3
Intraoperative Monitoring of Cranial Nerves During Otological Surgery: Prevention and Limitation of Inadvertent Injury
During ear and head and neck surgery, cranial nerves are at risk for inadvertent injury from mechanical, thermal, or ischemic trauma. Electrophysiological monitoring of the output of motor or sensory cranial nerves can detect signs of impending injury and in some instances preserve nerve function. In considering whether cranial nerve monitoring should be performed during a proposed operative procedure, three basic conditions for intraoperative monitoring should be met:(1) the output of the nerve can be recorded intraoperatively;(2) if signs of functional impairment appear, action can be taken to alleviate them; and(3) the monitoring procedure does not increase risk for the patient.
For many procedures in which cranial nerves are at risk, these conditions are satisfied. In these instances, monitoring is used routinely in institutions where it is available. Because electromyography (EMG) is used to monitor motor nerve function and evoked potentials (EPs) for sensory nerves, this chapter reviews the physiological basis and technological methodology involved in EMG and EP recording. This chapter also discusses the appropriate conditions for use, protocols for recording, rules for interpretation of results, training, supervision, communication within the monitoring team, and communication between the monitoring team and anesthesia and surgery teams.
Motor Nerves and Electromyography
The functional unit of muscle is called the motor unit. It consists of the group of muscle fibers connected to a single motor neuron. The electrical activity (summed action potentials) of the fibers in the motor unit is called the motor unit potential (MUP). These potentials can be recorded with surface electrodes or with needle electrodes placed in the muscle. The small voltage signals must be differentially amplified, filtered, and displayed on a computer screen and audio monitor. MUPs appear when a muscle is activated by volition, mechanical manipulation, thermal stimulation, or electrical stimulation of its nerve supply. As activation continues and more motor neurons become active, additional MUPs appear, and the frequency of their firing increases. Fig. 3–1A illustrates the shape of a single MUP taken from the muscle recording in Fig. 3–1B. The MUP was firing as a train of potentials, with a gradually increasing rate (displayed above the recording). The presence of such activity often indicates irritation of a motor nerve.
When a motor nerve is electrically stimulated, a large number of motor units in the muscle are synchronously activated. This summed electrical potential is called the compound muscle action potential (CMAP). These potentials are large (mV) and can be monitored in real time in the operating room without the use of computer averaging. The CMAP response to motor nerve stimulation is useful both to identify a structure as a motor nerve and to confirm its physical integrity. Fig. 3–1 depicts the CMAP responses of three muscles of the face elicited by electrically stimulating the trunk of the facial nerve at 5V with 0.2 msec duration pulses. The EMG traces are aligned to the onset of stimulation, at the dashed vertical lines. Each pulse elicits a single CMAP, and several responses are illustrated to show the reproducibility of the timing and waveforms. Stimulation verifies that the nerve is intact distal to the site of stimulation. Demonstrating the integrity of a motor nerve proximal to the area of surgical manipulation or a nerve not exposed in the operative field is theoretically possible by the use of blink reflex or F-waves. The blink reflex involves electrical stimulation of the supraorbital nerve, mono synaptic activation of the ipsilateral facial motor nucleus, conduction down the entire length of the facial nerve, and activation of the eye muscles. The F-wave response is recorded in facial nerve supplied muscles following electrical stimulation of the facial nerve, antidromic conduction to activate the facial nucleus motor neurons, then orthodromic conduction back to the muscles. Unfortunately, anesthesia or other technical problems have made use of these potentials unreliable in monitoring inaccessible segments of nerve.1, 2
FIGURE 3–1 (A) A single motor unit potential, firing repeatedly as a train in (B), with rate increasing over time.(C) Compound muscle action potential responses to mono-polar stimulation of the exposed facial nerve.(D) Mechanically elicited burst discharge. (E) Similar-sounding recording -artifact.
Because excitable cell membranes (either muscle or nerve) are sensitive to mechanical as well as electrical stimulation, continuous recording from muscle allows detection of nerve irritation or injury by surgical instruments. Fig. 3–1D shows an example of a burst response recorded from muscles innervated by all three branches of the facial nerve, following mechanical manipulation of the exposed nerve; Fig. 3–1E shows a similar-sounding recording artifact.
Evoked Potentials
Sensory evoked potentials are the electrical response of the nervous system to sensory stimulation. All modalities of human sensation (except perhaps taste and smell) can be used to measure the conduction time between a sensory end organ and various levels of sensory pathways in the central nervous system. Evoked potentials are of low amplitude (fractions to hundreds of μV) and require computer averaging to detect them. They cannot usually be recorded in response to a single stimulus and hence are not “real time” events. They are divided into near-field and far-field potentials depending upon their variation with electrode position and polarity. Far-field potentials are small, little affected by electrode position, and tend to be surface positive with respect to a neutral reference. Near-field potentials tend to be of greater amplitude but are influenced by electrode position. Because repeated sampling is required to discern the evoked potential signal from background noise, the rate of sampling is important, and this rate needs to be twice the fastest component of the sampled signal (Nyquist frequency). Despite these difficulties small potentials like the brainstem auditory evoked response (BAER) can be successfully recorded intraoperatively by averaging the responses to many stimuli.
■ Efficacy of Intraoperative Monitoring of Cranial Nerves
Various studies have shown the effectiveness of cranial nerve monitoring in reducing risk in otologic surgery. For instance, Harner and colleagues report that during acoustic neuroma surgeries for large tumors, facial nerve monitoring resulted in preserved facial nerve function in 67% of 48 patients, compared with only 33% of 48 matched patients not being monitored3. At our own institution, during 165 acoustic neuroma removal surgeries from 1967 to 1984, no patients had preserved hearing after surgery, and only ~50% had preserved facial nerve function. With monitoring, from 1985 to 2001, 115 of 126 patients (91%) had preserved facial nerve function, and 31 of 64 patients (48%) with normal hearing preoperatively had preserved hearing after surgery. Likewise, in a retrospective study of microvascular decompression of the fifth and seventh nerves, intraoperative monitoring of BAERs resulted in excellent outcomes: no patients had profound hearing loss. This significantly contrasts with the 7% of 152 unmonitored patients with profound hearing loss, operated upon by the same surgeon with otherwise identical techniques 4. Historically, the preservation of cranial nerve function has improved, partly due to improvements in surgical training and microsurgical techniques, but perhaps more importantly due to the increased prevalence of intraoperative monitoring.
The efficacy of diagnostic or predictive tests and systems is frequently evaluated by assessing specificity (conditional probability of true negatives) and sensitivity (conditional probability of true positives). They may be treated formally using the methodology of signal detection theory known as receiver operant characteristic (ROC) analysis. This allows for the evaluation of the effects of threshold setting on performance of the diagnostic system5. In intraoperative monitoring it is self-evident that the elimination of false-negatives is more important than limiting false-positives or detecting true-negatives. Thresholds of detection are frequently determined with these issues in mind. Unfortunately, there is little if any published material in which formal analyses have been performed. Blinded control studies are unavailable and could be considered unethical given the current standards of practice.
■ The Monitoring Team and Communication
The monitoring team consists of an experienced electrodiagnostic technician supervised by an M.D. or Ph.D. neurophysiologist6.It is important that the senior supervising neurophysiologist be on site and available, should unexpected technical or interpretive problems arise. Despite the reliability of modern equipment and the ability to transmit data in real time to remote locations, it is necessary for supervisory personnel to recheck initial setup and recordings because it is relatively easy to reverse connections, experience a broken wire, or connect a stimulator to an inactive output. It is most important to establish the pattern of communication desired. The technician can be in the operating room or adjacent to it. The auditory monitor can be available in the room for the surgeon to hear it or restricted so only the technician hears it. Communication between the monitoring staff and the surgeon needs to be clear-cut and timely. The effectiveness of monitoring is increased if the surgeon maintains frequent communication with the monitoring staff. Communication with the anesthesia team is important in assuring a stable baseline before operative manipulation is undertaken and to make sure neuromuscular blockade (if it is employed) is not excessive.
■ Which Operative Procedures Should Be Monitored?
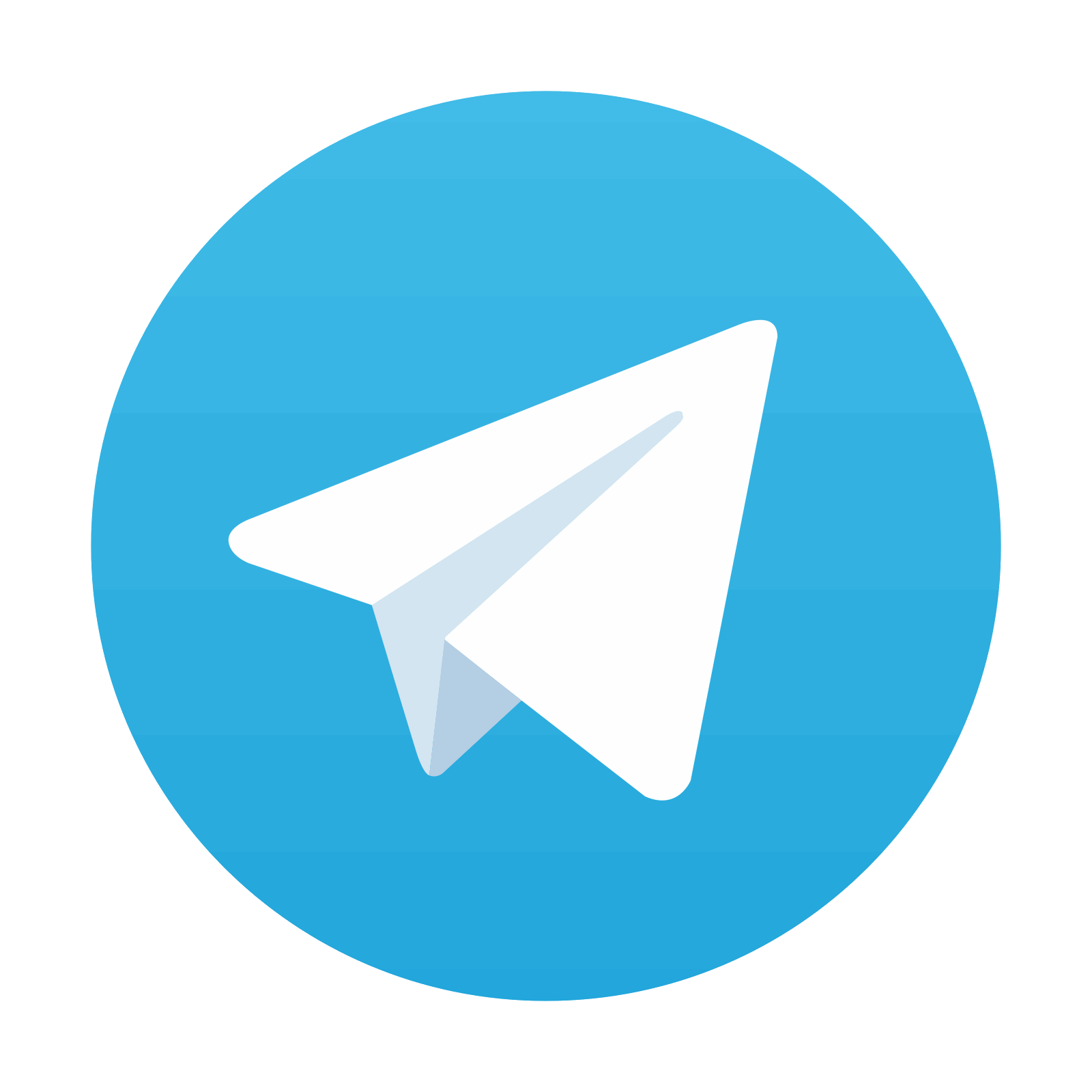
Stay updated, free articles. Join our Telegram channel

Full access? Get Clinical Tree
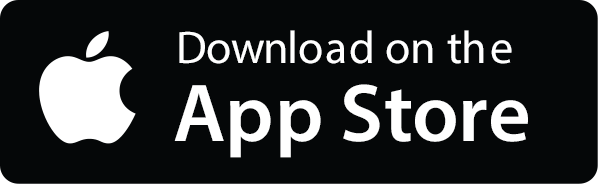
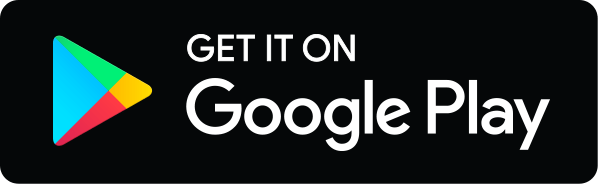