Indocyanine Green Angiography
Eric D. Weichel
Carl D. Regillo
Joseph I. Maguire
Because of the limitations of fluorescein angiography (FA) in imaging the choroidal circulation and associated pathology, investigators have searched for alternative dyes to improve choroidal angiography. The most promising has been indocyanine green (ICG) dye. Although ICG angiography (ICGA) has been performed for more than 30 years, it has not been until relatively recent, with technologic advances in high-resolution digital imaging systems or scanning laser ophthalmoscopes (SLO) together with infrared-sensitive video cameras, that the potential clinical advantages of ICG dye over sodium fluorescein were finally demonstrated.1,2,3,4
Investigations with ICGA and ocular applications date back to 1970 when Kogure et al.5 performed intra-arterial choroidal absorption angiography in monkeys. The quality of the images, using false-color infrared film along with the route of administration, limited this technique’s usefulness. Reports describing intravenous injection of the dye and the use of black and white infrared film for absorption angiography followed.6 These changes allowed easier, more consistent angiograms. In 1973, Flower and Hochheimer7 described a method of ICG fluorescence angiography, providing improved resolution of the choroidal vasculature compared with absorption angiography.
Although a variety of fundus conditions were subsequently studied using ICG fluorescence angiography, clinically useful choroidal images remained elusive due to the limited ability of available systems to enhance low ICG dye fluorescence.8 In 1986 Hayashi et al.9 reported the use of an infrared-sensitive video camera to perform ICGA. Compared to prior film studies, videoangiography, along with improvements in illumination and excitation-barrier filter separation, advanced image quality.
Numerous reports followed indicating a potentially significant advantage of ICGA over FA in imaging choroidal pathologic processes. In 1989, Destro and Puliafito1 demonstrated improved visualization of choroidal neovascularization (CNV) using ICGA compared with FA in selected cases. They also introduced the concept of late imaging, whereby images obtained after clearing of ICG dye from the choroidal circulation show persistent hyperfluorescent pathology against a dark background.
The use of higher-resolution digital monitors (1024-line) coupled with infrared-sensitive video cameras, described by Guyer et al.3 and then Yannuzzi et al.4 in 1992, provided even better images. ICG enhanced visualization of choroidal lesions, such as CNV, compared with FA was consistently obtained in several large clinical series.4,10,11 Similar ICGA diagnostic yields in the setting of exudative macular degeneration were also obtained by investigators using SLO systems.12,13
CHEMICAL AND PHYSICAL PROPERTIES OF INDOCYANINE GREEN
Indocyanine green (C43H47N2O6S2Na) is a water-soluble tricarbocyanine dye with a molecular weight of 775 daltons.14,15,16 Initially used in cardiac and liver function tests, ICG’s clinical utility in fundus angiography results from its spectral properties in the near-infrared range.3,8 Compared with sodium fluorescein, whose peak absorption and emission is in the visible spectrum, ICG has a peak absorption in serum between 790 and 805 nm and a peak emission at 835 nm.14,17,18 These spectral properties result in excellent penetration of the retinal pigment epithelium, macular xanthophyll, other ocular pigment, and even blood, allowing superior viewing of the choroidal vasculature. Since longer wavelengths undergo less scatter than shorter wavelengths, visualization through media opacities is improved as well.
ICG is more highly bound to blood proteins than sodium fluorescein. Nearly 98% of circulating ICG is bound to various serum proteins, such as albumin and a-lipoprotein.15,19,20 (Fluorescein dye is only 60% to 80% protein bound.) This high degree of binding and possible preferential binding to high–molecular-weight lipoproteins may explain the dye’s apparent poor penetration of capillary fenestrations in the choriocapillaris. This tendency of ICG to remain intravascular facilitates visualization of the choroidal vasculature.15,19,20
After intravenous injection, ICG is rapidly eliminated by the liver and exhibits minimal uptake in the peripheral tissues. ICG is not chemically altered in the liver and has been recovered in the bile unchanged.15,21 There is no apparent reabsorption in the bowel. The dye is not detected in the cerebrospinal fluid and does not appear to cross into the placental circulation.22
TOXICITY OF INDOCYANINE GREEN
ICGA is a relatively safe procedure with few reported adverse reactions over 20 years of clinical use in different areas of medical practice. The early nonophthalmic literature reported rare adverse effects such as urticaria, chills, hypotension, and dyspnea with a relatively large portion of the cases in patients with either iodine allergies or uremia.23 Two deaths were also reported in this review, with both cases in the setting of cardiac catheterization; a causal relationship to the dye itself was unclear.
In the ophthalmic literature, a comprehensive analysis revealed seven reactions in a consecutive series of 1923 ICGA procedures performed in 1226 patients.24 These reactions included nausea and vomiting in two cases, urticaria in two cases, vasovagal reactions in two cases, and acute hypotension in one case. This represents a 0.3% adverse reaction rate for ICGA. A review of the literature from this report identified 18 severe reactions and 3 deaths. With approximately 1 million doses sold at that point, a 1 in 333,333 incidence of death was estimated. There have been two additional cases of anaphylactic shock following ICGA.25,26 In comparison, FA has an estimated adverse reaction rate of between 2.7% and 11.7% along with a death rate of approximately 1 in 222,000 angiograms27; therefore, ICGA appears to be a safer test.
Given the chemical characteristics and pharmacodynamics along with the profile of patients who experienced some of the more serious adverse reactions associated with the use of ICG dye, ICGA is contraindicated in patients with iodine or shellfish allergies, liver disease, and end-stage renal disease. It should also be avoided in pregnancy at this time, given the lack of human toxicity data in this area.28 Extravasation of the dye causes local tissue irritation and can produce tenderness, but no significant permanent tissue damage has been reported.
FUNDUS CAMERA-BASED INDOCYANINE GREEN ANGIOGRAPHY
The fundus cameras used for ICGA are modified to include antireflective coatings and filters for maximal transmission of infrared wavelengths and to allow widening of the camera aperture to increase the amount of infrared light entering the camera. The images obtained with these modified cameras also allow color fundus or fluorescein angiogram images.
Digital imaging cameras are similar to 35-mm film cameras. Instead of a shutter and exposure of a silver-based film, an electromechanical shutter opens, sending light to a computer coupling device (CCD) containing light-sensitive elements called pixels. The camera then converts the analog signal to a digital signal and stores the image in a hard drive for immediate viewing. The CCD in an ICG camera is designed to sense infrared light wavelengths. The images are captured at one frame per second and displayed on a high-resolution video monitor. The stored images are then printed as hard copies or saved as digital files. The combination of an infrared light-sensitive camera with a digital imaging system allows high-resolution (1024 line) images needed for useful clinical ICG angiography. Computer software also allows clinicians to digitally enhance the ICGA images. These contrast-enhanced angiograms allow for higher diagnostic yield when compared to nonenhanced images.29
SCANNING LASER OPHTHALMOSCOPES–BASED INDOCYANINE GREEN ANGIOGRAPHY
The scanning laser ophthalmoscope uses a small laser beam to scan across the retina. The light reflected from this illuminated spot is detected and electronically coded for image generation. The confocal SLO places a small pinhole aperture in the plane conjugate to the focus plane, thereby removing any scattered and reflected light from outside of the focal plane while improving contrast sensitivity and image contrast.31 The advantages of the confocal SLO over fundus camera images include, but are not limited to, superior resolution at a focal plane of a particular depth and imaging through small pupils and media opacity.31
Two confocal scanning high-speed infrared laser ophthalmoscopes are being used in clinical practice (Heidelberg Retina Angiograph (HRA), Heidelberg Engineering GmbH, Heidelberg, Germany, and Rodenstock SLO, Canon, Tokyo, Japan). The high-speed ICGA (HS-ICGA) HRA allows frame capture up to 20 frames per second. Due to the confocal system and laser illumination, the HRA detects faint hyperfluorescence and digitizes with high pixel resolution. These 30-degree field-of-view images are usually obtained in the transit phase of the ICGA after a 0.3 mL bolus of ICG injected rapidly after a 5-mL saline flush. This imaging system allows high resolution and evaluation of vessels with a diameter of 50 microns.
Gelisken et al.32 examined 100 consecutive occult CNV patients with SLO versus high-resolution digital fundus ICGA. They found that the SLO was superior in detecting well-defined vessel structure, whereas the digital fundus camera best detected focal hyperfluorescent spots and late-appearing plaques.
NORMAL VIDEOANGIOGRAM AND ADMINISTRATION TECHNIQUE
From a clinical standpoint, ICGA using a digital video system can be grossly divided into early, middle, and late phases. Although SLO-based ICGA can be similarly described, investigators using this technique have concentrated more on the earlier images.12,13 Therefore, the features of a standard ICG videoangiogram based on recent studies using the standard 1024-line digital systems will be described.3,4,11
The “early” phase (0–3 minutes after injection) encompasses the period from the first appearance of ICG dye in the choroidal arterial circulation to the point of maximal ICG choroidal hyperfluorescence, usually occurring within the first minute after the injection of dye (Fig. 1A). During this phase, both medium and large choroidal arteries and veins are well visualized beneath the hyperfluorescent retinal vasculature. Individual choriocapillaris vessels cannot be distinguished. The areas surrounding middle and large choroidal vessels appear relatively hypofluorescent. This “pseudohypofluorescence” is, at least in part, a result of a smaller volume of blood in the choriocapillaris compared with the larger vessels, with the illumination intensity adjusted for the strongest portion of fluorescence.
In the “middle” phase of the angiogram (5–15 minutes after injection), the choroidal veins become less distinct as a nearly homogeneous, diffuse choroidal fluorescence emerges (see Fig. 1B). The fluorescence from the retinal vessels also begins to attenuate. Lesions that demonstrate abnormal hyperfluorescence on ICGA typically begin to stand out in contrast to the fading surrounding normal background fluorescence by this point in the study.
In the “late” phases (beyond 18–22 minutes), all details of normal retinal and choroidal vessels are lost as the hyperfluorescence fades even further (see Fig. 1C). The choroidal vessels now stand out in relief as hypofluorescent channels and retinal vessels are no longer visible, and the optic nerve head is dark. There is maximal contrast with any abnormal hyperfluorescent lesions. The edges of such lesions may exhibit some “fuzziness” at this stage, apparently from limited dye leakage.
For the standard ICGA, 25 mg (12.5 to 50 mg) of ICG dye in the manufacturer’s diluent is administered intravenously in a bolus fashion, similar to intravenous fluorescein angiogram protocols. Images are typically obtained at several second intervals until the retinal and choroidal circulations are maximally hyperfluorescent and then at approximately 30- to 60-second intervals for the first few minutes of the study to capture images through the early phase of the angiogram. Subsequent images are typically taken between 8 and 12 minutes for the middle phase and then between 18 and 25 minutes for the late phase. Most importantly, abnormal ICG hyperfluorescence is sufficiently identifiable by 25 minutes, but, occasionally, images obtained 30 to 40 minutes into the study are helpful. To image retinal and choroidal vessels in the late phase of the angiogram, a technique of reinjecting a small amount of ICG dye at 30 to 40 minutes into the study has been described.33
The available software packages of most digital videoangiogram systems have image-enhancing and image-tracing capabilities. Limited improvement of image contrast can be obtained with various enhancement techniques. The ability to align and superimpose images and tracings (“warp tracing”) can be helpful in determining the location or size of a particular angiographic finding, with respect to other anatomic structures (Fig. 2).4,11
APPLICATIONS
In general, the most promising clinical application of ICGA is as an adjunct to FA in the diagnosis and management of exudative age-related macular degeneration (AMD).2,3,4,11,12,13 ICGA may also be of value in evaluating other choroidal-based pathologic processes, such as choroidal tumors, central serous chorioretinopathy, and choroidal inflammatory or degenerative diseases.9 ICGA has been used in various ways to study choroidal vascular flow, and the technique, in general, may be helpful in elucidating the pathophysiology of diseases involving the choroidal vasculature.8,37,39,40,41,42,43
AGE-RELATED MACULAR DEGENERATION
PATHOPHYSIOLOGY OF CHOROIDAL NEOVASCULARIZATION
In AMD, it has long been postulated that focal choroidal ischemia may play a role in the development of CNV. Macular choroidal vascular “watershed” zones have been known to exist, but their causal relationship to CNV has never been determined. On ICG angiography, these presumed watershed zones appear as areas of relative, abnormal hypofluorescence in the early phases of the angiographic study.44 A published ICGA analysis by Ross et al.45 revealed a much higher incidence of the presumed watershed zones in the macula of eyes with AMD compared with age-matched control eyes (55% vs. 15%, respectively). Furthermore, Goldberg et al.46 found that 92% of eyes that developed choroidal neovascular membranes had watershed zones. These data support the notion that watershed zones play a role in CNV development and that ICG angiography may be useful once again in identifying eyes at the highest risk for the exudative transformation. Other studies have shown that ICGA can distinguish the different types of drusen and, therefore, may be useful to evaluate the risk of progression of AMD.47
CLASSIC CHOROIDAL NEOVASCULARIZATION
Classic CNV as determined by FA shows a similar appearance on ICGA with well-defined hyperfluorescence throughout the transit phase and leakage obscuring the borders of the lesion in the late frames. However, the leakage in the late frames tends to be less pronounced with ICGA. Overall, ICGA offers no advantage over fluorescein angiography in this setting.
OCCULT CHOROIDAL NEOVASCULARIZATION
Occult CNV assumes a variety of FA patterns.48 There can be obscuration of the neovascularization from fluorescein blockage by overlying fluid or pigment or from rapid leakage of the dye in the area of interest. Sometimes there is slow, irregular fluorescein leakage with poorly delineated borders. With occult CNV, there may be varying degrees of uncertainty as to the precise location and extent of the choroidal neovascular membrane using FA alone.
Both fluorescein and ICG dyes appear to be retained by CNV and will, therefore, exhibit hyperfluorescence relative to surrounding tissue.1 ICGA theoretically provides enhanced visualization of CNV in this setting because the ICG infrared fluorescence better penetrates pigment and fluid than the visible light fluorescence of sodium fluorescein, and the highly protein-bound ICG dye leaks less from abnormal vessels compared with fluorescein dye as described earlier. Several patterns of ICG hyperfluorescence for occult CNV have been observed.4,11,49,50 These include early-appearing small hyperfluorescent spots (hot spots), plaque-like hyperfluorescence, collections of abnormal vessels, and late-appearing hyperfluorescence with indistinct edges (Figs. 3, 4, and 5). A combination of these patterns can be seen. Although thin blood is easily penetrated by the infrared fluorescence (Fig. 6), thick blood will still obscure the underlying choroidal pattern to some degree. Reichel et al.51 demonstrated that ICGA was superior to fluorescein angiography in determining the extent of CNV secondary to age-related macular degeneration obscured by hemorrhage.
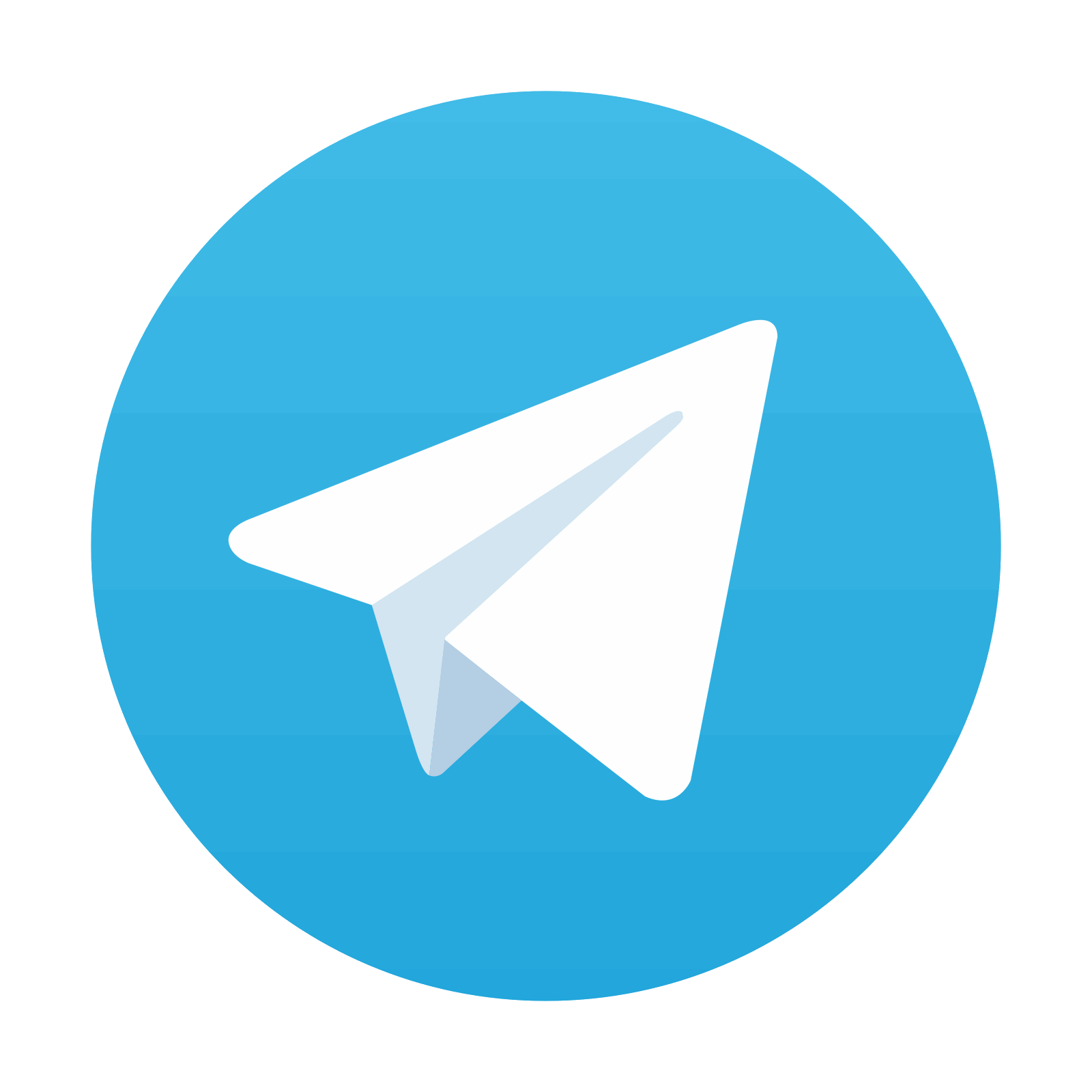
Stay updated, free articles. Join our Telegram channel

Full access? Get Clinical Tree
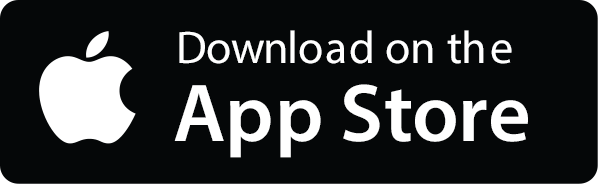
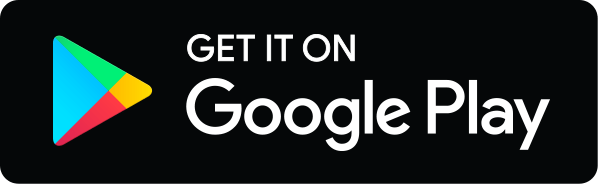