Introduction
Immunology is the study of host defence mechanisms. Even the simplest organisms have the ability to mount a variety of specific and non-specific responses to invasion or attack by foreign organisms. Where the host and pathogen meet and interact is where the immune response is initiated and the nature of both players determines the outcome. Thus humans can respond to pathogens in many different ways, depending on their genetic make-up, the type of foreign organism (e.g. virus, bacterium, fungus, etc.) and prevailing conditions or the setting in which the host is under threat.
The immune response is even determined by the nature of the tissue invaded. For instance, the eye (and the brain) respond when under foreign attack, but under certain circumstances the predicted response does not occur but is rather modulated by the tissue; this is called ‘immune privilege’ and is related to the special microenvironment and immunoregulatory mechanisms operating in these tissues.
Most of this chapter outlines the basic principles of immune responses, with reference to the eye as appropriate. At a very simple level, however, it must be remembered that the organism has very effective means to prevent host and pathogen meeting each other in the first place: namely, barriers such as the skin and mucous membranes with their surface coverings (including that most important layer of mucus present on mucosal surface tissues).
Overview of the immune system
Immunity is defined as the ability of the host to protect itself against a foreign organism or pathogen. To do this it requires an immune system comprising the cells and molecules used in the host’s defence. For unicellular hosts this may simply mean certain molecules on the cell surface that enable it to recognize foreign organisms. However, for higher-order hosts the immune system is a highly organized network of tissues, cells and molecules.
Hosts defend themselves by mounting an immune response involving the activation and recruitment of the cells and molecules of the immune system. Currently, the immune system is considered in terms of its specificity: the innate (natural or native) immune system and the acquired (adaptive) immune system . In its first line of defence against attack, the host uses the innate immune system because this is rapidly mobilized and is not dependent on previous exposure to the foreign invader, i.e. does not involve ‘memory’. This form of response is considered relatively non-specific in that the same sort of response occurs to most foreign organisms and even to injury itself. It involves activation of cells (such as macrophages, see p. 377 ) and molecules (such as complement, see p. 405 ) which are present in the tissues and fluids of all higher organisms.
Innate immunity, however, has some degree of specificity in that innate immune cells such as macrophages ‘sense’ pathogens through receptors that recognize generic molecular ‘patterns’ which characterize different types of pathogens (pathogen-associated molecular patterns or PAMPs). Thus there are receptors for broad groups of molecules present in different organisms such as viral DNA, fungal carbohydrates and bacterial endotoxins (see Ch. 8 , p. 464 ). However, as stated above immune responses can be initiated not only by pathogens, but simply by injury, or by molecules such as plant pollens (causing allergies), and especially by ‘alloantigens’ (those molecules that differentiate individuals within a species and cause rejection of donor-unrelated transplants). Accordingly, Matzinger (2012) developed Janeway’s PAMP hypothesis to suggest that innate immunity has evolved to allow the host to sense ‘danger’ or damage to tissues and includes anything that threatens host or tissue viability, namely pathogens of all varieties, sterile trauma such as chemical or thermal injuries, non-self proteins such as those present in allografts, and even ‘self’ proteins if these have become ‘abnormal’ (as in the case of cancer cell proteins, prion proteins, and even self proteins (auto-antigens).
There has been a recent explosion of knowledge concerning the innate immune system (see below). After the initial discovery of the phagocytic leucocyte (macrophage) by the Russian zoologist Metchnikoff at the beginning of the 20th century, much of immunology was devoted to immunochemistry of antigens and antibodies and then, in the latter half of the 20th century, to developments in cellular immunology, involving cells of the adaptive immune system such as T cells and B cells. Specificity for pathogen recognition by innate immune cells was considered non-existent until Janeway and others identified receptors in the fruit fly Drosophila which were important not only for wing patterning in development but also for recognition of pathogens, such as fungi. His earlier proposal that there were classes of receptors recognizing broad groups of pathogens such as viruses, fungi, bacteria, including mycobacteria, and parasites was shown to be true by the discovery of Toll receptors. Other classes of pathogen-associated molecular patterns were later discovered. However, as Matzinger pointed out, pathogens were not the only agents which activated the innate immune system. Allergens also initiate immune responses, as do allografts, where infectious pathogens were considered not to play a part. Her concept of molecules being either ‘dangerous’ or ‘harmless’ has changed the thinking of immunologists in many ways. In addition, she has developed her concepts to consider that different tissues respond to the same insult immunologically in different ways: for instance, the skin and the liver might produce a modulated immune response to the same antigen. In one sense this is a rewriting of the notion of immune privilege, which has been long accepted as a modified immune response taking place in the eye (see p. 457 ).
Accordingly the molecular patterns associated with danger signals and tissue damage are known as danger- or damage-associated molecular patterns (DAMPs) and include PAMPs. Thus bacterial and viral products such single- or double-stranded RNA, endotoxin, complement fragments, reactive oxygen species, heat shock proteins, high mobility group box (HMGB) proteins, and many other molecules (sometimes called ‘alarmins’) can act as DAMPs.
The host-defence immune system thus includes:
- •
physicochemical barriers such as the skin, eyelids, tears (see Ch. 4 )
- •
molecules normally present in body fluids such as blood, tears and aqueous humour (e.g. complement, lysozyme, antiproteases). Antibacterial defensins are also on this list of ocular surface proteins
- •
phagocytic and cytotoxic cells such as polymorphonuclear leucocytes, macrophages, eosinophilic granulocytes, natural killer (NK) cells
- •
molecules released by cells responding to attack and acting on other cells (cytokines), such as interleukins and tumour necrosis factor-α (TNF-α) (see below) and complement.
The acquired immune system includes:
- •
specific immune systems associated with barrier surfaces (the skin immune system and the mucosa-associated immune system, MALT) where memory T cells reside and have ‘first call’ on invading pathogens
- •
a constant source of naive T and B lymphocytes with potential to modulate their receptors to specifically recognize foreign organisms and molecules (antigens)
- •
molecules that specifically counteract foreign antigens (antibodies); these proteins are known as immunoglobulins and there are five types (see p. 396 )
- •
non-specific molecules (e.g. cytokines) released by antigen-specific cells (e.g. lymphocytes) which will recruit further myeloid cells to sustain the response as needed.
Immunity after infection is normally termed active immunity in that the host has responded actively to the stimulus. However, immunity may be transferred passively by antibodies or cells. Vaccination procedures that involve the administration of antibodies are termed passive immunization , while those that involve inducing a response to the antigen or even the attenuated live organism are termed active immunization (see Ch. 8 ).
The development of acquired immunity involves a number of discrete phases, including:
- •
an afferent phase in which the foreign antigen is transported from the site of entry and presented by specialized antigen-presenting cells (APCs) to the lymphocytes in the lymphoid tissue (see below)
- •
a phase of T-cell activation in which T cells are transformed from a resting to an active state
- •
an effector stage in which T cells induce other cells, such as B cells and macrophages, to remove the antigen. If the antigen remains intracellular, as with virus-infected cells, the T cells themselves attack the infected cell (cytotoxic T cells).
Throughout the development of the acquired immune response there are several checkpoints that prevent a runaway overwhelming inflammatory reaction. Most of these regulatory mechanisms are in place to prevent adaptive immune responses to self-antigens but they also participate in downregulatory responses to foreign antigens and help to restore homeostasis.
Innate immunity with its early warning, rapid-response system provides a reliable means of protecting the host against most extracellular organisms (pathogens) and is a property of every living being. It might be asked, therefore, why the acquired immune system has evolved? In part this is due to the remarkable ability of organisms to evade the immune system. Many pathogens reside and hide within the host cell, as in the case of protozoa such as Toxoplasma , obligate parasites such as Chlamydia or, more frequently, viruses. Viruses use the host cell machinery for survival and replication by incorporating their genome, or at least part of it, into the DNA of the host cell. This may lead to viral latency or persistence, but may also allow the immune system to recognize the infected cells by the expression of the foreign antigen on the surface of the cell in addition to the self molecules (also termed antigens because they can induce an immune response). It is the recognition of the foreign antigen in the context of self-antigen (i.e. together with self-antigens, the peptide–major histocompatibility complex (MHC); see p. 424 ) that led to the evolution of the acquired immune system. However, many pathogens continue to evade the immune system by subverting the function of the innate immune cells in which they reside or by becoming ‘latent’ as in the case of herpes simplex-infected neurones.
The innate immune system is the initial primary responder to pathogens or other challenges. The acquired immune system has developed a considerable degree of sophistication, based on memory of previous pathogen encounters, with an increasingly recognized range of different cell types: T and B lymphocytes; T and B cell subsets (e.g. T helper (Th), T cytotoxic (Tc), T and B regulatory (Treg and Breg) cells; and even subsets of these (e.g. Th0, Th1, Th2, Th17) (see below), each designed for specific cellular functions. In addition, T and B cells have evolved in fundamentally different directions – T cells to deal with surface-bound antigen (usually cell-associated) and B cells to deal with soluble (extracellular) antigen. The sophistication and specificity of the acquired immune system involving T cells has thus been harnessed to assist the innate immune system in dealing more efficiently with extracellular organisms, for instance via B cells.
Certain basic concepts about immune mechanisms can therefore be derived from the above considerations:
- •
extracellular foreign antigen is normally cleared by the innate immune system, with some assistance from B-cell activity
- •
intracellular foreign antigen may evade the immune system and remain latent during the lifetime of the host unless (1) it kills the cell, is released and generates a second innate immune response to remove the pathogen; (2) it is expressed on the surface of the cell in the context of self-antigen and is recognized by the acquired immune system, which kills the infected cells and clears the pathogen (applies to many viruses, e.g. influenza); (3) the host immune system is compromised, in which case the intracellular pathogen proliferates freely, killing many cells and ultimately the host
- •
all cellular defence mechanisms involve interactions of cell surface molecules (receptors) with complementary molecules (ligands).
The total number of separate genomes from microorganisms colonizing a specific site (known as an environmental ‘niche’) constitutes a ‘microbiome’ for that site. There are 10 times more microbial cells than human cells in the body and although it does not weigh much more that 500 grams, its importance to normal physiological processes is such that some regard it as a separate and essential ‘organ’. Most of the organisms that constitute the microbiome cannot be cultured and have been identified by genetic mapping techniques. The most important role for the microbiome may be in immunoregulation and prevention of disease including autoimmune disease, diabetes, atherosclerosis, cancer and other diseases associated with chronic inflammation and obesity. It may also have a role in psychiatric disease through its effects on neurotransmitter production.
High-throughput sequencing has revealed substantial intra-individual microbiome variation at different anatomical sites, and inter-individual variation at the same anatomical sites (see figure). However, the microbiome at specific anatomical sites can remain remarkably stable over time in each individual. The figure indicates the relative proportion of sequences determined at the taxonomic phylum level at eight anatomical sites. Certain features, such as the presence (+) or absence (−) of Helicobacter pylori , can lead to permanent and marked perturbations in community composition. (adapted from Costello et al., 2012).

This lack of immune responsiveness to foreign antigens can be described as ‘tolerance’ of foreign organisms and indeed the immunological obverse of ‘immunity’ is ‘tolerance’. Tolerance as a defining characteristic can be applied to any antigen, but is especially applied to self-antigens, since clearly we do not normally react to our own tissues. It has been realized that tolerance to self-antigens, and indeed to any antigen, is an active process in which certain T cells (T regulatory cells [Tregs]) are critical for its normal function. Many effector immune responses to infectious foreign antigens are controlled by the induction of a specific Tregs to that organism. Tregs are part of our normal circulating T-cell population and when Treg function goes awry, autoimmune diseases can ensue; diseases such as various forms of dry eye disease (Sjögren syndrome, see Ch. 9 ), rheumatoid arthritis and some forms of uveitis are considered autoimmune diseases. Indeed there is a growing recognition that both the innate and the acquired immune response co-evolved with microbes, each exerting counter-regulation on the other.
To summarize, acquired immune responses have certain features that are inherent to them:
- •
specificity – based on certain features/determinants (known as epitopes) of the antigenic structure
- •
non-responsiveness to many antigens (prototypically, self-antigens), also known as tolerance, the loss of which underpins autoimmune disease
- •
diversity – around 10 9 individual epitopes can be distinguished – this is known as the lymphocyte ‘repertoire’ and represents the range of potential foreign antigens to which immune responses can be generated
- •
memory – the secondary immune response, specific to that antigen and present in both B and T cells
- •
specialization – the immune response is customized to different microbes
- •
ability to downregulate – the immune response is strictly regulated/limited in magnitude and time through specific and non-specific mechanisms.
Cells and molecules of the immune system
The cells of the immune system are mostly specialized to participate in processes belonging either to the innate or the acquired immune systems ( Box 7-2 ). Some cells are central to both and the distinction between innate and adaptive immune cells is becoming blurred with the discovery of cells such as innate lymphoid cells (see p. 382 and Video 7-1).
The myeloid system and innate immunity
The cells directly involved in bacterial killing and removal of damaged host tissue at the site of entry of the foreign organism are the cells of the myeloid system. Some of these cells are recruited to the site of injury during acute inflammation. Their defining feature is the presence of cytoplasmic organelles (lysosomes) containing a battery of hydrolytic enzymes for both intracellular and extracellular killing.
Neutrophilic granulocytes
Neutrophilic granulocytes (polymorphonuclear leucocytes) are the most common white cell in the circulation and are attracted to sites of inflammation by chemotaxis (see p. 387 ). These are fully differentiated cells with no capacity for proliferation. They are primary scavengers, causing much of their effect via release of free radicals and proteases from their numerous cytoplasmic granules and lysosomes. These include defensins, lysozyme, lactoferrin and oxidative enzymes (e.g. NADPH-dependent oxidases, myeloperoxidases and catalase), which are also present in ocular fluids such as tears. The half-life of neutrophils is 1–2 days.
This limited ‘clearing-the-bugs’ role has been revised recently with many new functions being ascribed to neutrophils. PMNs have been shown to use a remarkable mechanism for ‘trapping’ bacteria and other ‘dangerous’ material by extruding NETS (neutrophil extracellular traps), composed predominantly of nuclear DNA material, during the process of cell death. NETS are very ‘sticky’ and powerfully prevent bacterial dissemination. However, they can also have deleterious effects by trapping metastatic cells and protecting them from immune surveillance (see eFig. 7-2 ).
Neutrophils may also participate in the adaptive immune response through release of cytokines and extracellular vesicles which mediate intercellular communication.
Myeloid mononuclear cells
Monocytes, macrophages and dendritic cells are cells of the mononuclear phagocyte system. Like neutrophils, these cells are derived from haematopoietic bone marrow stem cells and differentiate into a variety of tissue macrophages (histiocytes) with specific functions ( Box 7-3 ).
Macrophages have many functions as part of the innate immune system: they phagocytose dead and damaged cells and organisms in inflammatory exudates; they release cytokines of various sorts, which may activate other cells such as lymphocytes and eosinophils; and they are involved in the acquired immune system as antigen-presenting cells (APCs), as effector cells involved in the process of cell lysis, and by removing antibody-coated (opsonized) cells and particulate material.
Macrophages are, however, not simple cells. There are several varieties of macrophages, some with ‘housekeeping’ roles as resident cells whose job is to remove dead and dying cells during the normal tissue cell turnover. Other macrophages are recruited to sites of inflammation, differentiating from circulating blood monocytes, and are highly active in tissue destruction. These cells are sometimes referred to as classically activated ‘M1’ macrophages with a strongly pro-inflammatory function, while resident and other macrophages are considered to be alternatively activated ‘M2’ macrophages and function more in healing processes including fibrosis and new vessel formation ( eFig. 7-1 ).
The functional subtyping of macrophages is of considerable importance to the understanding of disease processes including many ocular diseases such as uveitis, and macular degeneration. The initial classification into M1 classically activated macrophages and M2 alternatively activated macrophages has developed further into a realization that there is greater plasticity in macrophage function than was once thought ( eFig. 7-1 ). Classically activated macrophages remain major players in innate immune defence with the job of removing dangerous foreign and endogenous antigens, clearing necrotic and damaged tissue and setting the scene for repair of tissue. This is conducted by alternatively activated macrophages involved in such processes as generating new blood vessels to restore blood supply to the tissues and produce factors which rein in the excesses of the inflammatory response. Meanwhile a third type of macrophage differentiates to mediate other aspects of wound healing and repair, i.e. to promote maturation of blood vessels and fibrosis, while a fourth hybrid type of macrophage has features of a regulatory (anti-inflammatory) macrophage, producing the anti-inflammatory cytokine IL-10 if it encounters bacterial factors such as lipopolysaccharide.

Macrophages, like B cells, usually capture antigen (e.g. ‘opsonized’ bacteria) for processing and presentation to T cells via surface receptors such as the complement receptor and the Fc receptor. The Fc receptor on macrophages binds the Fc portion of antibody–antigen complexes while B cells express antibody (immunoglobulin) on their surface and use the Fab portion of the antibody to capture antigen. In both cases, preformed antibody to that antigen is needed and so the immune system must have already been exposed to antigen. It is clear therefore that macrophages and B cells are only effective as APCs in the later stages of an ongoing immune response.
In contrast, the most potent APC in the immune system is the dendritic cell (DC). The DC is the key cell in linking the adaptive and innate immune systems. While the function of most myeloid cells is to engage in innate immune defence functions by marshalling forces against infectious and non-infectious danger signals, the DC takes this one step further: after phagocytosing, killing and digesting an invasive microorganism the DC, unlike the macrophage or the B cell, or even ‘non-professional’ APCs, can present the processed antigen to naive T cells and induce an entirely new adaptive immune response with all its features, including memory (see above section). There are several subsets of DCs but, in essence, there are two major groupings: resident, non-migratory DCs which are located in the secondary lymphoid tissues such as the spleen and the lymph node and migratory DCs which arise in the bone marrow and migrate to the tissues where they are available as sentinels to register pathogens and other danger signals and transport antigen to the secondary lymphoid tissues where they activate the adaptive immune system (T and B cells). Tissues at the front line of attack, e.g. lungs, skin, gut mucosa and conjunctiva, are rich in DCs (see eFig 7-2 ) .
Several types of dendritic cell have been identified on the basis of cell surface markers (CD markers, see p. 381 ). Two main subsets are recognized: plasmacytoid DC and conventional DC ( eFig. 7-2 ). Both are derived from precursors in the bone marrow, the myeloid-derived precursor cells (MDP), which also generates precursor cells for the macrophage lineage. Such precursors circulate in the blood and seed the tissues in homeostatic conditions where they provide a surveillance function for pathogens and danger signals. They also proliferate and respond rapidly to danger and can become inflammatory DCs. Some of these are derived from monocytes which can become either tissue inflammatory macrophages (see above) or become Tip DCs (TNF-α, NO-producing DCs) which are active in antigen presentation and generation of the immune adaptive immune response.

Studies on DC lineage and phenotype have mostly been performed in the mouse and human. DC subsets and markers are defined by several different cell surface markers which are variably expressed on different DC subtypes ( www.RnDsystems.com ). For a summary of how DCs control adaptive immunity, see review by Miriam Merad and colleagues and a summary in the accompanying poster on www.biolegend.com ( ).
Tissue-resident DCs constantly traffic from the bone marrow to the tissues and then to the secondary lymphoid organs. If they do not detect ‘dangerous’ antigens, but only self-antigens, they do not activate the adaptive immune system and in fact maintain immune tolerance by sustaining the population of endogenous Tregs, i.e. it seems that, although DCs have the powerful potential to rapidly activate adaptive immunity, e.g. in the case of a viral infection, their homeostatic (and probably main function) is to maintain tolerance.
Other granulocytes
Mast cells/basophils/eosinophils are all part of the granulocyte series of cells. Basophils (life span: 2–3 days) are the circulating equivalent of mast cells (life span: weeks), which occur only in the tissues. However, mast cells and eosinophils may have separate lineages and so their ontogeny is not yet decided.
Two types of mast cell are described: the connective tissue mast cells and the mucosal mast cells on the basis of their granule proteases, their susceptibility to degranulation by immunoglobulin E (IgE) and their requirement for different maturation signals (see below). Mucosal mast cells line epithelial and connective tissue mast cells line endothelial (blood vessel) barriers and are thus important innate immune cells, contributing to the initial response to external pathogens or endogenous danger signals by releasing mediators and increasing vascular permeability ( Fig. 7-1 ). Mast cells have a further important role of engineering the contraction of the innate immune response.

Eosinophils account for about 0.1% of circulating leucocytes. Their numbers are elevated in chronic allergic disorders, in both the circulation and the tissues. They are also produced in large numbers in response to parasite and helminth infections and contain a panel of particularly potent parasite-specific proteases. Like mast cells they have high-affinity IgE receptors, and are probably effectors of tissue damage in allergic disease, including asthma and chronic allergic conjunctival disorders such as atopic keratoconjunctivitis and vernal keratoconjunctivitis (see p. 445 ). Interestingly, eosinophils are major components of allergic disease, and have been reported to be prominent in fungal antigen-driven allergy. In this respect, eosinophils, like mast cells and basophils, are classical biomarkers of type 2 immune responses (see p. 418 ) and are associated with alternatively activated (M2) macrophages. As such they promote not only immune homeostasis but also metabolic homeostasis by promoting glucose tolerance and preventing fat deposition.
Natural killer cells are an infrequent but important constituent of innate immune cells, although they are technically lymphoid cells. Their main function is to provide defence against virally infected cells and tumour cells which they perform in an antigen-independent manner. Classically they are considered not to generate immunological memory, which is the domain of the adaptive immune system, and so they are not contributors to long-term protective immunity. They express the surface antigen CD56 at both high and low levels. In humans they express the KIR (‘killer’ inhibitory receptor) family of receptors and in mice a range of Ly-49 antigens which allow subsets of NK cells to be identified and which vary between strains of mice. NK cells use inhibitory receptors to prevent them responding to healthy cells which express MHC class I (‘self’) antigens (see p. 424 ) but respond to stressed cells which may have low levels of MHC class I (absent self-antigen) and kill such cells using cytotoxic mechanisms.
NKT cells are a further set of innate immune cells of lymphoid origin which also express a single invariant T-cell receptor which binds CD1 antigens (combined with glycolipid antigens, see p. 412 ), and thus like NK cells they are essentially involved in innate immune responses to microbial lipid, particularly from Gram-negative microorganisms expressing the glycolipid, glucuronyl ceramides.
The lymphoid system and acquired immunity
The two most important features of the acquired immune system are exquisite antigen specificity and immunological memory. These are properties of lymphocytes. In contrast to cells of the myeloid system, which remove debris and organisms by mechanisms that have limited specificity (thus macrophages will phagocytose broad ranges of organisms using ‘pattern recognition receptors’ (PRRs) to recognize PAMPs; see p. 389 ), each clone of lymphoid cell responds to a single antigen. T cells respond to antigen by proliferating and releasing cytokines, while B cells respond by maturing to plasma cells and producing antibodies.
T cells
T cells (for thymus-derived) are lymphoid mononuclear cells that recognize antigen in conjunction with self-antigen. T helper (Th) cells respond to antigen in association with MHC class II self-antigen, while T cytotoxic (Tc) cells respond to antigen combined with MHC class I antigen (see p. 424 ). T cells release cytokines, which are required for T-cell and B-cell proliferation and differentiation, and also for innate immune cell activation. T lymphocytes express surface markers (molecules detectable by specific monoclonal antibodies) characteristic of their phenotype. Thus Th cells are described as CD4 + cells and Tc cells are known as CD8 + cells ( Box 7-4 ).
The letters CD mean cluster of differentiation; this term refers to a molecule that has a defined structure, that can be recognized by a group or cluster of monoclonal antibodies, and that identifies a specific lineage or differentiation stage in the cell. CD numbers are continually being defined and the 10th Human Leukocyte Differentiation Antigen (HLDA10) workshop took place in conjunction with the Australasian Society of Immunology in December 2014. At HLDA9 there were 363 CD labelled genes/molecules.
Further details can be obtained through the following link: www.hcdm.org
Some of the better-known CD numbers are shown below.
CD No. | Cell/molecule | Function/role |
---|---|---|
1 | Thymocytes, Langerhans’ cells | |
3 | T cells | |
4 | T helper cells | Adhesion |
8 | T cytotoxic cells | |
11a | Leucocytes (αL chain of LFA-1) | Adhesion |
11b | Leucocytes (αM chain of LFA-1) | Adhesion |
11c | Leucocytes (αX chain of LFA-1) | Adhesion |
19 | B cells | |
25 | Activated lymphocytes, IL-2 receptor and macrophages | |
28 | T cells | Co-stimulation |
40 | Many cells | Co-stimulation |
44 | T and B cells | Activated memory cells |
45 | Common leucocyte antigen | |
45RA | Naive T cells | |
45RO | Memory T cells | |
56 | NK cells | |
62E,L,P | E, L and P selectins | Adhesion |
68 | Macrophages | |
69 | Many cells | Short-term activation |
95 | Many cells | Fas |
120 | TNF-α receptor | |
142 | Tissue factor | Coagulation |
152 | Cytotoxic T lymphocyte antigen-4 | Immunoregulation |
169 | Sialoadhesin | Macrophages |
183 | CXCR3 | Chemokine |
284 | Toll-like receptor 4 | Myeloid cells |
332 | FGF receptor | growth factor |
363 | SIP receptor | lymphocyte homing |
FGF, fibroblast growth factor receptor; LFA, leucocyte functional adhesion molecule; IL, interleukin; NK, natural killer; TNF, tumour necrosis factor; SIP, sphingosine-1-phosphate.
This general categorization of T cells (CD4 and CD8, T cells) was found to be insufficient to explain the numerous different functions of T cells. After many years of controversy over whether a T-cell subset existed which suppressed (regulated) the immune response, the discovery of a T cell which was required to prevent spontaneous autoimmune disease indicated that regulatory T cells (Tregs) occurred in normal healthy individuals.
T cells, particularly CD4 + T cells, are defined by the cytokines they produce and the transcription factors they utilize. Cytokines are multifunctional short-acting, short-range mediators of cellular activities, released by T cells and other immune and non-immune cells (see p. 399 ). Cytokines are distinguished from other mediators as the molecules of ‘intercellular communication’. In addition to CD4 + Tregs, CD4 + T cells now include several subsets such as Th1, Th2, Th17, IL22-secreting CD4 + T cells and it is likely that this T cell specialization will be increasingly recognized ( Fig. 7-2 ). Subsets of Tc cells and B cells (including B regulatory cells) with defined roles in pathogenesis of disease have been described.

Further subsets of T cells occur, such as the γδT cells (which possess a T-cell receptor (TCR) with a γ–δ dimer, rather than the αβ TCR present on conventional T cells) and NKT cells which combine properties of innate immune NK cells but possess an αβ T-cell receptor.
B cells
B cells are mononuclear lymphoid cells that are specialized for the secretion of antibody. There are five types (isotypes) of antibody (IgG, IgA, IgM, IgD and IgE) and B cells require ‘help’ from T cells to function.
During a primary immune response, IgM antibody is initially produced by activated B cells. In secondary immune responses, the B cells switch to producing IgG (isotype switching), often with higher binding capacity (affinity) for the antigen, a process termed affinity maturation. During allergic immune responses, a further isotype switch occurs from IgG to IgE. IgA forms part of the mucosal immune system, being present in large amounts in surface secretory fluids including tears. IgA is the most abundant immunoglobulin in the immune system. IgD is present in low amounts in the circulation.
B cells recognize and bind antigen via surface immunoglobulin (sIg) which is in effect the B-cell receptor. Antigen binds to sIg in the afferent phase of the secondary immune response; in contrast, antigen binds to the secreted form of immunoglobulin (antibody) in the effector phase of the response. Secondary afferent (cognitive) interactions are antigen-specific, but effector functions are not (see below).
B-cell subsets also exist such as marginal zone B cells and B1 cells, which like γδ T cells and NKT cells lack diversity of αβ T cells.
Blurring the margins between innate and adaptive immunity
Some cells do not fit into this simple scheme separating innate from adaptive immunity. These includes natural killer (NK) cells, NKT cells and lymphoid tissue inducer (LTi) cells, all of which belong to the recently rebranded set of innate lymphoid cells (ILCs). γδT cells and some of the subsets of B cells described above also probably belong here.
Natural killer cells
Natural killer (NK) cells are circulating granulocytes but are part of the lymphoid system and are particularly effective against tumour cells and virus-infected cells. Previously called null cells because they lack any of the specific lymphocyte markers including a T-cell receptor, they recognize and kill virus-infected cells when activated for instance by the cytokine IL-2. Similarly, they have receptors for antibody allowing them to kill antibody-coated cells. NK cells are characterized by inhibitory receptors which bind MHC class I, thus preventing them from killing uninfected healthy cells. In contrast, NKT cells are specialized lymphoid cells that only express a single type of TCR which responds to lipid antigen presented via the molecule CD1 on antigen-presenting cells (thus they are known as invariant NKT cells).
Lymphoid tissue inducer cells
LTis have characteristics of both NK cells and lymphocytes, but are specialized to produce lymphotoxin-α and -β (LTα, LTβ) which are required for the development of lymph nodes and other secondary lymphoid tissue.
Innate lymphoid cells
ILCs lack both αβ and γδ T-cell receptors, a feature which could include them in the myeloid system, but have lymphocyte characteristics. Thus NK cells and LTis can be regarded as one type of ILC. However, three further subsets of ILC have recently been identified which secrete cytokines normally associated with CD4 T cells, such as IFN-γ (Th1-like), IL-4 (Th2-like) and IL-22/IL-17 (TH17-like).
Initial response of the host to injury (the innate immune response)
The acute inflammatory response is the host’s initial reaction to challenge. During this response, invasive pathogens are removed by cells of the innate immune system brought to the site of injury by changes in tissue components such as blood vessels and extracellular matrix. Meanwhile antigen from degraded microorganisms is transported to lymphoid tissues to activate the acquired immune system.
The acute inflammatory response
The acute inflammatory response goes through three phases:
- •
tissue damage and the acute early response
- •
the delayed cellular response and phagocytosis
- •
resolution of the inflammation and tissue remodelling.
- •
tissue damage and release of mediators
- •
vascular changes
- •
leucocyte activation and adhesion
- •
leucocyte emigration.
Tissue damage and the release of mediators
The response to tissue injury (physical, chemical or mediated by microorganisms) is immediate. Reactions occur at several levels, both locally and systemically. Immediate local reactions include the release of tissue factors and chemoattractants (chemokines, see p. 404 ) from damaged tissue and microorganisms. Vessels are also damaged, inducing venous stasis and the leakage of plasma components; platelet and leucocyte activation with intravascular clotting occurs; plasma/serum transudation and exudation lead to tissue fibrin deposition and activation of serum components such as complement (see p. 405 ).
There are several classes of inflammatory mediators derived from both inflammatory and damaged tissue cells:
- •
vasoactive amines (e.g. histamine and serotonin)
- •
cytokines and chemokines
- •
lipids (e.g. prostaglandins, thromboxane and leukotrienes)
- •
free radicals (see Ch. 4 , Box 4.4-8 , p. 193 )
- •
neuropeptides (e.g. substance P, vasoactive intestinal peptide)
- •
endothelium-derived mediators (endothelin, nitric oxide, prostacyclin, platelet-activating factor, etc.)
- •
plasma-derived mediators (e.g. complement, kinins and clotting cascade peptides)
- •
leucocyte-derived mediators (e.g. granule proteases, phospholipase A 2 )
- •
bacterial products (e.g. endotoxin, proteases and chemotactic factors including formylated peptides).
Class | Agent |
---|---|
Free radicals/gases | Hydrogen peroxide |
Hypochlorite | |
Chloramine | |
OH radical | |
Nitric oxide | |
Enzyme | Proteinase 3 |
Collagenase | |
Elastase | |
Azurocidin | |
Cathepsin G | |
β-glucuronidase | |
Myeloperoxidase | |
Lysozyme | |
Peptide | Defensin |
β-lysin | |
Vasoactive intestinal peptide | |
Ion binders | Lactoferrin |
Calprotectin |
MMP | Interstitial collagens | Basement membrane | Elastin | Other proteins |
---|---|---|---|---|
Collagenases | ||||
MMP-1 | Types III, I, II, VII, X | Laminin | ||
Entactin | ||||
MMP-8 | Types I, III, II | Fibronectin | L-selectin | |
MMP-13 | Types II, I, III | (±) Proteoglycan | ||
Stromelysin | ||||
MMP-3 | Fibronectin | ± | EGF-like proteins | |
Laminin | ||||
MMP-10 | Entactin | ± | Plasminogen | |
Proteoglycan | ||||
Stromelysin-like | ||||
MMP-7 | Fibronectin | + | Plasminogen | |
MMP-12 | Laminin | ↓ | ||
Entactin | ++ | Angiostatin; | ||
Proteoglycan | α 1 -antitrypsin | |||
Gelatinases | ||||
MMP-2 | Types I, VII, X, XI | IV/V | ++ | |
Fibronectin | ||||
MMP-9 | Laminin | ++ | ||
Entactin | ||||
Proteoglycan | ||||
Membrane-type | ||||
MMP-14 | Types I, III, II | Fibronectin | ||
MMP-15 | Laminin | |||
MMP-16 | Entactin | |||
MMP-17 | Proteoglycan | |||
MMP-24 | ||||
Furin-recognition site | ||||
MMP-11 |

However, much of the cell and tissue damage is mediated by free radicals, particularly hydrogen peroxide (H 2 O 2 ) and superoxide anions (see Ch. 4 , p. 193 ), which are released as part of the respiratory burst ( Box 7-5 ). Interestingly flavonoids and adenosine, both important molecules in ocular physiology, inhibit the respiratory burst.
The neutrophil respiratory burst describes the activation of neutrophils and their utilization of oxygen during the inflammatory cascade. The stimulus for this response is the release of mediators from injured tissue cells. Tissue damage is caused by free radical release and tissue proteases (see also Ch. 4 ).

The central component of the respiratory burst is H 2 O 2 , which is metabolized through several pathways, some of which cause further tissue damage (e.g. superoxide and chloramine) while others reduce it to water. Tissue damage is therefore dependent on the levels of reduced glutathione (GSH) in the milieu. In addition, during the respiratory burst, immune cells activate a Na( + )/H( + ) membrane exchanger protein which is required to control intracellular pH and cell volume. NADP, nicotinamide adenine dinucleotide phosphate; NADPH, reduced NADP; PKC, protein kinase C.

Hypochlorous acid (HOCI) is a short-lived, highly reactive oxidant that is lipophilic and membrane permeant. It binds proteins and renders them more susceptible to proteases. Chlorinated proteins are more immunogenic and may provide a link between the innate and the acquired immune systems by acting as ‘DAMPs’.
Free radicals may also combine with reactive nitrogen species (nitric oxide) released from inflammatory cells ( Box 7-6 ).
Nitric oxide was originally described as endothelium relaxing factor because it was found to be the agent released by the endothelium that was responsible for inducing autocrine vasodilatation in response to insult. Nitric oxide is a gas produced by the activity of the enzyme nitric oxide synthase (NOS) on interaction of the amino acid arginine with oxygen:

There are at least three isoforms of nitric oxide synthase, endothelium-derived (eNOS), neuronal (nNOS) and inducible (iNOS). The iNOS is released from inflammatory and other cells, particularly macrophages, and is involved in both immunoregulation and tissue damage through its interaction with superoxide radicals released from activated neutrophils. In its latter role it may also function as an antibacterial agent by damaging bacterial cell membranes. The prostanoids are co-released with NO through induction of cyclo-oxygenase-2.
NO interacts with superoxide anions to produce per-oxynitrite, which is believed to be directly involved in membrane damage (see equation below). NO has direct effects on many other proteins such as the important zinc finger regions of many enzymes where it nitrosylates free cysteine SH groups with ejection of the Zn moiety from the configured protein. These damaging effects to parenchymal cells may also be the basis for T-cell apoptosis (cell death), so leading to downregulation of the immune response.
O 2 • + NO • → ONOO − + H • ⇌ ONOOH → HO • + NO 2 + → NO 3 − + H +
Thus NO produced constitutively in small amounts has a physiological role involving guanyl cyclase and increases in cGMP, while in large amounts it may be cytotoxic, producing depression of mitochondrial respiration, metal enzyme damage with consumption of both Zn and Fe ions and DNA damage.
Vascular changes
The immediate cause of inflammation is release of plasma into the extravascular space and the instantaneous coagulation of proteins with activation of inflammatory mediators. Plasma release (vascular leakage) is caused by changes to the blood vessels induced by the inflammatory mediators, particularly reactive nitrogen species (RNS), especially in conditions such as the ischaemia–reperfusion damage of stroke and vascular occlusion. The immediate response of the vascular endothelium is to undergo retraction and this is associated with transient vasoconstriction. The major vascular response, however, is vasodilatation mediated initially by nitric oxide (NO). The initial vasoconstriction is mediated by several locally released compounds, particularly endothelin, which is released by pericytes and smooth muscle cells to act on the endothelium. The later vasodilatation is also mediated by locally released factors, in this case the gas nitric oxide. NO is synthesized by specific enzymes from the amino acid arginine and has widespread physiological and pathological effects, some of which are related to its role as a free radical (see Box 7-6 ). This is accompanied by an increased blood flow, the opening of capillary channels and the leakage of plasma into the extracellular space. This in turn leads to an increase in the tissue osmotic pressure, thus attracting further fluid build-up in the tissues (oedema). In response to this, there is an increase in lymphatic drainage from the injured site, thus reducing the tissue swelling and at the same time increasing the flow of antigenic material to the draining lymph nodes (see below). These vascular changes vary in degree with the severity of the tissue injury and protective measures are often in place such as the expression of caveolin-1 in vessel walls which mitigates the increased vascular permeability.
The vascular endothelium also undergoes significant functional and morphological changes during the inflammatory response. Whereas the normal endothelium presents a non-adhesive surface to circulating cells such as platelets and leucocytes, during inflammation the endothelium becomes much more adhesive, an effect achieved by the expression of specific adhesion molecules on its surface. There are three major classes of adhesion molecule, the selectins, the integrins and the cell adhesion molecules (CAMs), each with different functions (see below) ( Fig. 7-4 and Table 7-3 ). In addition, the endothelium may undergo a marked morphological transformation, changing from a flat resting cell to a large protruding cell with multiple cytoplasmic organelles. These inflammation-associated vascular changes occur almost exclusively in the postcapillary venule and, in this respect, this region resembles the high endothelial venule cells in the lymph node, which are specialized for leucocyte adhesion (see below).

Endothelium | Leucocyte | |
---|---|---|
Loose attachment | P-selectin | PSGL-1, PTX3 |
E-selectin | PSGL-1, ESL, CD44 | |
PSGL, GlyCAM | L-selectin | |
Slow rolling | ICAM-1 | LFA-1/PSGL-1 |
E-selectin | PSGL-1, ESL, CD44 | |
Arrest/firm adhesion | ICAM-1 | LFA-1 |
VCAM-1 | VLA-4 | |
Hyaluronan | CD44 | |
Crawling | ICAM-1 | MAC-1 |
Extravasation | ICAM-1, ICAM-2 | LFA-1, MAC-1 |
VCAM-1 | VLA-4 | |
CD99 | CD99 | |
PECAM-1 | PECAM-1 | |
JAM-A, -B, -C | LFA-1, VLA-4, MAC-1 | |
CD99L2 | ? |
Leucocyte activation and adhesion to the endothelium
Neutrophils are attracted to the site of inflammation through a series of discrete events that occur during margination and extravasation of the cell from the vessel. These involve rolling, loose attachment, firm adhesion and then extravasation/migration of the cell through the intercellular junction. Each of these steps is mediated by the reciprocal expression of adhesion molecules and their respective ligands on the surface of leucocytes and the endothelium (see below) ( Table 7-3 ). During the later stages of the response (24–72 h) when other inflammatory cells are involved (monocytes and lymphocytes), similar adhesion mechanisms are involved but with different sets of molecules. Thus, the coordinated expression of adhesion molecules appears to regulate the nature of the inflammatory cell exudate.
Adhesion of leucocytes to the endothelium thus involves a series of molecular events
- •
Selectin–ligand (S–L) interactions occur during the initial rolling phase of leucocyte endothelial cell interactions. These are initially low-strength interactions and are enhanced by the upregulation of selectins on the endothelium by inflammatory cytokines such as interleukin-1 (IL-1) and TNF-α or by contact with an activated T cell.
- •
Leucocyte activation by chemokines is mediated in part through upregulation of specific chemokine receptors (see later) which induces polarization and firm adhesion of the cell to the endothelium.
- •
Integrin–CAM interactions induce spreading of the leucocyte on the endothelial cell surface and prevent detachment of the leucocyte.
- •
Extravasation of leucocytes through the endothelium is mediated by expression of PECAM-1 (CD31) on both the leucocyte and the endothelium, possibly through a ‘zipper’ mechanism in which disassembly of intercellular tight (occludin) and adherens junctions occurs with the expression of several junctional adhesion molecules (JAMs; see Table 7-3 ). CD99, expressed on both leucocytes and endothelium, is also important in transendothelial migration of monocytes.
- •
Migration of leucocytes through the tissues is the final stage and is induced through binding of chemokines selective for each cell type which activate signalling pathways in the translocating cell activating the motor machinery (actin–myosin cytoskeleton) to propel the cell forward up the chemotactic gradient ( Fig. 7-5 ).
FIGURE 7-5
Neutrophil migration up a chemical gradient. ( A ) Cells sense the concentration gradient and polarize towards it with a wide leading edge and a trailing tail. Receptors for the chemoattractant cluster in the membrane of the leading edge, increasing the signal at this end of the cell. ( B ) Diagram of the seven-coil transmembrane receptor (the same basic structure as rhodopsin) that mediates the intracellular signal via Gprotein links. This activates a protein, tyrosine kinase, to initiate actin assembly and induce forward movement of the cell.
Leucocyte migration into the tissues and chemotaxis
Many of the mediators released in the earliest stages of tissue injury are attractants for inflammatory cells. Both thrombin and the cleavage product fibrinopeptide B from fibrin lead to leucocyte chemotaxis from the onset of vessel leakage and fibrin formation. Prokaryotic peptides released from bacteria, such as formyl-methionine-leucine-phenylalanine, are powerful neutrophil and monocyte chemotactic agents. Activated complement components (see p. 405 ) have an important role as chemoattractant agents in the neutrophil/monocyte response. Other important chemoattractants include interleukin-8 (IL-8), a cytokine (chemokine) released from tissue cells including the retinal pigment epithelium (RPE), tumour necrosis factor-α (TNF-α) and platelet release compounds such as platelet-activating factor (PAF), transforming growth factor-β (TGF-β), platelet-derived growth factor, platelet-derived endothelial cell growth factor, and many others. Lipid mediators, such as the leukotrienes, are also important neutrophil chemoattractants, while certain cytokines, such as monocyte chemotactic protein (MCP) and the macrophage inflammatory proteins (MIP-α, MIP-β and the chemokines; see below) are selective for mononuclear cell chemotaxis. Cytokines that induce inflammatory cell migration are known as chemokines (inducing movement through chemotaxis) and receptors for different chemokines are present on different cells, thus regulating not only the numbers of cell that migrate into the tissue but also the type of cells and thus the quality of the inflammatory exudate (see p. 375 ).
Cells such as neutrophils and monocytes ‘sense’ a chemical gradient of these attractants and migrate up the gradient by using specific cell surface receptors clustered preferentially towards the leading, polarized edge of the cell ( Fig. 7-5 ). These receptors (e.g. the C5a receptor) are composed of seven transmembrane segments (in a manner similar to the transmembrane spanning segments of rhodopsin; see Ch. 4 , p. 261.e1 ) that possess a cytoplasmic connection to a Gprotein-linked second messenger system. This activates the intracellular machinery (actin–myosin motor) required for forward movement. Recent studies have also shown that activation of G protein receptors occurs in waves, thus enhancing the overall effect of the gradient.
Phagocytosis and removal of damaged tissue and microorganisms
Recovery from inflammation requires the removal of dead microorganisms and necrotic tissue by phagocytic cells (polymorphonuclear leucocytes and macrophages). Even in the absence of microorganisms, altered (damaged) self-proteins are recognized by cells of the innate immune system and phagocytosed. In the eye this is classically seen with lens-induced uveitis, in which denatured lens crystallin proteins are released into the anterior chamber in cases of traumatic and hypermature cataract. In the latter circumstance, engorged macrophages may block the outflow channels and produce a ‘phacolytic glaucoma’ (see Ch. 9 , p. 512 ).
Phagocytosis, particularly of bacteria, is facilitated by certain molecules of the innate immune system known as opsonins, which are present in plasma and bind to the surface of microorganisms when they are released into the extracellular space. One of the complement components, C3b (see below), acts as an opsonin. Interestingly, different actin cytoskeletal structures are constructed to phagocytose different types of particle, e.g. IgG-coated versus complement-coated particles. In addition, the role of microbial pathogen receptors such as Toll-like receptors (see next section) on maturation of the phagosome is unclear but appears not to play a significant part.
Molecules of the acquired immune system also promote phagocytosis, particularly antibodies which bind avidly to the foreign antigen by specific interaction of their antigen-binding site (the Fab portion of the molecule) but are non-specifically removed by binding of their Fc portion to the phagocyte cell surface (see section on antibodies below, p. 396 ). Cells that express high levels of surface receptors for C3b and Fc are termed ‘professional phagocytes’, and include neutrophils and macrophages. Studies in genetically targeted knockout mice suggest that the Fc pathway for phagocytic activation is the major one.
Activation of innate immune cells by microorganisms
Invading microorganisms release factors that attract leucocytes to the site of inflammation and also induce those same leucocytes to engage in attempted removal of the offending agent. This requires activation of the immune cells. How is this activation of the innate immune response achieved? As indicated in the introduction, this was previously considered to occur by non-specific pathogen–host cell interaction, i.e. there was one general innate immune mechanism whereby the body reacted to many different types of microorganisms. However, it has long been recognized that responses to different organisms vary greatly, some being virulent or lethal while others are harmless, and this is partly due to how different classes of microorganisms activate the initial innate immune cells they encounter. This they do via broadly specific ligands (PAMPs) which bind to pathogen-recognition receptors (PRRs) (see p. 391 ). There are several classes of both membrane and soluble PRRs, including the Toll-like receptors (TLR) ( Table 7-4 ), carbohydrate-binding (C)-type lectins, retinoic acid-inducible gene-1 (RIG-1) helicases, nucleotide-binding oligomerization domain protein (NOD)-like receptors (NLRs), the scavenger receptors and some soluble PAMPS such as the collectins (including complement proteins) and acute phase proteins. The components (PAMPs, DAMPs) recognized by the PRRs include pathogen cell wall material, bacterial DNA and proteins, viral DNA and RNA, and lipoproteins from microbes as well as endogenous ligands such as self-DNA (if it is in the wrong place, such as the cytoplasm) and particulate material such as uric acid and cholesterol crystals.
TLR | Localization | Pathogen-derived agonists | Endogenous agonists | Synthetic agonists |
---|---|---|---|---|
TLR1 and TLR2 | Extracellular | Bacteria: peptidoglycan, lipoproteins, LTA Fungi: zymosan | – | Pam 3 Cys |
TLR2 and TLR6 | Extracellular | Bacteria: lipoproteins | Veriscan | MALP2 |
TLR3 | Intracellular | Viruses: dsRNA | mRNA | Polyl:C |
TLR4 | Extracellular | Bacteria: LPS Viruses: RSV fusion protein Fungi: mannan Protozoa: glycoinositolphospholipids | Saturated fatty acids, β-defensins, oxLDL * , amyloid-β * | Lipid A derivatives |
TLR5 | Extracellular | Bacteria: flagellin | – | – |
TLR7 and TLR8 | Intracellular | Viruses: ssRNA | Self-RNA | Imiquimod, R-848 |
TLR9 | Intracellular | Bacteria: CpG DNA Viruses: CpG DNA Protozoa: CpG DNA, haemozoin | Self-DNA | CpG-ODNs |
TLR11 | Extracellular | Uropathogenic bacteria Protozoa: profilin-like molecule | – | – |
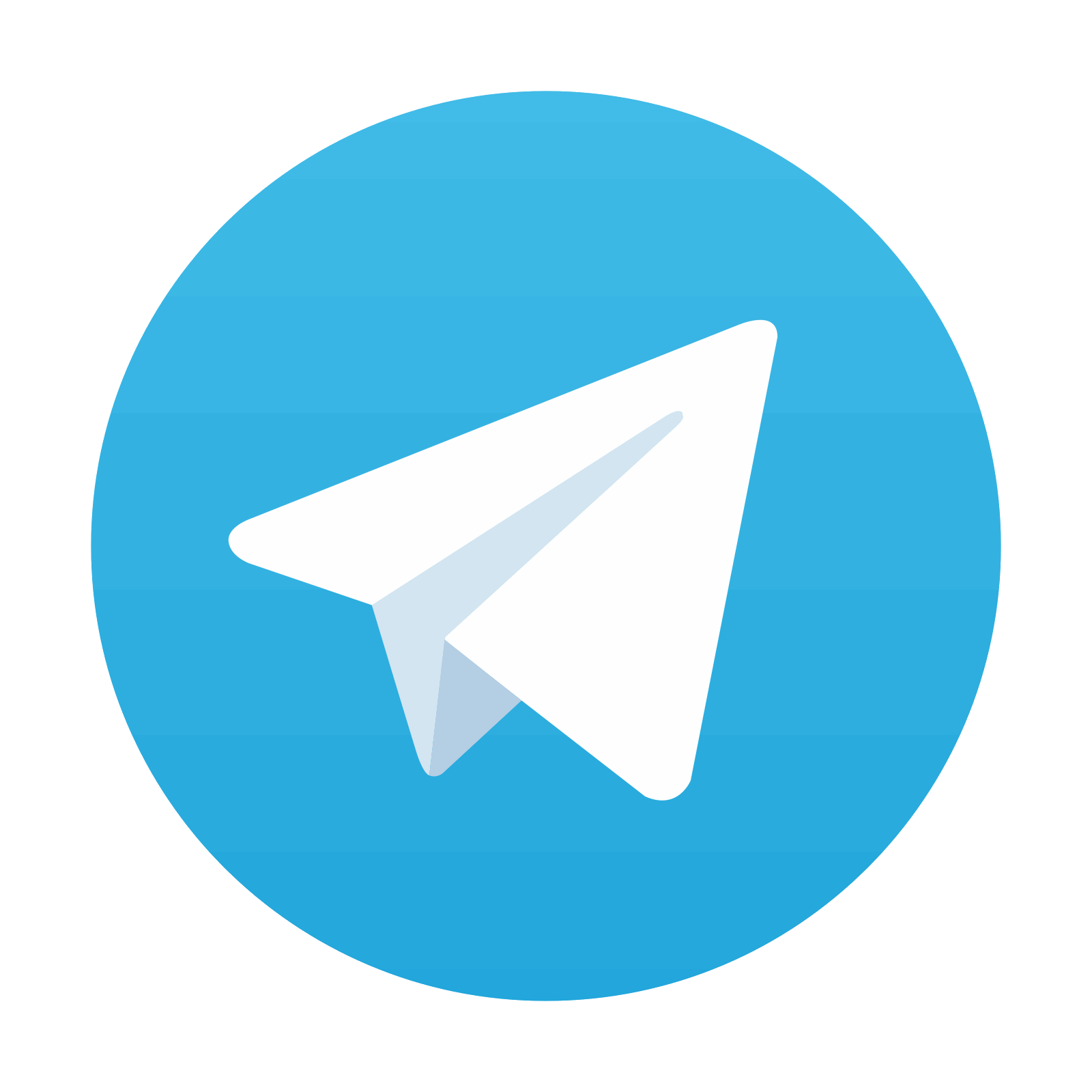
Stay updated, free articles. Join our Telegram channel

Full access? Get Clinical Tree
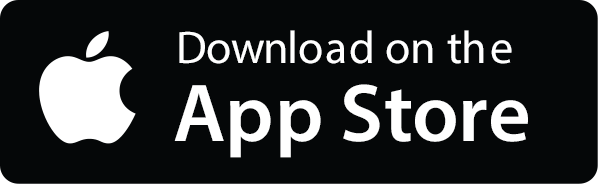
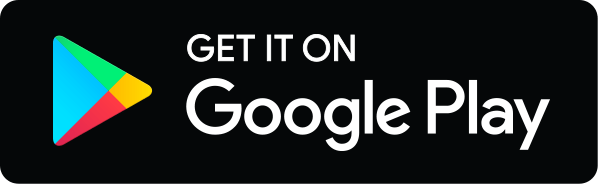
