Laryngopharyngeal Reflux
Joel H. Blumin
Nikki Johnston
Reflux of gastric contents into the esophagus, gastroesophageal reflux (GER), is a normal physiologic phenomenon that occurs in most people, particularly after meals. Brief and infrequent exposure of the esophagus to gastric contents does not result in injury and disease, implying that there are intrinsic defense mechanisms that act to maintain mucosal integrity. In fact, based upon pH monitoring studies, up to 50 reflux episodes a day (below pH 4) are considered normal (1). It is thought that esophageal symptoms and complications arise when reflux is prolonged and/or there is a breakdown in the defense mechanisms. This is termed gastroesophageal reflux disease (GERD). GERD is accepted as possibly the most common chronic disease of adults in the United States affecting more than 30% of Western society (2).
When gastric reflux travels more proximal into the laryngopharynx, it is termed laryngopharyngeal reflux (LPR) (3). Other terms, such as gastropharyngeal reflux and esophagopharyngeal reflux (EPR), have been used synonymously. These are all considered as part of extraesophageal reflux (EER), reflux involving structures other than, or in addition to, the esophagus. LPR contributes to several otolaryngologic symptoms, inflammatory disorders, and perhaps also neoplastic diseases of the laryngopharynx (3,4,5,6,7,8,9) and appears to be as common in children and infants as adults (10). It is estimated that 10% of patients visiting otolaryngology clinic have reflux-attributed disease, and up to 55% of patients with hoarseness have reflux into their laryngopharynx (3,7). LPR is actually one of the most common factors associated with inflammation in the upper airways and is a common source of chronic laryngitis (3,4). Compared to the esophagus, where up to 50 reflux episodes (below pH 4) a day are considered normal or physiologic, the laryngopharynx appears to be more sensitive to injury by gastric refluxate. It has been shown experimentally that three reflux episodes per week or less into the laryngopharynx results in severe damage of the laryngeal epithelium (3). Recently published normative data utilizing impedance monitoring statistically demonstrated that one or more reflux events per 24-hour period are significant for diagnosis of LPR and that LPR events are indeed rare in an asymptomatic population (11).
Symptoms of LPR include dysphonia, throat clearing, postnasal drip, chronic cough, dysphagia, globus pharyngeus, excessive phlegm, heartburn, dyspnea, laryngospasm, and wheezing (12). Signs of LPR include those of laryngeal inflammation such as erythema and edema of the larynx, especially in the postcricoid and hypopharyngeal areas. Additional signs associated with LPR include vocal fold edema, diffuse laryngeal erythema and edema, pseudosulcus vocalis, obliteration of the laryngeal ventricles, thickened laryngopharyngeal mucus, and granulomata (12). One should consider that many of these signs are not specific to LPR and can be associated with other forms of laryngopharyngeal inflammation.
REVIEW OF GASTRIC REFLUX CONTENTS AND PHYSIOLOGY
Gastric Acid
Gastric acid is produced by parietal cells in the stomach. Parietal cells contain an extensive secretory network, called canaliculi, from which gastric acid is secreted into the lumen of the stomach. These cells are part of the fundic glands in the gastric mucosa. Gastric acid is approximately pH 2 in the human stomach lumen, the acidity being maintained by the proton pump H+/K+ ATPase. The resulting highly acidic environment in the stomach lumen causes proteins from food to denature, thus exposing the protein’s peptide bonds. The chief cells of the stomach secrete inactive enzymes pepsinogen and renin for protein breakdown. Gastric acid then activates pepsinogen into pepsin—this enzyme then helps digestion by breaking the
bonds linking amino acids, a process known as proteolysis. In addition, the acidic environment of the stomach inhibits growth of many microorganisms; the gut’s bacterial load is controlled and this helps prevent infection.
bonds linking amino acids, a process known as proteolysis. In addition, the acidic environment of the stomach inhibits growth of many microorganisms; the gut’s bacterial load is controlled and this helps prevent infection.
Secretion of Gastric Acid
Gastric acid secretion happens in several steps. Chloride and hydrogen ions are secreted separately from the cytoplasm of parietal cells and mixed in the canaliculi ultimately forming hydrochloric acid (HCl). This acid is then secreted into the lumen of the oxyntic gland and gradually reaches the stomach lumen. Chloride and sodium ions are secreted actively from the cytoplasm of the parietal cell into the lumen of the canaliculus. This creates a negative potential of −40 to −70 mV across the parietal cell membrane that causes potassium and sodium ions to diffuse from the cytoplasm into the parietal cell canaliculi. The enzyme carbonic anhydrase catalyzes the reaction between carbon dioxide and water to form carbonic acid. This acid immediately dissociates into hydrogen and bicarbonate ions. The hydrogen ions leave the cell through H+/K+ ATPase antiporter pumps. At the same time, sodium ions are actively reabsorbed. The majority of secreted potassium and sodium ions therefore return to the cytoplasm. The highest concentration that gastric acid reaches in the stomach is 160 mM in the canaliculi. This is about 3 million times that of arterial blood, but is isotonic with other bodily fluids. The lowest pH of the secreted acid is 0.8, but as the acid is diluted in the stomach lumen with other secretions, the intragastric pH will range between 1 and 3.
There are three phases in the secretion of gastric acid:
The cephalic phase: 30% of the total gastric acid produced is stimulated by anticipation of eating and the smell or taste of food.
The gastric phase: 60% of acid secreted is stimulated by the distension of the stomach with food. Digestion also produces proteins, resulting in even more gastrin production.
The intestinal phase: the remaining 10% of acid is secreted when chyme enters the small intestine, and is stimulated by small intestine distension.
Regulation of Secretion
Gastric acid production is regulated by both the autonomic nervous system and several hormones. The parasympathetic nervous system, via the vagus nerve, and the hormone gastrin stimulate the parietal cell to produce gastric acid, both directly acting on parietal cells and indirectly by stimulating secretion of the hormone histamine from enterochromaffin-like (ECL) cells. Vasoactive intestinal peptide, cholecystokinin, and secretin all inhibit production. The production of gastric acid in the stomach is tightly regulated by positive regulators and negative feedback mechanisms. Four types of cells are involved in this process: parietal cells, G cells, D cells, and ECL cells. In addition, the endings of the vagus nerve (CN X) and the intramural nervous plexus in the digestive tract also significantly influence secretion. Nerve endings in the stomach secrete two stimulatory neurotransmitters: acetylcholine and gastrin-releasing peptide. Their action is both direct on parietal cells and mediated through the secretion of gastrin from G cells and histamine from ECL. Gastrin acts on parietal cells directly and indirectly too, by stimulating the release of histamine. The release of histamine is the most important positive regulation mechanism of the secretion of gastric acid in the stomach. Its release is stimulated by gastrin and acetylcholine, and inhibited by somatostatin.
Neutralization of Acid
In the duodenum, gastric acid is neutralized by sodium bicarbonate. This also blocks gastric enzymes that have their pH optima in the acid range. The secretion of sodium bicarbonate from the pancreas is stimulated by secretin. This polypeptide hormone gets activated and secreted from S cells in the mucosa of the duodenum and jejunum when the pH in duodenum falls below 4.5 to 5.
This neutralization is described by the equation:
HCl + NaHCO3 → NaCl + H2CO3
The carbonic acid instantly decomposes into carbon dioxide and water, and is eliminated through the kidneys in urine.
Role of Gastric Acid in Disease
In hypochlorhydria and achlorhydria, there is low or no gastric acid in the stomach, potentially leading to problems as the disinfectant properties of the gastric lumen are decreased. In such conditions, there is greater risk of infections of the digestive tract (such as infection with Helicobacter bacteria). In Zollinger-Ellison syndrome and hypercalcemia, there are increased gastrin levels, leading to excess gastric acid production, which can cause gastric ulcers. Reflux of gastric acid into the esophagus and more proximally into the laryngopharynx and other extraesophageal sites also cause significant injury and disease—to be discussed in more detail later in this chapter.
Pharmacology
The H+/K+ pump enzyme is the target of proton pump inhibitors (PPIs), used to increase gastric pH in diseases that feature excess acid. Histamine H2 antagonists indirectly decrease gastric acid production. Antacids have high pH and neutralize existing acid.
Pepsin
Pepsin is expressed as a pro-form zymogen, pepsinogen, whose primary structure has an additional 44 amino
acids. In the stomach, chief cells release pepsinogen. The acid present in the stomach activates this zymogen. The hormone gastrin and the vagus nerve trigger the release of both pepsinogen and HCl when food is ingested. HCl creates the acidic environment that allows pepsinogen to unfold and cleave itself in an autocatalytic fashion, thereby generating pepsin, the active form of the enzyme. Pepsin then cleaves the 44 amino acids from pepsinogen creating more pepsin. Pepsin will digest up to 20% of ingested protein’s amide bonds by cleaving preferentially after the N-terminal of aromatic amino acids such as phenylalanine, tryptophan, and tyrosine. Pepsin exhibits preferential cleavage for hydrophobic, preferably aromatic, residues in P1 and P1′ positions. Increased susceptibility to hydrolysis occurs if there is a sulfur-containing amino acid close to the peptide bond, which has an aromatic amino acid. Pepsin cleaves Phe-Val, Gln-His, Glu-Ala, Ala-Leu, Leu-Tyr, Tyr-Leu, Gly-Phe, Phe-Phe and Phe-Tyr bonds in the B chain of insulin. Peptides may be further digested by other proteases in the duodenum and eventually absorbed in the intestine. Pepsin is stored as pepsinogen; so it will only be released when needed and does not digest the body’s own proteins in the stomach’s lining.
acids. In the stomach, chief cells release pepsinogen. The acid present in the stomach activates this zymogen. The hormone gastrin and the vagus nerve trigger the release of both pepsinogen and HCl when food is ingested. HCl creates the acidic environment that allows pepsinogen to unfold and cleave itself in an autocatalytic fashion, thereby generating pepsin, the active form of the enzyme. Pepsin then cleaves the 44 amino acids from pepsinogen creating more pepsin. Pepsin will digest up to 20% of ingested protein’s amide bonds by cleaving preferentially after the N-terminal of aromatic amino acids such as phenylalanine, tryptophan, and tyrosine. Pepsin exhibits preferential cleavage for hydrophobic, preferably aromatic, residues in P1 and P1′ positions. Increased susceptibility to hydrolysis occurs if there is a sulfur-containing amino acid close to the peptide bond, which has an aromatic amino acid. Pepsin cleaves Phe-Val, Gln-His, Glu-Ala, Ala-Leu, Leu-Tyr, Tyr-Leu, Gly-Phe, Phe-Phe and Phe-Tyr bonds in the B chain of insulin. Peptides may be further digested by other proteases in the duodenum and eventually absorbed in the intestine. Pepsin is stored as pepsinogen; so it will only be released when needed and does not digest the body’s own proteins in the stomach’s lining.
Pepsin is maximally active at pH 2, but has activity up to pH 6.5. While inactive at pH 6.5 and above, it remains stable to pH 8. The enzyme is not irreversibly inactivated (denatured) until pH 8. While the stomach is designed to resist damage by pepsin, reflux of pepsin into the esophagus and laryngopharynx causes damage even above pH 4. Pepsin is considered an important etiologic factor in reflux disease of the aerodigestive tract and a biomarker for reflux, whose levels and acidity can be related to the severity of damage.
Bile
Bile is produced by hepatocytes in the liver, draining through the many bile ducts that penetrate the liver. During this process, the epithelial cells add a watery solution that is rich in bicarbonates that dilutes and increases alkalinity of the solution. Bile then flows into the common hepatic duct, which joins with the cystic duct from the gallbladder to form the common bile duct. The common bile duct in turn joins with the pancreatic duct to empty into the duodenum. If the sphincter of Oddi is closed, bile is prevented from draining into the intestine and instead flows into the gallbladder, where it is stored and concentrated up to five times its original potency between meals. This concentration occurs through the absorption of water and small electrolytes, while retaining all the original organic molecules. Cholesterol is also released with the bile, dissolved in the acids and fats found in the concentrated solution. When food is released by the stomach into the duodenum in the form of chyme, the duodenum releases cholecystokinin, which causes the gallbladder to release the concentrated bile to complete digestion.
The human liver can produce close to one litre of bile per day. About 95% of the salts secreted in bile are reabsorbed in the terminal ileum and reused. Blood from the ileum flows directly to the hepatic portal vein and returns to the liver where the hepatocytes reabsorb the salts and return them to the bile ducts to be reused, sometimes two to three times with each meal.
Action of Bile Salts in Digestion
Bile acts to some extent as a surfactant, helping to emulsify the fats in food. Bile salt anions have both a hydrophilic and hydrophobic side, and therefore tend to aggregate around droplets of fat (triglycerides and phospholipids) to form micelles, with the hydrophobic sides toward the fat and hydrophilic to the outside. The hydrophilic sides are positively charged due to the lecithin and other phospholipids that compose bile, and this charge prevents fat droplets coated with bile from reaggregating into larger fat particles. Ordinarily, the micelles in the duodenum have a diameter of around 14 to 33 µm.
The dispersion of food fat into micelles thus provides an increased surface area for the action of the enzyme pancreatic lipase, which digests the triglycerides, and is able to reach the fatty core through gaps between the bile salts. A triglyceride is broken down into two fatty acids and a monoglyceride, which are absorbed by the villi on the intestine walls. After being transferred across the intestinal membrane, fatty acids are reformed into triglycerides, then absorbed into the lymphatic system through lacteals. Without bile salts, most of the lipids in the food would be passed out in feces, undigested.
Since bile increases the absorption of fats, it is an important part of the absorption of the fat-soluble substances, such as the vitamins D, E, K, and A. Besides its digestive function, bile serves also as the route of excretion for bilirubin, a by-product of red blood cells recycled by the liver.
The alkaline bile also has the function of neutralizing any excess stomach acid before it enters the ileum, the final section of the small intestine. Bile salts also act as bactericides, destroying many of the microbes that may be present in the food.
Role of Bile in Reflux Disease
Reflux of bile is known to cause esophagitis and is likely to play a role in reflux-attributed laryngeal injury and disease (13). The mechanism of bile-induced mucosal injury is thought to be related to “intramucosal trapping” of bile acids that results in mucosal damage primarily by disorganizing membrane structure or interfering with cellular metabolism. Taurocholic acid does this at pH 2 and chenodeoxycholic acid at pH 7. Some bile acids cause damage at high pH due to their lipophilic properties at the pH at or near the pKa value. For example, taurocholic acid (which is a conjugated bile acid with a pKa ˜2) and
chenodeoxycholic acid (which is an unconjugated acid with pKa ˜7) are unionized and therefore can enter the cell at an acidic and neutral pH, respectively. Once inside the cell, bile acids are trapped by ionization and subsequently cause mucosal damage.
chenodeoxycholic acid (which is an unconjugated acid with pKa ˜7) are unionized and therefore can enter the cell at an acidic and neutral pH, respectively. Once inside the cell, bile acids are trapped by ionization and subsequently cause mucosal damage.
Trypsin
Trypsin is secreted into the duodenum, where it acts to hydrolyze peptides into their smaller building blocks— amino acids (these peptides are the result of the enzyme pepsin breaking down the proteins in the stomach). This enables the uptake of protein in the food because peptides (though smaller than proteins) are too big to be absorbed through the lining of the ileum. Trypsin catalyzes the hydrolysis of peptide bonds.
Trypsin is produced in the pancreas in the form of inactive zymogen, trypsinogen. When the pancreas is stimulated by cholecystokinin, it is then secreted into the small intestine. Once in the small intestine, the enzyme enteropeptidase activates it into trypsin by proteolytic cleavage. The resulting trypsins themselves activate more trypsinogens (autocatalysis), so only a small amount of enteropeptidase is necessary to start the reaction. This activation mechanism is common for most serine proteases, and serves to prevent autodigestion of the pancreas.
Involvement of Trypsin in Reflux Disease
PATHOPHYSIOLOGY OF LPR
Gastric refluxate, containing acid, pepsin, bile, and trypsin, obviously passes through the esophagus (as in GER) and enters the laryngopharynx (as in LPR), yet patients with laryngeal symptoms and injury do not often have esophageal symptoms or injury. It is thought that LPR patients have intact esophageal defense mechanisms that prevent esophageal injury by the refluxate. For example, in the esophagus, peristaltic motility helps clear the refluxate, salivary bicarbonate neutralizes the refluxate, and mucus secretions prevent the refluxate from penetrating the epithelium. In addition, the upper esophageal sphincter (UES) closes to prevent refluxate from entering the laryngopharynx. It is thought that UES function is defective in many patients with reflux-attributed laryngeal symptoms and endoscopic findings. The larynx lacks many of the intrinsic defense mechanisms present in their esophageal counterparts, perhaps explaining its increased sensitivity to gastric refluxate compared to the esophagus.
Reflux is thought to cause laryngeal symptoms and induce extraesophageal pathology by several different mechanisms. First, by direct contact of acid and pepsin with the epithelium—the microaspiration theory (4). Second, the trauma theory suggests that exposure of the laryngeal epithelium to gastric refluxate alone is not sufficient to cause injury and that an additional factor, such as vocal abuse or concomitant viral infection, is necessary to induce mucosal lesions (3,16). Finally, the esophageal-bronchial reflex theory suggests that acid in the distal esophagus stimulates vagally mediated reflexes that cause chronic cough, which in turn causes laryngeal symptoms and lesions (17).
Differences between LPR and GERD
Ossakow et al. (18) compared symptoms of patients with reflux esophagitis with those of patients with laryngitis. They found that hoarseness was the most prevalent symptom of patients with LPR (100%), although none of the patients with GERD reported experiencing hoarseness. Heartburn was present in the majority of patients with GERD (89%), but only a small percentage of LPR patients (6%). Wiener et al. (19) demonstrated abnormal pH studies in 78% of patients with hoarseness, all of whom had normal esophageal manometry, and of these, 72% had normal endoscopy with biopsy. This supports the theory that the majority of LPR patients have normal acid clearance mechanisms (20). Although both esophagitis and laryngitis are likely to be caused by the damaging effects of the corrosive refluxate, significantly more damage occurs in the larynx compared to the esophagus following exposure to acid and pepsin. This may be because the esophagus has better defense mechanisms to counteract such damage, for example, peristalsis, saliva, and bicarbonate production (3,21). Therefore, although there may not be sufficient reflux to cause esophagitis, it may be enough to develop symptomatic LPR.
The pattern and mechanism of LPR and GERD are different. LPR patients typically have upright (daytime) reflux with good esophageal motor function and no esophagitis, whereas GERD patients have supine (nocturnal) reflux and esophageal dysmotility (3,22,23). GERD patients often have a dysfunction of the lower esophageal sphincter (LES), while the UES is a primary defect in LPR patients (24). The different pattern and mechanism of reflux may explain the different clinical manifestations observed in LPR and GERD.
Role of Nonacid and Weakly Acid Reflux in Laryngeal Inflammation and Disease
Studies using combined multichannel intraluminal impedance with pH monitoring (multichannel intraluminal impedance-pH [MII-pH]; discussed later in this chapter) reveal a positive symptom association with nonacidic
reflux and a significant association between nonacidic and weakly acidic reflux and persistent symptoms on PPI therapy (25,26,27). Using MII-pH monitoring, Tamhankar et al. (25) showed that PPI therapy decreases the H+ ion concentration in the refluxed fluid, but does not significantly affect the frequency or duration of reflux events. Kawamura et al. (28) reported on a comparison of GER patterns in 10 healthy volunteers and 10 patients suspected of having reflux-attributed laryngitis. Using a bifurcated MII-pH reflux catheter, the investigators found that gastric reflux with weak acidity (above pH 4), is more common in patients with reflux-attributed laryngitis compared to healthy controls. Oelschlager et al. (29) demonstrated that the majority of reflux episodes into the pharynx are in fact nonacidic. Sharma et al. (27) reported that 70/200 (35%) patients on at least twice daily PPI had a positive symptom index for nonacidic reflux. Finally, Tutuian et al. (26) also recently reported that reflux episodes extending proximally are significantly associated with symptoms irrespective of the pH of the refluxate. Patients with signs and symptoms associated with nonacidic and weakly acidic reflux would likely have a negative pH test and would not benefit from PPI therapy. Diagnosis and treatment has focused on the acidity of the refluxate because it was thought that the other components of the refluxate would not be injurious at higher pH. However, it is now known that certain bile acids are injurious at higher pH (30,31), and recent data supports a role for pepsin in reflux-attributed laryngeal injury and disease, independent of the pH of the refluxate (32,33). Given these data, it seems that a treatment regimen based on acid suppression alone would be bound to fail. Much of the controversy that has revolved around LPR management can easily be explained by this inflammation caused by weakly or nonacidic agents.
reflux and a significant association between nonacidic and weakly acidic reflux and persistent symptoms on PPI therapy (25,26,27). Using MII-pH monitoring, Tamhankar et al. (25) showed that PPI therapy decreases the H+ ion concentration in the refluxed fluid, but does not significantly affect the frequency or duration of reflux events. Kawamura et al. (28) reported on a comparison of GER patterns in 10 healthy volunteers and 10 patients suspected of having reflux-attributed laryngitis. Using a bifurcated MII-pH reflux catheter, the investigators found that gastric reflux with weak acidity (above pH 4), is more common in patients with reflux-attributed laryngitis compared to healthy controls. Oelschlager et al. (29) demonstrated that the majority of reflux episodes into the pharynx are in fact nonacidic. Sharma et al. (27) reported that 70/200 (35%) patients on at least twice daily PPI had a positive symptom index for nonacidic reflux. Finally, Tutuian et al. (26) also recently reported that reflux episodes extending proximally are significantly associated with symptoms irrespective of the pH of the refluxate. Patients with signs and symptoms associated with nonacidic and weakly acidic reflux would likely have a negative pH test and would not benefit from PPI therapy. Diagnosis and treatment has focused on the acidity of the refluxate because it was thought that the other components of the refluxate would not be injurious at higher pH. However, it is now known that certain bile acids are injurious at higher pH (30,31), and recent data supports a role for pepsin in reflux-attributed laryngeal injury and disease, independent of the pH of the refluxate (32,33). Given these data, it seems that a treatment regimen based on acid suppression alone would be bound to fail. Much of the controversy that has revolved around LPR management can easily be explained by this inflammation caused by weakly or nonacidic agents.
Antireflux surgery, such as fundoplication, is one of the few options for patients with persistent reflux-attributed symptoms and endoscopic findings. Unlike medical therapy, which does not stop reflux from occurring but only increases the pH of the refluxate, surgical therapy restores the physiologic separation between the abdomen and thorax and strengthens the LES, preventing refluxate from coming up the esophagus. Iqbal et al. (34) recently reported a study in which 85% of patients who had a fundoplication for extraesophageal symptoms of reflux had a positive outcome. The majority of these patients had refractory symptoms on maximal acid suppression therapy. These data additionally question the role of acid alone in the development of reflux-related pathology and highlight a potential role for the other components of the refluxate.
A role for pepsin in nonacid reflux has been postulated in the recent literature. As already stated, this enzyme is maximally active at pH 2, but can cause tissue damage above this pH, with complete inactivation not occurring until pH 6.5 (33,35,36). While pepsin is inactive at pH 6.5, it remains stable until pH 8 and thus can be reactivated when the pH is reduced. Pepsin is not irreversibly inactivated until pH 8 (33,36). Thus, even if the pepsin, which has been detected in, for example, laryngeal epithelia, is inactive (37,38) (mean pH of the laryngopharynx is 6.8), it would be stable and thus could sit inactive/dormant in the laryngopharynx and have the potential to become reactivated by a decrease in pH, either by a subsequent acid reflux event or perhaps once taken up by laryngeal epithelial cells and transported through intracellular compartment of lower pH (33,39,40,41).
Johnston et al. described how pepsin causes laryngeal epithelial cell damage at pH 7, and thus in nonacidic refluxate. These data may help explain why some patients have refractory symptoms on maximal PPI therapy, and help explain the reported symptom association with nonacidic reflux events. The authors report mitochondrial and Golgi damage in laryngeal epithelial cells exposed to pepsin at pH 7 (40). Cell toxicity was also demonstrated using the MTT cytotoxicity assay. Pepsin at pH 7 was also found to significantly alter the expression levels of multiple genes implicated in stress and toxicity. Of most clinical significance, they report that pepsin (0.1 mg/mL, pH 7) induces a proinflammatory cytokine gene expression profile in hypopharyngeal FaDu epithelial cells in vitro similar to that which contributes to disease in GER patients (42). Collectively, these data suggest a mechanistic link between exposure to pepsin, even in nonacidic refluxate, and cellular changes that lead to laryngopharyngeal inflammatory disease. In this context, the unexpected finding that pepsin is taken up by human laryngeal epithelial cells by receptormediated endocytosis is highly relevant. Pepsin has been previously assumed to cause damage by its proteolytic activity alone, but the discovery that pepsin is taken up by laryngeal epithelial cells by receptor-mediated endocytosis opens the door to a new mechanism for cell damage. It is possible that inactive, but stable, pepsin at pH 7 taken up by laryngeal epithelial cells becomes reactivated once inside the cell in compartments of lower pH such as late endosomes and the trans-reticular golgi where pepsin presence has been confirmed (32). The therapeutic potential of receptor antagonists and irreversible inhibitors of peptic activity to prevent pepsin uptake and/or reactivation are currently being studied.
OTHER CLINICAL MANIFESTATIONS OF EER
EER has been implicated as a source or cofactor of inflammatory disease of the mucosa of the entire head and neck. Subsites affected by reflux include mucosa of the nose, paranasal sinuses, eustachian tube and middle ear, nasopharynx, oropharynx, hypopharynx, larynx, subglottis, trachea, and lower airway. Connection of EER to specific disease states has been demonstrated in an ever expanding list of conditions of the aerodigestive tract including otitis media (OM), sinusitis, cough, sleep disordered breathing,
laryngitis, laryngospasm, airway stenosis, and lower airway problems such as asthma, chronic obstructive pulmonary disease, interstitial pulmonary fibrosis, and chronic lung transplant rejection. Evidence for these has been based largely on clinical findings that correlate to pH probe studies confirming EER or detection of elements of refluxate in the subsite in question. Animal and basic science studies have been used to propose or confirm a mechanism, but in most cases, direct cause and effect in the human condition has yet to be confirmed. Mechanism for disease is typically explained as occurring either by direct contact of refluxate and resulting inflammation of the mucosa or via a vagally mediated neurogenic process as previously discussed.
laryngitis, laryngospasm, airway stenosis, and lower airway problems such as asthma, chronic obstructive pulmonary disease, interstitial pulmonary fibrosis, and chronic lung transplant rejection. Evidence for these has been based largely on clinical findings that correlate to pH probe studies confirming EER or detection of elements of refluxate in the subsite in question. Animal and basic science studies have been used to propose or confirm a mechanism, but in most cases, direct cause and effect in the human condition has yet to be confirmed. Mechanism for disease is typically explained as occurring either by direct contact of refluxate and resulting inflammation of the mucosa or via a vagally mediated neurogenic process as previously discussed.
Extraesophageal Reflux and the Middle Ear
It has been proposed that refluxate that reaches to the nasopharynx can induce an inflammatory cascade that leads to middle ear dysfunction and OM. This can occur from direct inflammation and obstruction of the eustachian tube or by flow through the eustachian tube into the middle ear (43,44). Ambulatory pH probe studies of children with OM show a high rate of acid reflux (45). The presence of pepsin within the middle ear has also been shown in children with OM (46,47). The relatively neutral pH environment of the middle ear has led to criticism of the role of reflux in OM. Data showing presence of bile acids in effusions (48) as well as the recent expansion of our understanding of pepsin-induced mucosal injury at weakly or nonacidic pH provides another possible explanation (49). There have been limited evaluations of the effects of acid suppressive treatment in OM. In two small studies (50,51), there were significant clearing of effusions with antireflux therapy alone.
Extraesophageal Reflux and the Nose and Paranasal Sinuses
Although an association between sinonasal disease and gastric hypersecretion was first documented in 1950, only more recently has the awareness of this association been more fully investigated. In the late 1990s, several studies revealed a high association between EER and sinonasal disease. DiBaise et al. (52) performed pH probe studies on a group of patients with chronic rhinosinusitis (CRS) and noted 78% of patients had evidence of reflux. A larger epidemiologic study by El-Serag et al. (53) demonstrated that adults with GER were more likely to also have a history of sinusitis. In another study, patients with sinusitis were evaluated for reflux and those with objective findings of reflux were treated with acid suppression with a PPI. The majority of patients in this small noncontrolled study demonstrated improvement in their sinonasal symptoms following treatment for reflux. More recent studies of acid detection by pharyngeal probe have demonstrated its presence in the nasopharynx and multiple studies have demonstrated a high prevalence of GER with sinusitis in the pediatric population; however, cause and effect have yet to be proved (54).
The mechanism for disease is similar to other sites of the head and neck and can be explained by direct mucosal contact of refluxate or via a vagally mediated neurogenic process. With respect to the neurogenic mechanism, abnormalities of the autonomic system have been demonstrated in those with sinusitis and reflux. These abnormalities have been proposed to affect sinonasal mucosal edema and ostial function (55).
As with OM, there have been attempts to evaluate the effect of acid suppression and CRS (56). Results have been positive, but study design has been questioned. Bothwell noted that children with medically refractive CRS experienced relief of symptoms and avoided operation after acid suppressive therapy (57). Patients with CRS and a positive pH probe study tend to respond better to acid suppressive therapy then their cohorts without positive pH studies (52,58).
Extraesophageal Reflux and Stenotic Lesions of the Larynx and Airway
Stenotic lesions of the larynx and trachea continue to be a challenge to the otolaryngologist and patient. Although many of these lesions are posttraumatic or related to collagen-vascular disease, a subset remains idiopathic. Reflux has long been suggested to have a significant role in development of and worsening of laryngotracheal stenosis (LTS).
Bogdasarian and Olson (59) first suggested that reflux of gastric acid might play a significant role in the cause of posterior glottic stenosis. Later, Bain et al. (60) reported a case of subglottic stenosis (SGS) in a 57-year-old woman without a history of operation, trauma, or intubation. Her preoperative airway was described as being the size of a pencil eraser and then 5 months after a Nissen fundoplication and endoscopic airway management, her airway returned to a normal caliber. Little et al. (61) reported a case of a 12-year-old girl with recalcitrant SGS who continued to produce exuberant granulation tissue. During an endoscopic procedure, she was noted to have gastric contents in her larynx and hypopharynx. This patient was then treated with H2-receptor blockers and antireflux behavioral management; she was subsequently decannulated and there was no further formation of granulation tissue.
In Koufman’s widely cited manuscript on the description of EER, 225 patients with a variety of laryngeal disorders were systematically evaluated for reflux (3). Ambulatory 24-hour pH monitoring was preformed in 182 patients of whom 32 had LTS. Over three-quarters of those with LTS had abnormal pH studies. This group also had the highest rate of acid reflux identified of the seven categories of EER that were evaluated. In 1997, Little et al. (10) evaluated 222 children with a 24-hour dual probe pH monitoring.
About one-quarter of these patients had laryngeal findings and those with laryngomalacia or laryngeal stenosis had the highest number of pharyngeal reflux events. Maronian et al. (62) studied nineteen patients with SGS and was able to test fourteen with a three or four port pH probe monitor. Only two patients did not demonstrate pharyngeal reflux events. About one-half of the studied patients had idiopathic SGS and they concluded that reflux was the probable cause. The remaining group had concomitant disease states including sarcoidosis, Wegener’s granulomatosis, laryngeal trauma, and intubation. Reflux was involved in all tested patients and it was concluded that it acts as a synergistic factor that stimulates granulomatous disease to react and subsequently result in the development of stenosis.
About one-quarter of these patients had laryngeal findings and those with laryngomalacia or laryngeal stenosis had the highest number of pharyngeal reflux events. Maronian et al. (62) studied nineteen patients with SGS and was able to test fourteen with a three or four port pH probe monitor. Only two patients did not demonstrate pharyngeal reflux events. About one-half of the studied patients had idiopathic SGS and they concluded that reflux was the probable cause. The remaining group had concomitant disease states including sarcoidosis, Wegener’s granulomatosis, laryngeal trauma, and intubation. Reflux was involved in all tested patients and it was concluded that it acts as a synergistic factor that stimulates granulomatous disease to react and subsequently result in the development of stenosis.
Clinical evaluations of acid suppression and management of LTS have been overwhelmingly positive. In most studies, patients taking H2 blockers or PPIs show enhancement of surgical outcomes (63). Antireflux operation in the form of Nissen fundoplication is commonly recommended for those with LTS and confirmed EER as demonstrated from pH probe or MII-pH studies.
DIAGNOSIS
The diagnosis of LPR is based on a constellation of patient symptoms, clinical evaluation with visualization of the laryngopharynx, and specific invasive testing procedures (16). Many paradigms for diagnosis and treatment exist. The best diagnosis is based on a specific testing procedure, but many patients forego this and elect to undergo empiric therapy with acid suppression (such as a PPI, available now in the United States over the counter) as it is safe and effective. Response to treatment has been questioned as placebo effect (64
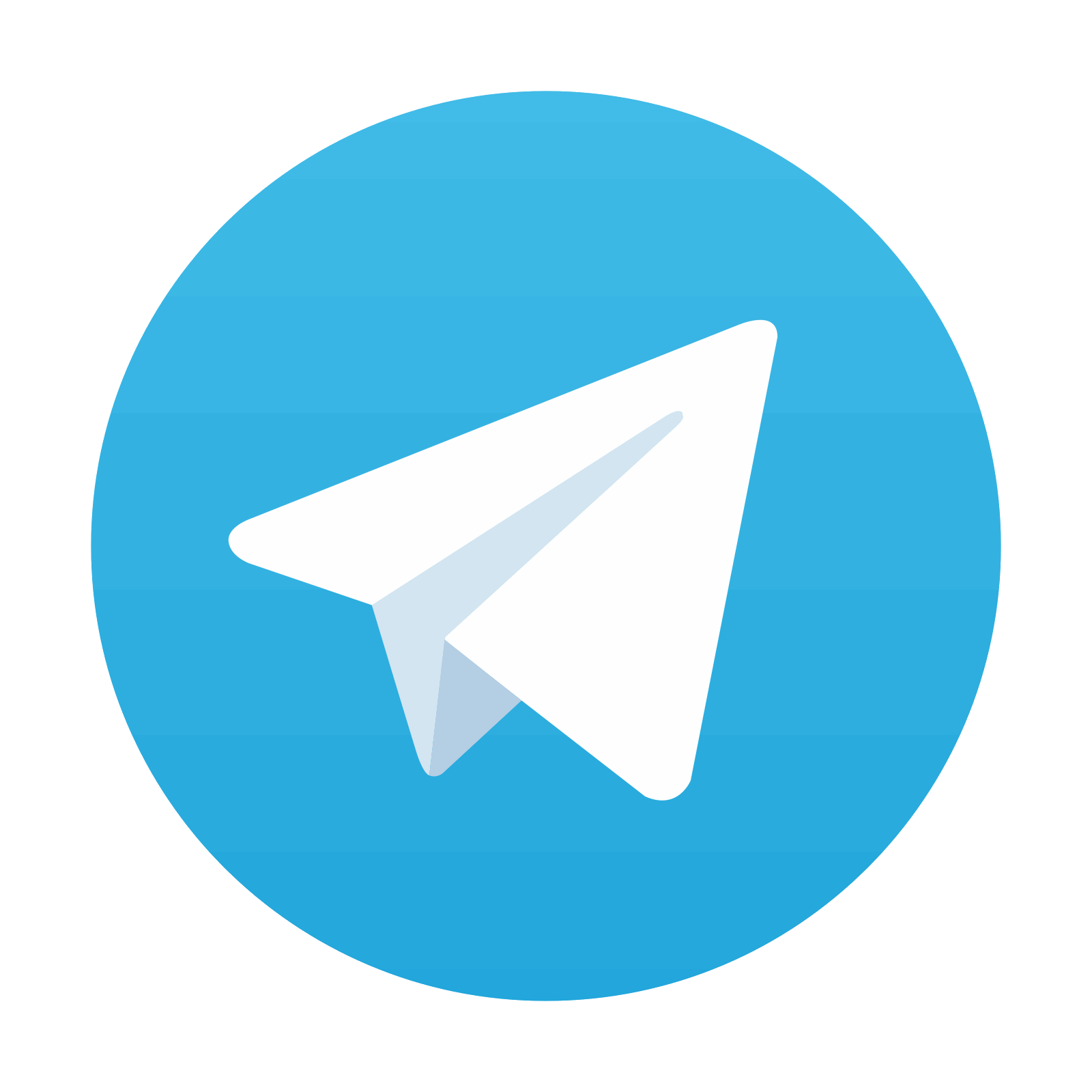
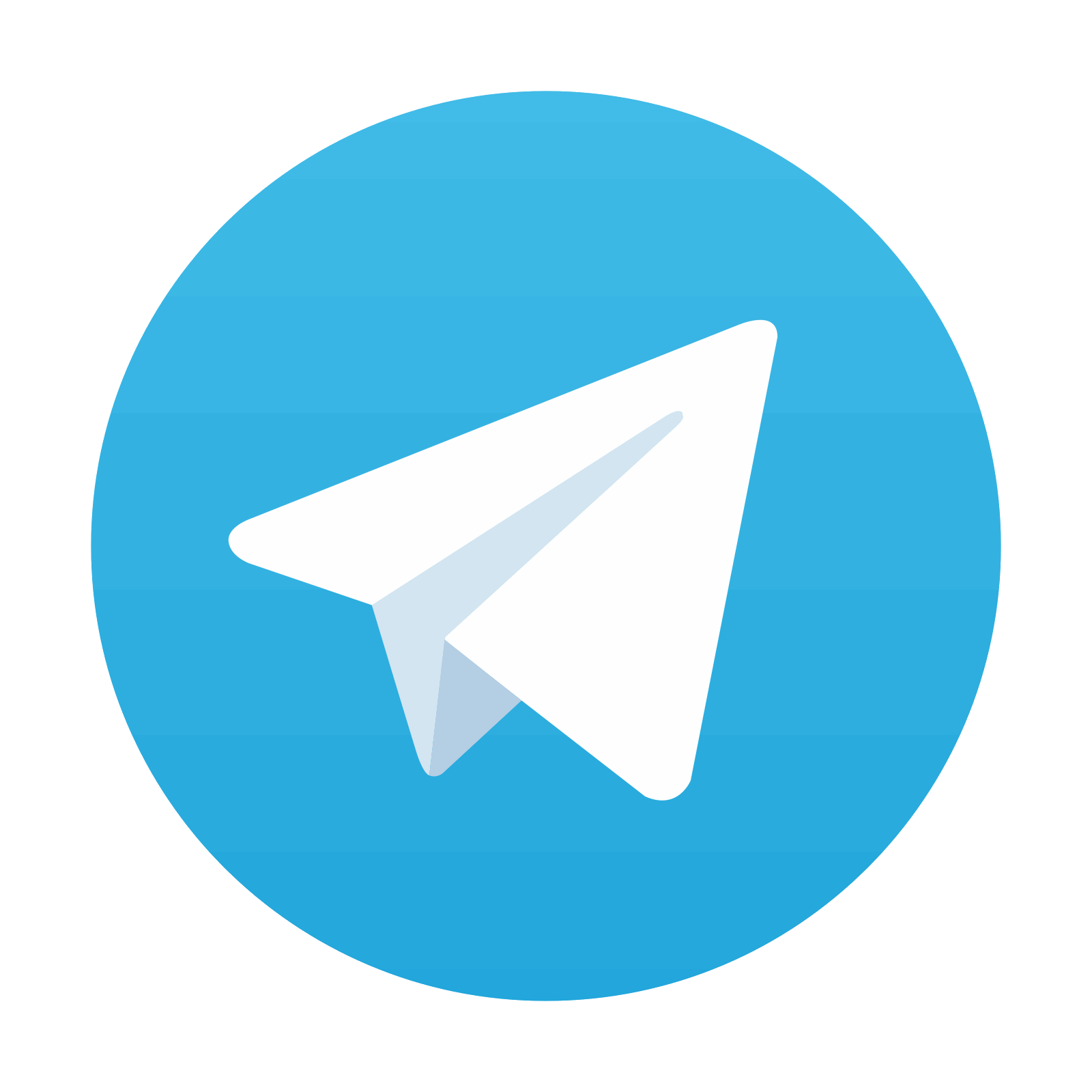
Stay updated, free articles. Join our Telegram channel

Full access? Get Clinical Tree
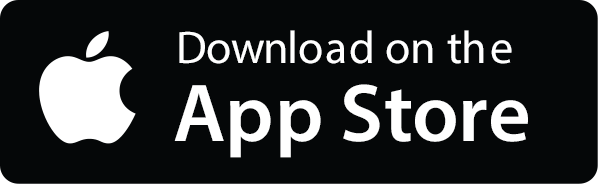
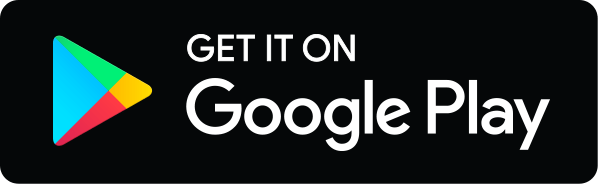
