Fig. 8.1
Ultrasound biomicroscopy image of an eye implanted with Boston keratoprosthesis. Note the poor details of the contact lens (CL), assembled keratoprosthesis (KPro), and corneal graft as well as the artifact below the KPro base
8.3 Anterior Segment Optical Coherence Tomography
8.3.1 Introduction
AS-OCT, first described in 1994, is a noncontact, noninvasive tool that can provide high-resolution cross-sectional images of the anterior segment to the depth of the iris plane [20, 21]. The first-generation AS-OCT uses 1,310 nm wavelengths and time domain technology (TD-OCT), which produces A-scans by varying the position of the reference mirror [22]. However, the speed of the TD-OCT is limited by the mechanical cycle time of the movement of the reference mirror [21]. Commercially available TD-OCT devices include the SL-OCT (Heidelberg Engineering, Heidelberg, Germany) and the Visante OCT (Carl Zeiss Meditec, Dublin, CA), which allows for approximately 18 μm resolution and 2000 A-scans per second [23, 24].
Newer-generation OCT uses Fourier or spectral domain technology (SD-OCT), in which the interference between the sample and the reference reflection is detected as a spectrum and Fourier transformation is used to produce the A-scan [21–23]. It uses a reference mirror that is stationary, which enables improved image acquisition speed, reduces the impact of motion artifact, and improves image definition. Commercially available SD-OCT devices include Cirrus HD-OCT (Carl Zeiss Meditec Inc, Dublin, CA), Spectralis Anterior Segment Module (Heidelberg Engineering, Heidelberg, Germany), and RTVue (Optovue, Inc., Fremont, CA). However, SD-OCT devices have shorter wavelengths (840 nm) and smaller scan widths than TD-OCT, which allows the latter to have deeper tissue penetration and a wider area of capture in a single image [22].
For KPro, these advances in AS-OCT have allowed for cross-sectional images of the implanted device and visualization of otherwise poorly seen anterior chamber structures (Figs. 8.2 and 8.3). AS-OCT was found to be a superior imaging technique to UBM and provided high-resolution images of the implanted KPro, bandage contact lens, device-carrier cornea interface, donor graft, anterior chamber, iris, and angle [19].
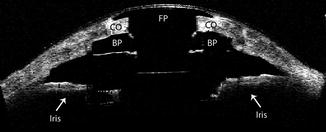
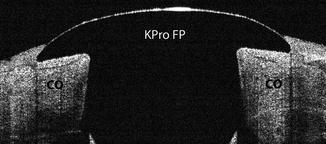
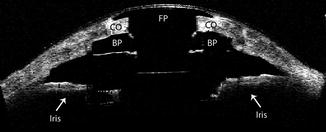
Fig. 8.2
Visante (time domain) anterior segment OCT scan demonstrating the implanted Boston type 1 keratoprosthesis. FP front plate, BP back plate, CO donor cornea
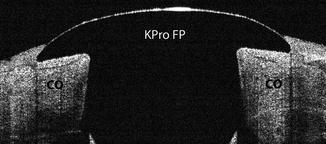
Fig. 8.3
Optovue (spectral domain) anterior segment OCT scan showing the implanted Boston type 1 keratoprosthesis with high resolution of the front plate (KPro FP) and donor graft (CO). Note the apposition of the front plate and the donor corneal graft
However, disadvantages of AS-OCT include requirement of fixation during image acquisition and no transmission through metal (titanium) or the pigmented posterior layer of the iris, allowing for no visualization of the posterior chamber.
8.3.2 Keratoprosthesis-Donor Cornea Interface
Evaluation of the integrity of the device-donor cornea interface is important because potential complications such as infection, desiccation, and melting around the stem may occur. The potential space that exists between the device and donor cornea may allow access to microbial organisms and proteolytic enzymes from the extraocular fluid into the eye, increasing the risk of endophthalmitis and corneal melt. However, theoretically this potential space should be closed and remain watertight because the front plate and back plate are secured by the titanium locking ring [25].
AS-OCT has been used to image the device-donor interface, as detailed evaluation is limited by slit lamp examination (Fig. 8.4). Garcia et al. assessed the anatomic stability of this interface and found that of 15 eyes imaged only two were visualized to have a gap between the front plate and the donor cornea [25]. With pressure application on the globe, none of the eyes had any change in the device-donor interface (including gaping or aqueous fluid escape) and were thus deemed anatomically stable. However, Fernandez et al. found that 44 % of eyes imaged with spectral domain AS-OCT identified a gap, measuring an average of 40 μm in the vertical dimension (range of 8–104 μm,) between the front plate and the donor cornea in at least one image quadrant [26]. But, as the authors note, some eyes had a very small size space between the device-donor interface, and the gap size that may be clinically significant is yet to be established.
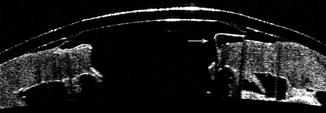
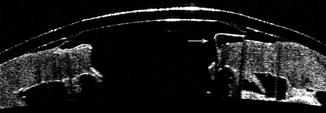
Fig. 8.4
Imaging of the device-donor interface using Visante AS-OCT. A significant vertical gap is demonstrated between the posterior surface of the front plate and the carrier donor graft, which may lead to a corneal melt (white arrow)
In addition, stromal thinning typically begins adjacent to the KPro stem, where the front plate covers the donor cornea, and early detection is not always possible on slit lamp examination [2]. Early recognition of stromal thinning and tissue recession is important as it may lead to extensive thinning and subsequent corneal melt and device extrusion. The rate of corneal melt has been reported to range from 6.1 to 25 %, with a higher incidence in patients with autoimmune ocular surface disease, and often necessitates KPro explanation and replacement with another KPro or penetrating keratoplasty [14, 27, 28]. However, early stromal thinning and tissue retraction are easily detected by AS-OCT, and prompt treatment of aggressive lubrication, anti-collagenolytic, and anti-inflammatory therapies can be initiated (Fig. 8.5).
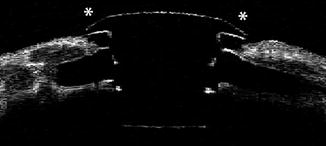
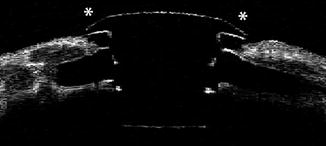
Fig. 8.5
AS-OCT imaging of the device-donor interface demonstrating stromal thinning and tissue recession that has led to corneal melt (asterisk)
Epithelial migration over the device-cornea interface and KPro optic has also been described, initially in a case report in 2010 (Fig. 8.6) [29]. Subsequently, Fernandez et al. observed that in the majority of eyes (83 %) in their study, epithelium extended onto this junction and appeared as a highly reflective layer extending from the donor cornea over the edge of the front plate on AS-OCT [26]. This was confirmed to be corneal epithelium by light microscopy and immunohistochemical analysis [30]. Epithelial migration across the device-graft junction may be clinically beneficial as it may constitute a barrier to microorganisms and proteolytic enzymes from the tear film. However, further investigation is needed to determine how epithelialization of the device-donor interface occurs and its clinical benefits and consequences.
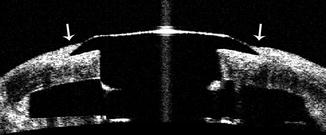
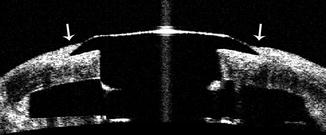
Fig. 8.6
AS-OCT scan of the junction between the keratoprosthesis device and donor cornea. Epithelial tissue is easily visualized on AS-OCT and is observed to extend onto the keratoprosthesis front plate (arrow)
8.3.3 Retroprosthetic Membrane (RPM)
Retroprosthetic membrane (RPM) formation is the most frequent complication after KPro implantation, with a reported incidence of 25–65 % of cases [5, 31]. In a large multicenter study, the Boston Type 1 Keratoprosthesis study group found the rate of RPM development to be 31.7 % [10]. The most significant risk factors for membrane formation were preoperative surgical indications of infectious keratitis and aniridia [10]. Treatment of clinically significant RPM is by Nd:YAG laser membranotomy or surgical removal if the membrane cannot be treated adequately with laser.
The development of RPM is a clinical diagnosis, with the retro-optic portion of the membrane easily documented by slit lamp examination. However, with AS-OCT imaging, both the retro-optic and retro-back plate portion of the membrane can be well visualized (Fig. 8.7). Garcia et al. first described the AS-OCT appearance of RPM as a thickening of the base of the front plate stem and found detailed views of the membrane by coronal scans [19].
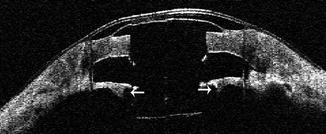
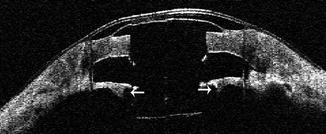
Fig. 8.7
Cross-sectional AS-OCT scan demonstrating the implanted keratoprosthesis. Posterior to the back plate, a thick retroprosthetic membrane can be seen (arrows). Note the characteristic concaving sloping of the membrane adjacent to the keratoprosthesis stem and the discrete shadowing across the membrane at the outer margin of the titanium locking ring
Sivaraman et al. observed that visualization of a retro-back plate membrane on AS-OCT was associated with a concurrent retro-optic membrane seen on clinical exam; however, the converse was not observed in 28.6 % of patients [32]. This suggests that clinical examination alone may be insufficient to detect retro-back plate membrane formation. It is clinically important as retro-back plate RPM may be a risk factor of corneal melt due to significant occlusion of back plate holes and restriction of access of nutrition from the aqueous to the donor graft [2, 32]. This is further supported in that thicker membranes were found in patients with corneal melt compared to eyes that did not melt, as increased thickness of membranes is likely to result in greater obstruction of aqueous diffusion. Features of retro-back plate RPM on AS-OCT include a characteristic concave sloping of the RPM adjacent to the KPro stem, continuity of the membrane with the donor cornea, and discrete shadowing across the membrane at the outer margin of the titanium locking ring [32].
8.3.4 Anterior Chamber and Angle Anatomy
Evaluation of the anterior chamber structures and angle anatomy has become increasingly more important after KPro surgery. Gradual synechial closure of the anterior chamber angle has been theorized as one of the multiple mechanisms of de novo glaucoma development or glaucoma progression after KPro implantation. Thus, evaluation of the anterior segment and angle using AS-OCT may be helpful in the early detection and monitoring of secondary angle closure.
In a retrospective series of 52 eyes that underwent AS-OCT imaging at a single time point postoperatively, Kang et al. observed that the majority of eyes had shallow anterior chamber depths, extensive angle closure, and peripheral and proximal synechiae formation [33]. In fact, in 25 eyes with all representative meridians of the 360° angle imaged, an average of 8.68 clock hours of angle closure was observed. In a prospective series using qualitative serial AS-OCT images captured in the horizontal meridian, Panarelli et al. also found that four of ten patients developed synechial angle closure during the first year after KPro surgery [34]. Further collaborating these results, Kang et al. performed a prospective quantitative evaluation of the angle status using preoperative and serial postoperative 360° AS-OCT imaging in 11 eyes [35]. They found that KPro implantation induced significant progressive angle closure in 63.6 % of patients as well as a shallowing (mean of 1.59 mm) of the anterior chamber depth (Fig. 8.8). In addition, 72.7 % of eyes had synechiae formation postoperatively with 7.3 and 6.7 clock hours of peripheral anterior synechiae and iris-back plate touch, respectively [35]. The understanding of these anatomical relationships and structural changes of the angle may have clinical implications for the detection and management of progressive angle closure.
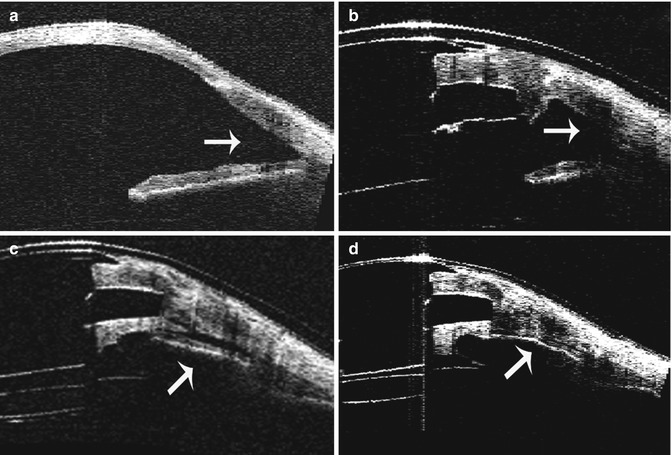
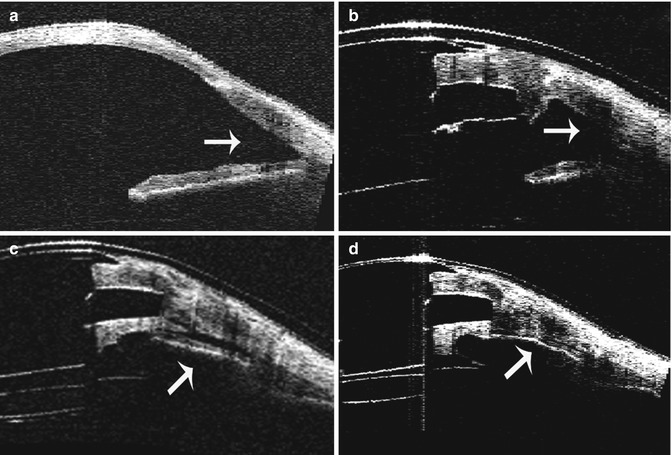
Fig. 8.8
Serial AS-OCT imaging of a patient with implanted keratoprosthesis at the 180° meridian. (a) Preoperative AS-OCT showing an open anterior chamber angle. (b) AS-OCT image of the same eye at 1 month post keratoprosthesis implantation showing an open angle. (c) AS-OCT image at 3 months postoperatively with progressive shallowing of the anterior chamber angle. (d) AS-OCT scan at 6 months postoperatively of the same eye demonstrating a closed angle with iridocorneal adhesion
8.4 Diagnostic Imaging Tools in Glaucoma
Glaucoma is a significant cause of postoperative visual loss or limited visual recovery in otherwise successful KPro surgery. The progression of preexisting glaucoma (7–23 %) or the de novo development of glaucoma (2–28 %) has been well documented in previous studies [5, 8, 9, 36–39]. Although direct assessment of the optic nerve and visual field testing are important components of glaucoma evaluation, the lack of an accurate and reproducible method to measure intraocular pressure renders glaucoma detection challenging. Consequently, other imaging modalities may play a critical role in evaluating and monitoring glaucoma after KPro.
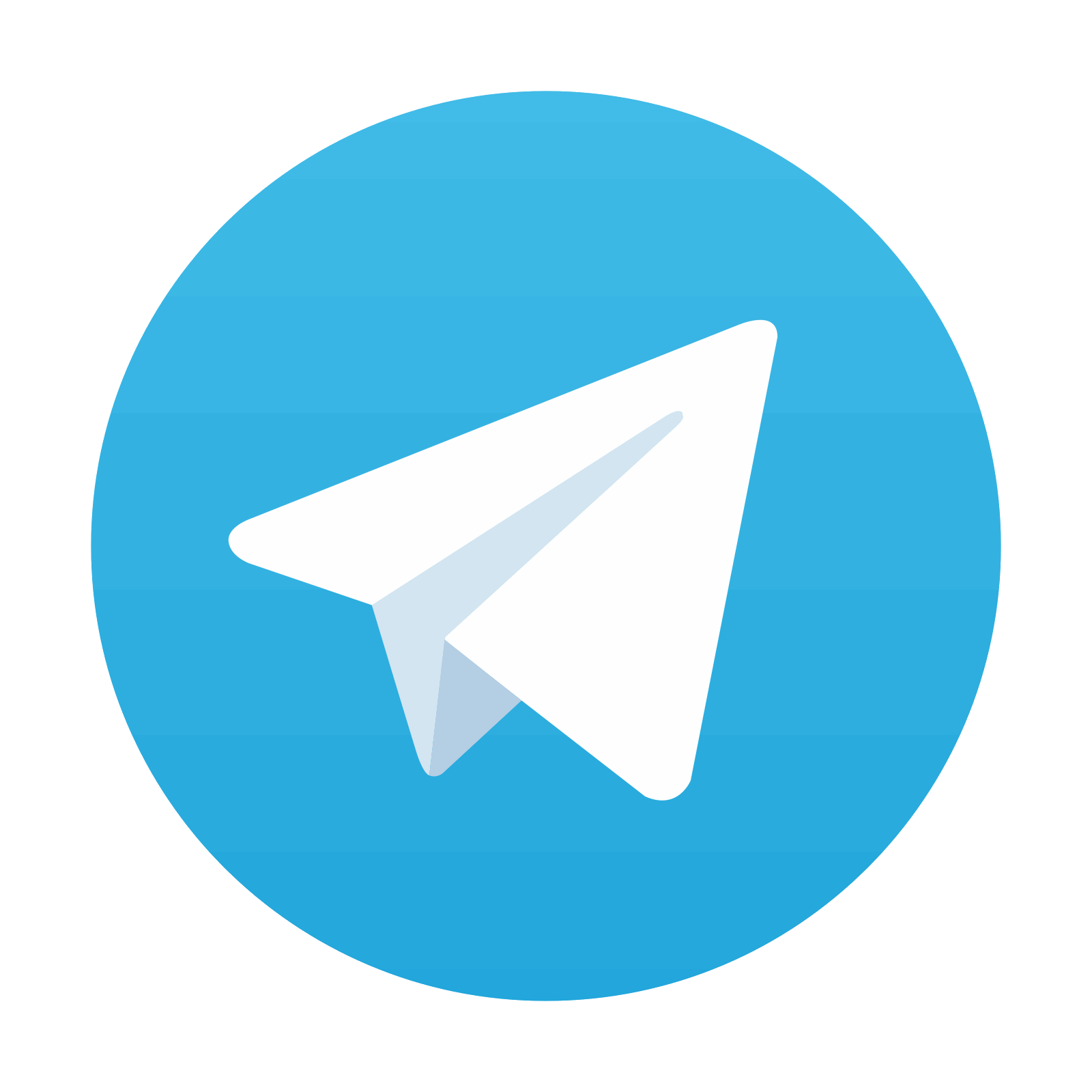
Stay updated, free articles. Join our Telegram channel

Full access? Get Clinical Tree
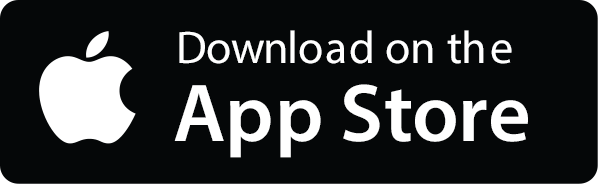
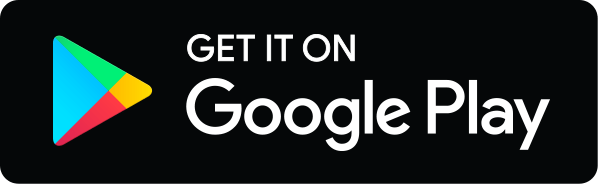