Heredity of Refractive Errors
Donald O. Mutti
Karla Zadnik
Debates about the etiology of refractive error have often been characterized by more heat than light, but that state of affairs is changing thanks to improved technology and the considerable efforts of researchers at the molecular and patient-based levels. A simple dichotomy of heredity or environment as etiological factors is being transformed by a rich array of new data into a more complex understanding of precisely defined roles and interrelationships for each. Questions such as “what genes might be important in refractive error,” “what does the function of these genes tell us about the etiology of refractive error,” “what is the relative strength of the contribution of heredity vs. environment to refractive error,” and “what in the environment might influence the growth of the eye” have all been recently and more thoroughly addressed.
The human genome presents the molecular geneticist with about 3.2 billion base pairs containing an estimated 25,000 to 30,000 protein-encoding genes.1,2 Each million base pairs are roughly equal to a centimorgan (cM), a segment of DNA with a probability for recombination during meiosis of 1%.3 The size of the genome in terms of centimorgans is estimated to be 2,809 cM in males and 4,782 cM in females.1 Two general approaches are used in genetic studies of disease: linkage and association [see Young (2007), Christensen and Murray (2007), Iyengar (2007), and Tang et al. (2008) for recent reviews].4,5,6,7 Linkage analysis is designed to identify a region of a chromosome that is inherited along with the disease phenotype because the disease gene is in some proximity to genetic markers, or sequence variations of known location within that region. This proximity is determined from the recombination fraction (θ), the probability of recombination between homologous chromosomes during meiosis. This value lies between θ = 0 for loci that are very close together and therefore are always transmitted together and θ = 0.50 for loci that are far apart and, therefore, recombine randomly between the two chromosomes in a pair. Families are required for these studies because of the need for “informative” meioses to occur. The log odds (LOD score) are then calculated describing the log of the ratio of the odds of obtaining the observed pattern of inheritance if the marker is linked to the disease gene at a particular value of θ divided by the odds of obtaining the observed pattern of inheritance if the marker is not linked to the disease gene and θ = 0.50. The LOD score is calculated over a range of values of θ from 0.50 to 0 to find the maximum positive LOD score. A LOD score of 3 or more is generally considered strong evidence of linkage. The smaller the value of θ at this maximum positive LOD, the closer the marker is to the putative disease gene locus.
An association study is more allele specific than region specific. The question posed in an association study is whether a certain marker or single nucleotide polymorphism (SNP) is over-represented in diseased individuals compared to controls or to the general population. This design is similar in concept to a case-control study in risk factor research (Schlesseman [1982] is a classic text in this area).8 Families are not necessarily required for an association study, but, if present, can provide information on both linkage and association. The assumption is that the marker will be over-represented if it is in fact close to the disease gene or is the disease-causing mutation itself. False positives are possible, however, if the marker is in proximity to alleles that are indirectly related to the disease, such as alleles that are over-represented in a particular ethnicity with a high prevalence of disease, but are not the cause of the disease itself. In the space of only about 10 years since their development, these techniques have provided a wealth of information on the heredity of refractive error at the molecular level.
The molecular genetics of refractive error
There are 14 regions that have been given a MYP designation by the Human Genome Organisation Gene Nomenclature Committee (Table 1). The linkage to Xq28 (MYP1) was found in an extended pedigree descending from inhabitants of the Danish island of Bornholm, hence the designation Bornholm Eye Disease for the phenotype.9 Affected individuals displayed myopia, typically high myopia (median = -8.50 D), although lower levels of myopia were also considered affected, along with optic nerve hypoplasia (although Young et al. (2004) notes that this may just be temporal conus),24 thinning of the retinal pigment epithelium, visible choroidal vasculature, and subnormal dark-adapted ERGs. All the affected men also had deutan color vision defects. The authors suggested that Bornholm Eye Disease might represent a mutation among color blindness genes resulting in cone dysfunction, myopia, and amblyopia, or that the deutan defects were merely closely liked to the genes responsible for Bornholm Eye Disease.9 Young et al. (2004) studied a Minnesota family descended from inhabitants of other Danish islands close to Bornholm and found linkage to the same Xq28 region as for Bornholm Eye Disease.24 These subjects had protan color vision defects, however, and showed evidence of two independent mutations of the same gene. The authors concluded that the cone abnormalities were most likely secondary to the myopia and that the color vision defects were unrelated to the disease state. Not all X-linked myopia is linked to this region, however (a list of studies that either confirm or fail to confirm loci from other investigations can be found in Table 2). Zhang et al. examined two Han Chinese families with inheritance of high myopia (-6.00 D or more myopia) occurring in an X-linked manner.19,20 Color vision defects were present but not related to the myopia. Affected individuals showed temporal crescents typical of high myopes, an abnormal ERG, and reduced visual acuity. No mention is made of the optic nerve hypoplasia found in Bornholm Eye Disease. Significant linkage was found at Xq23-25 (MYP13) over an interval of 25 cM (14.9 Mb; DXS1210 to DXS8057) in one family. The second family showed significant linkage at Xq25-27.2 (also MYP13) over an interval of 37.3 cM (24.11 Mb; DXS1001 to DXS8043). There was a 4.8 cM (3.68 Mb) area of overlap in the linkage results for these two families, hence the same designation of MYP13 for each study.19,20 The MYP1 region is 6 Mb to 17 Mb away from these regions and not linked to myopia in these families. Several candidate genes are mentioned for this region, although none has been shown yet to have functional significance in myopia: retinal guanylyl cyclase 2F (GUCY2F, involved in the resynthesis of cGMP), glutamate dehydrongenase 2 (GLUD2), glutamate receptor, ionotrophic, AMPA 3 (GRIA3), baculoviral IAP repeat-containing protein 4 (BIRC4, relevant to apoptosis), stromal antigen 2 (STAG2), and leucine-rich repeats and calponin homology (CH) domain containing 2 (LRCH2).19 A complete list of candidate genes in the regions linked to myopia can be found in Table 2.
Table 1. Summary of currently identified genetic loci related to refractive error. | |||||||||||||||||||||||||||||||||||||||||||||||||||||||||||||||||||||||||||||||||||||||||||||||
---|---|---|---|---|---|---|---|---|---|---|---|---|---|---|---|---|---|---|---|---|---|---|---|---|---|---|---|---|---|---|---|---|---|---|---|---|---|---|---|---|---|---|---|---|---|---|---|---|---|---|---|---|---|---|---|---|---|---|---|---|---|---|---|---|---|---|---|---|---|---|---|---|---|---|---|---|---|---|---|---|---|---|---|---|---|---|---|---|---|---|---|---|---|---|---|
|
Table 2. Summary of confirmation status of genetic loci related to refractive error with listing of candidate genes at these loci. | ||||||||||||||||||||||||||||||||||||||||||||||||||||||||||||||||||||
---|---|---|---|---|---|---|---|---|---|---|---|---|---|---|---|---|---|---|---|---|---|---|---|---|---|---|---|---|---|---|---|---|---|---|---|---|---|---|---|---|---|---|---|---|---|---|---|---|---|---|---|---|---|---|---|---|---|---|---|---|---|---|---|---|---|---|---|---|
|
Families of Chinese/Hawaiian or European ancestry were identified by having early onset (before age 12 years) high myopia (-6.00 D or more myopia) in two or more generations in studies linking myopia to 18p11.31 (MYP2).10,11 Pathologies such as Stickler syndromes, Marfan syndrome, or juvenile glaucoma were excluded. Among the 25 regulatory or structural candidate genes within the region mapped, the authors felt that the α subunit of laminin (LAMA1) was the candidate gene with the most relevance to myopia. Laminin is an elastic component of human sclera that may play a structural role by binding scleral elaunin and oxytalan fibers to collagen fibers.48 Finer mapping using linkage disequilibrium identified a marker near the original interval in the 18p11.31 region as the most significant (D18S52).11 A marker separated by about 0.8cM (D18S63) but within this 18p11.31 region was also highly significantly associated with high levels of myopia in a community in Sardinia.25 This may represent multiple mutations of a single gene or mutations of several nearby genes. Further evidence of genetic complexity within this chromosomal region was provided by Lam et al. in a sample of Chinese families with a history of high myopia (-6.00 D or more) in at least two generations.26 Two widely spaced markers, perhaps 5.4 Mb apart, one within (D18S476) and one outside (D18S62) of the interval reported by Young et al. (2001),11 were significantly linked with myopia in this sample. There was no evidence of heterogeneity, again suggesting the influence of multiple genes perhaps acting in concert with environmental effects. The 18p11.31 region is not uniformly linked to high myopia, however. Evidence for heterogeneity in this trait comes from Naiglin et al. (1999) who studied 18 French families with myopia of -6.00 D or more and found no linkage to 18p11.31 region, nor to collagen genes COL1A1, COL1A2, COL3A1, to proteoglycan genes for versican (VCAN) or aggrecan (ACAN), nor to five loci corresponding to Stickler and Marfan syndromes.27 Interestingly, all types of myopia may not be created equal, genetically speaking. More common levels of lower myopia (-0.75 D or -1.00 D) have not been linked to this region either.29,30 Despite mild evidence for linkage in a few families, the more homogeneous sample and adequate statistical power rule out a substantial role for the 18p11.31 region in milder forms of myopia.29 Transforming growth factor-β-induced factor homeobox 1 (TGIF1) is in the region 18p11.31 within the interval mapped for MYP2. SNP analysis by Lam and co-workers found that one polymorphism (657 T→G) was significantly associated with a protective effect in 71 Hong Kong Chinese subjects with high myopia (-6.00 D or more myopic) compared to 105 controls with less myopia than -2.00 D.31 Scavello et al. (2004) also examined polymorphisms within the interval containing TGIF1 and MYP2 but found that none of the numerous sequence variations found in this region was associated with high myopia in families with MYP2-associated high myopia.32 SNPs within TGIF1 were also not associated with myopia in a sample of 330 Japanese subjects with high myopia (more myopic than -9.25 D) compared to 330 controls who were less myopic than -4.00 D.33 These different results might be attributed to either ethnic variation (Chinese in the Lam et al. study, Northern European in heritage in the Scavello et al. sample, Japanese in the study by Hasumi et al.),31,32,33 SNP choice, or that TGIF1 is not the gene for high myopia in the MYP2 region. Zhou and Young (2005) evaluated the gene for lipin-2 (LPIN2), more for its proximity to marker D18S481 on 18p than for any obvious functional significance.35 Seven subjects with MYP2-associated myopia were compared with six nonmyopic controls. No polymorphisms were associated with myopia, and none resulted in amino acid substitutions. The Young laboratory (Scavello et al., 2005) also reported negative results for seven other candidate genes within 18p11.31: clusterin-like 1 (retinal; CLUL1), elastin microfibril interfacer 2 (EMILIN2), myomesin 1 (MYOM1), myosin regulatory light chain 2 and 3 (MRCL2, MRCL3), discs, large (Drosophila) homolog-associated protein 1 (DLGAP1), and zinc finger protein 161 homolog (mouse; ZFP161).34
In the ten years since the discovery of the first two genetic loci for myopia, heterogeneity has been the dominant theme as numerous other regions have been linked to myopia. Yet there have been no strong biological connections made between the physiological processes that result in myopia and the genes within these regions. How these chromosomal regions produce myopia remains a mystery. Young et al. (1998) found that chromosome region 12q21-q23 (MYP3) was linked to the same phenotype of myopia onset (before age 12 years) and high myopia (-6.00 D or more) in two or more generations of an Italian/German family.12 Markers for MYP2 were not linked to myopia in this family. MYP2, MYP3, and MYP5 were evaluated in 51 families in the UK with at least two siblings with myopia of at least -6.00 D.28 Results indicated that families could have myopia due to significant linkage with MYP3, but that only 24% (95% CI, 2% to 48%) of the families were so linked. There was not significant and consistent evidence of linkage to MYP2 and only weak evidence of linkage to MYP5. Stickler syndromes loci COL2A1 and COL11A1 and Marfan locus FBN1 were also not linked to myopia in these families.28 More common levels of lower myopia (-0.75 D or -1.00 D) were also not linked to the MYP3 region.29,30 The candidate genes within this region include phenylalanine hydroxylase (PAH), lumican (LUM), decorin (DCN), and dermatan sulfate proteoglycan (DSPG3, currently epiphycan, EPYC).12 The authors felt that lumican and decorin were the more likely candidates as they are present in corneal stroma and in sclera.12 Lumican may have a role to play in scleral developmental. Mice with a lumican deficiency by virtue of being either heterozygous or homozygous for a null mutation in the lumican gene show enlargement of collagen fibers that the authors speculate could lead to alterations in ocular shape.49 These genes perhaps interact with fibromodulin because double-null mice exhibit thin sclera, increased axial length, and retinal detachment – all traits in common with human high myopia.50 Neither dermatan sulfate nor decorin mRNA levels changed during the course of induction of or recovery from minus-lens induced myopia in the tree shrew.51,52 However, markers for LUM, fibromodulin (FMOD), EPYC, keratocan (KERA), and DCN have not been related to the myopia trait in MYP3-related pedigrees.36 One SNP (rs3759223, C→T) in LUM was significantly overrepresented in a sample of 120 highly myopic (more myopia than -10.00 D) Han Chinese subjects in Taiwan compared to 137 control subjects with refractive error between than -1.50 D and +0.50 D.37 No associations were found between myopia and SNPs in DSPG3 (EPYC) or DCN.37
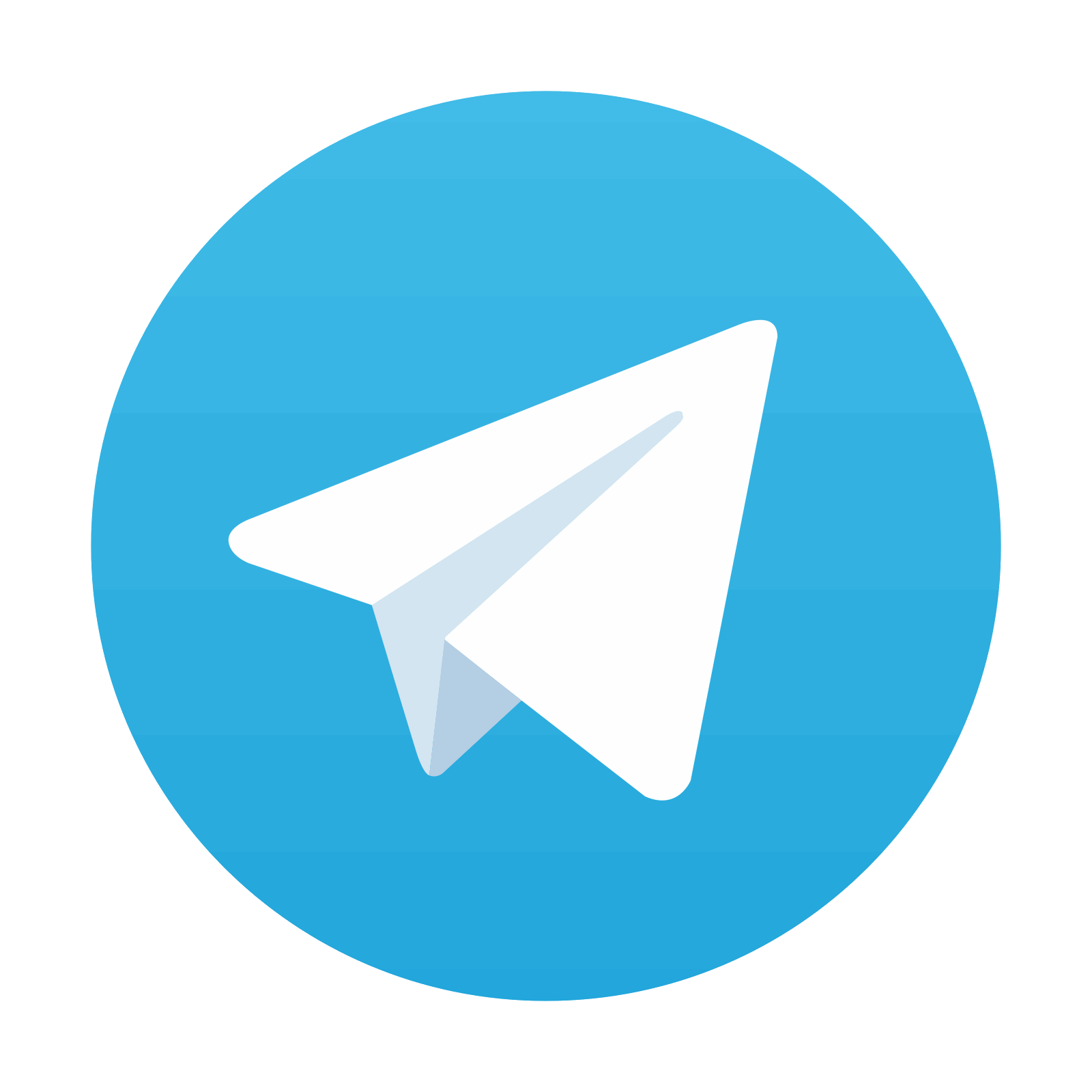
Stay updated, free articles. Join our Telegram channel

Full access? Get Clinical Tree
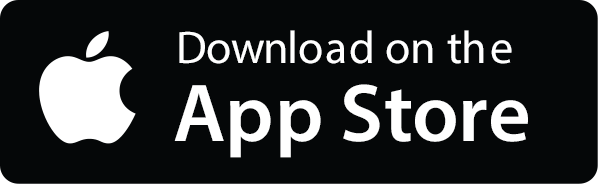
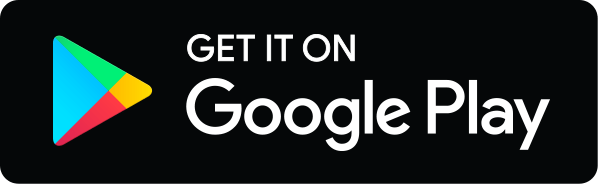