosclerosis is limited to the fissula ante fenestram only, this should free the remainder of the stapes. If so, the stapedotomy opening is sealed with adipose tissue. The technique may be converted to classic laser stapedotomy in appropriate cases. With this novel technique, it has been stated that high-frequency hearing (6–8 kHz) was better preserved compared with standard laser stapedotomy and also lasted as long as standard laser stapedotomy. Low incidence of refixation was also noteworthy.
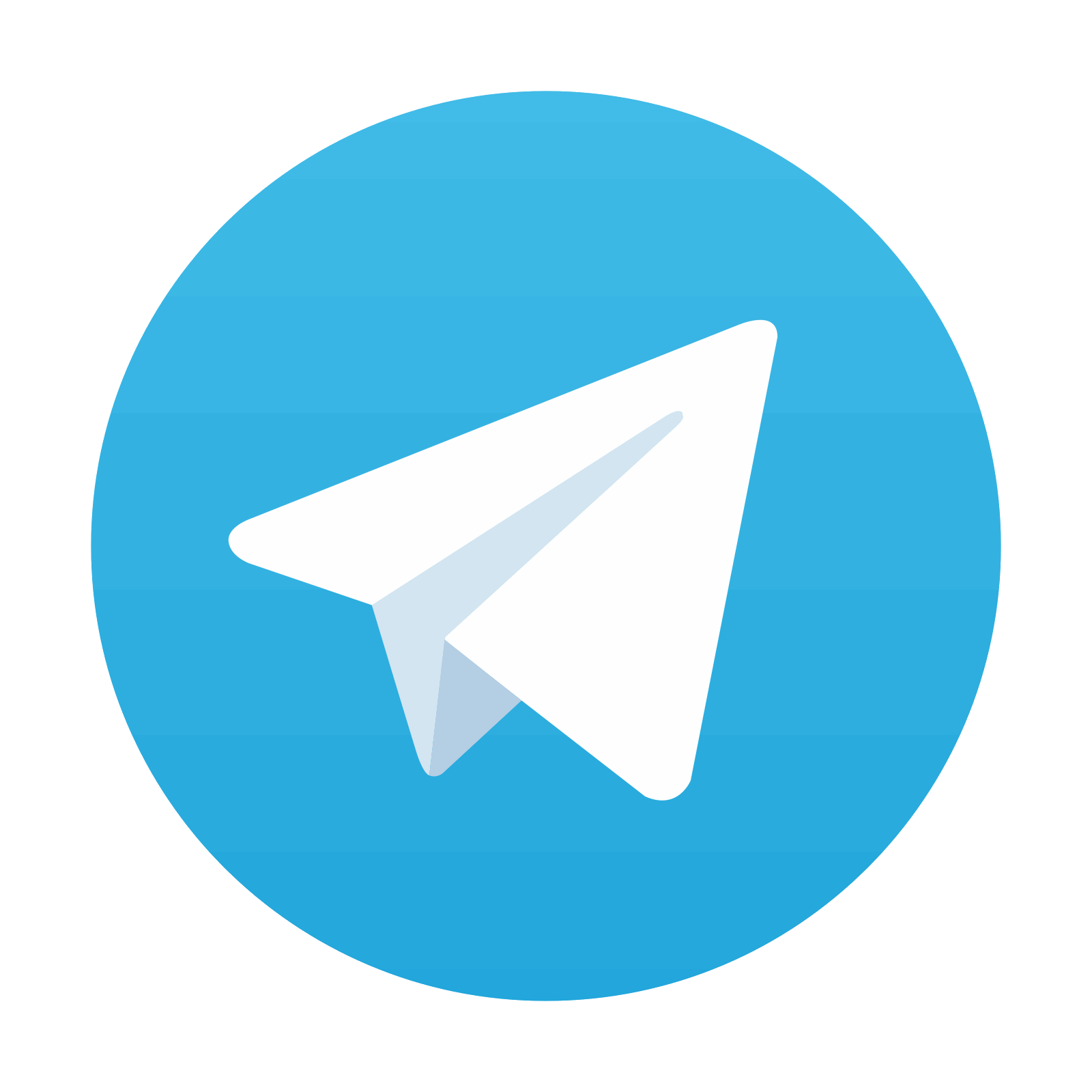
Stay updated, free articles. Join our Telegram channel

Full access? Get Clinical Tree
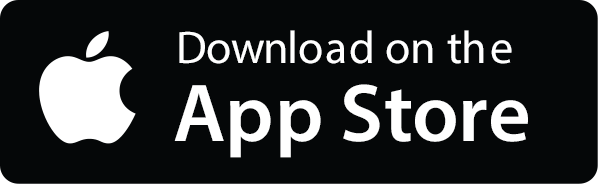
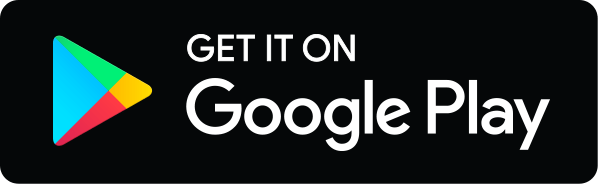