Head and Neck Imaging
Tanya J. Rath
Due to advances in cross-sectional imaging, computed tomography (CT), magnetic resonance imaging (MRI), and ultrasound have largely replaced conventional radiography in head and neck imaging and are invaluable primary imaging modalities used to evaluate pathology in the head and neck. They are complementary to a thorough physical exam and essential to characterize the deep tissue extent of mucosal lesions as well as evaluate pathology originating in the other spaces of the neck. Nuclear medicine has poor spatial resolution but provides useful functional and metabolic information. This chapter provides an overview of the various imaging modalities available in the head and neck as well as describes the application, strengths, and weaknesses of these modalities as they pertain to head and neck imaging. A thorough discussion of the physics of these modalities and image interpretation is beyond the scope of this chapter and is described in detail in several dedicated imaging and physics textbooks (1,2,3,4).
COMPUTED TOMOGRAPHY
Imaging Principles
CT employs the use of ionizing radiation to image patients. An x-ray tube rotates 360 degrees around the patient, while a well-collimated x-ray beam is generated and passed through the patient. The beam is partly absorbed or scattered as it passes through the patient. Tissues of different density and thickness attenuate the beam to varying degrees. A circumferential bank of detectors opposite the beam measures the degree of x-ray transmission through the patient. Each volumetric imaging unit (voxel) is assigned a numeric value, called a Hounsfield unit (HU). Water is assigned a value of 0 HU. The matrix of HUs is then displayed in a gray scale, resulting in an anatomic cross-sectional image. Various algorithms can be applied to optimize evaluation of different structures such as bone, soft tissue, or lung parenchyma (Fig. 11.1).
Most scanners are now multidetector helical or spiral scanners in which the patient translates through the x-ray tube while it continuously rotates around the patient. Using this design, spatial resolution is improved and large volumes can be imaged with reduced scan time, resulting in greater anatomic coverage with decreased motion artifact. Data can be acquired in less than 1-mmthick slices and reconstructed at various intervals in the axial, coronal, and sagittal planes. Images can be obtained during quiet respiration or in a short single breath hold and the entire neck can be scanned in less than 1 minute.
Applications and Technique by Region of Interest
Temporal Bone
CT is the study of choice to evaluate osseous integrity of the temporal bone including the otic capsule, ossicular chain, cortical plates, and air spaces. Main indications include evaluation of temporal bone fracture, congenital anomalies of the otic capsule, conductive hearing loss, inflammatory disease, and erosive neoplastic processes affecting the temporal bone.
Direct coronal images are typically no longer necessary due to high-resolution helical multidetector imaging. A small field of view is employed with a highresolution matrix. Technique is optimized to evaluate bony detail and 0.5- to 0.625-mm-thick axial images and thin-cut coronal reconstructions are routinely provided (Fig. 11.2). Additional oblique reformats such as Stenvers view and Pöschl view can be useful to demonstrate semicircular canal dehiscence (Fig. 11.3). All data are displayed in bone algorithm windows. Thicker 2.5-mm axial images to evaluate the soft tissues and posterior fossa structures are useful as well. Contrast is typically not necessary but can be helpful in evaluating the soft tissues
and intracranial compartment for complications related to otomastoiditis. It may also be useful in the evaluation of tumors involving the temporal bone, particularly if MRI is contraindicated.
and intracranial compartment for complications related to otomastoiditis. It may also be useful in the evaluation of tumors involving the temporal bone, particularly if MRI is contraindicated.
Central Skull Base
Skull base CT technique is similar to the temporal bone in that it is typically performed without contrast and displayed in high-resolution bone algorithm with axial, sagittal, and
coronal reconstructions using a typical slice thickness of 1.25 mm. However, the field of view is larger, focused on the central skull base, including the basiocciput and the sphenoid bone. The main indications for skull base CT include evaluation of fractures, dysplasias, primary bone lesions of the skull base, and secondary neoplastic involvement of the skull base. It nicely demonstrates the characteristic “groundglass” appearance of fibrous dysplasia. This is particularly useful when fibrous dysplasia is incidentally found on MRI where it can be easily mistaken for a skull base tumor due to its variable signal characteristics and enhancement pattern (Fig. 11.4). CT evaluates the osseous integrity of the skull base and skull base foramina affected by neoplastic processes including but not limited to nasopharyngeal carcinoma, pituitary lesions, chordoma, chondrosarcoma, meningioma, Langerhans cell histiocytosis, metastasis, and myeloma. Enlargement or distortion of skull base foramina related to perineural spread of neoplasm can sometimes be appreciated, though direct imaging of perineural spread of neoplasm and intracranial extension is better demonstrated by MRI.
coronal reconstructions using a typical slice thickness of 1.25 mm. However, the field of view is larger, focused on the central skull base, including the basiocciput and the sphenoid bone. The main indications for skull base CT include evaluation of fractures, dysplasias, primary bone lesions of the skull base, and secondary neoplastic involvement of the skull base. It nicely demonstrates the characteristic “groundglass” appearance of fibrous dysplasia. This is particularly useful when fibrous dysplasia is incidentally found on MRI where it can be easily mistaken for a skull base tumor due to its variable signal characteristics and enhancement pattern (Fig. 11.4). CT evaluates the osseous integrity of the skull base and skull base foramina affected by neoplastic processes including but not limited to nasopharyngeal carcinoma, pituitary lesions, chordoma, chondrosarcoma, meningioma, Langerhans cell histiocytosis, metastasis, and myeloma. Enlargement or distortion of skull base foramina related to perineural spread of neoplasm can sometimes be appreciated, though direct imaging of perineural spread of neoplasm and intracranial extension is better demonstrated by MRI.
Maxillofacial Structures and Sinonasal Cavities
CT is the first study of choice to evaluate the osseous structures and adjacent soft tissues of the face and the sinonasal cavity, particularly in the setting of trauma or suspected inflammatory sinonasal disease. Normal anatomy and anatomic variations, mucosal disease and osseous integrity of the anterior skull base, and maxillofacial structures are well demonstrated.
Evaluation of the paranasal sinuses with plain film radiography has largely been replaced with CT. At some institutions, a screening sinus CT is performed to look for inflammatory disease with thick cuts in the axial or coronal plane performed at skip intervals with the technical parameters optimized for low-radiation exposure similar to plain films. This technique, however, does not well evaluate the anatomy and all walls of the sinonasal structures. It has fallen out of favor with the advent of helical multidetector CT and CT dose reduction strategies.
A high-resolution helical CT of the sinonasal structures is performed if detailed evaluation of maxillofacial trauma, sinus inflammatory disease, odontogenic disease, sinonasal neoplasm, or anatomy for presurgical planning is required. The widespread availability of helical multidetector CT precludes the previous need for direct coronal imaging to demonstrate the anterior ostiomeatal unit and replicate the endoscopic view of the paranasal sinuses. A typical protocol includes contiguous helical scanning with 1.25-mm-thick images obtained through the paranasal sinuses with multiplanar reformats created in the axial, sagittal, and coronal planes. The mandible can be included in the field of view if required. In the setting of trauma, three-dimensional (3-D) volume-rendered shaded surface display reconstructions can be helpful for planning craniofacial reconstruction, creating 3-D models and evaluating surgical results. Advantages of high-resolution helical imaging with multiplanar reformats include patient comfort, better demonstration of the anterior and posterior walls of the sinuses, and decreased motion and dental amalgam artifact. Contrast is not required for routine inflammatory sinus disease or trauma. In patients with acute odontogenic infection, contrast delineates associated soft tissue abscess and cellulitis. It can be helpful in defining the extent of sinonasal neoplasms or complications of sinusitis such as orbital cellulitis, subperiosteal abscess, or intracranial abscess (Fig. 11.5).
Orbit
Both CT and MRI are useful for evaluation of the orbit. CT is typically the initial study of choice when patients present to the emergency room with acute orbital symptoms. MRI is preferable if intracranial pathology or optic neuritis is suspected on the basis of clinical and physical exam. MRI better images the optic nerve and nerve sheath complex as well as the intracranial compartment, which can be affected when patients present with visual symptoms. Noncontrast CT is useful in cases of orbital trauma, evaluation of osseous orbital lesions, foreign body, calcifications, and thyroid orbitopathy (Fig. 11.6). In cases of acute
orbital cellulitis, contrast-enhanced orbital CT is the first study of choice. Thin-cut images are obtained in the axial plane and reconstructed in the coronal and sagittal planes to better demonstrate the relationship of the optic nerve to the extraocular muscles.
orbital cellulitis, contrast-enhanced orbital CT is the first study of choice. Thin-cut images are obtained in the axial plane and reconstructed in the coronal and sagittal planes to better demonstrate the relationship of the optic nerve to the extraocular muscles.
Neck, Soft Tissues
A standard contrast-enhanced soft tissue neck CT evaluates the suprahyoid and infrahyoid soft tissues of the neck, including the aerodigestive tract, lymph nodes, glandular structures, and larynx. While soft tissue contrast is greater with MRI, contrast-enhanced CT is frequently the first study of choice in the evaluation of a neck mass or mucosal lesion of the upper aerodigestive tract because of its short acquisition time, universal availability, and reduced cost as compared to MRI. Generally speaking, neck CT is the preferred modality for evaluation of sialolithiasis, inflammatory neck processes, posttraumatic evaluation of the neck soft tissues, and evaluation of osseous cortex. There are few indications for noncontrast neck CT, including sialolithiasis, evaluation of patients with thyroid cancer who may require future treatment with radioactive I-131 and evaluation of neck pathology in patients with an absolute contraindication to IV administration of iodinated contrast. Contraindications to iodinated contrast administration include advanced renal insufficiency (not on dialysis), acute renal failure, and history of anaphylactic reaction to iodinated contrast. Patients with a history of milder allergic reactions to iodinated contrast should receive pretreatment with steroids to reduce the likelihood of a contrast reaction.
Good soft tissue contrast can be achieved with most lesions in the neck when iodinated contrast is administered and appropriate technique is employed with contrast present in both arteries and veins during the image acquisition. Contrast administration technique is critical to delineating the mucosal-submucosal interface, optimizing mucosal enhancement, and maximizing lesion detectability (Fig. 11.7). Using a helical scanner, 100 to 150 mL of contrast is administered via a power injector with an initial delay in image acquisition following the contrast injection to ensure adequate arterial and venous opacification. Exact protocols vary according to the scanner and institution. Scanning too early during the arterial phase may cause poor soft tissue contrast and lesion detectability, resulting in a false-negative study.
A routine contrast-enhanced neck CT typically extends from above the internal auditory canals caudally through the aortic arch, to include the course of the left recurrent laryngeal nerve (Fig. 11.8). Images are displayed at a slice thickness of 2 to 3 mm in the axial plane in both soft tissue and bone algorithm. In patients with dental amalgam or metal causing streak artifact, reangled images to avoid dental amalgam should be obtained because it can obscure pathology (Fig. 11.9). Coronal and sagittal reconstructions are commonly used to supplement axial images.
Modifications to a soft tissue neck CT protocol can be tailored to an area of interest or specific clinical question. In cases of a laryngeal mass, thin axial reconstructions through the larynx parallel to the laryngeal ventricle in bone and soft tissue algorithm are created to evaluate the extent of the lesion, paraglottic extension, and evidence of laryngeal cartilage erosion. Coronal reconstructions are helpful to evaluate for transglottic spread of tumor in the paraglottic space. At some institutions, additional images using the modified Valsalva maneuver (exhaling against a closed glottis or blowing through a straw) and phonation (patient exhales and makes the sound “eeee”) are obtained, which dilates the laryngeal ventricle and pyriform sinuses in an attempt to better demonstrate the extent of tumor and anatomy. The added benefit of such techniques is controversial and must be weighed against added radiation exposure (5,6). In patients with a buccal mucosal lesion, a “puffed cheek” technique can be used to increase conspicuity of the lesion (7). If sialolithiasis is suspected, a noncontrast CT through the major salivary glands and oral cavity can be performed to evaluate for radio-opaque sialoliths that can be obscured by iodinated contrast. This can be followed by a routine contrast-enhanced neck CT in patients with clinical evidence of acute sialoadenitis. A good clinical history and communication between the referring clinician and radiologist is required to ensure that the protocol is tailored to answer the clinical question.
Neck, CT Angiogram
Helical multidetector CT angiography (CTA) has revolutionized vascular imaging in the past decade. Conventional angiography is still considered the “gold standard” for evaluation of vascular injury and vascular anatomy, but it has significant disadvantages including lengthy procedure, cost, and invasiveness with numerous risks including
dissection and stroke. Because of its accessibility, speed, and noninvasiveness, CTA is commonly used as a screening tool for vascular injury in patients with a history of blunt and penetrating neck trauma (8,9). CTA is also the primary imaging modality at many institutions prior to carotid endarterectomy. Conventional angiography is largely reserved for equivocal cases or cases requiring percutaneous intervention. CTA is best performed on a MDCT scanner (at least 16-slice or greater) using very thin cuts (e.g., 0.5-mm collimation) following the administration of iodinated contrast. Data are obtained from the thoracic aortic arch through the skull base. Multiplanar reformats are created
and ideally 3D volume rendered reconstructions should be created by the interpreting radiologist because essential data can be lost during the postprocessing (Fig. 11.10).
dissection and stroke. Because of its accessibility, speed, and noninvasiveness, CTA is commonly used as a screening tool for vascular injury in patients with a history of blunt and penetrating neck trauma (8,9). CTA is also the primary imaging modality at many institutions prior to carotid endarterectomy. Conventional angiography is largely reserved for equivocal cases or cases requiring percutaneous intervention. CTA is best performed on a MDCT scanner (at least 16-slice or greater) using very thin cuts (e.g., 0.5-mm collimation) following the administration of iodinated contrast. Data are obtained from the thoracic aortic arch through the skull base. Multiplanar reformats are created
and ideally 3D volume rendered reconstructions should be created by the interpreting radiologist because essential data can be lost during the postprocessing (Fig. 11.10).
MAGNETIC RESONANCE IMAGING
Imaging Principles
When a patient is placed in a strong magnetic field, the protons within the soft tissues of the body align with the field. To create an MR image, a radiofrequency pulse is applied that displaces the protons from alignment with the magnetic field. When the pulse is terminated, the displaced protons release a radiofrequency signal as they realign with the applied magnetic field. A receiving coil detects the signal emitted from the protons in the patient’s tissues and spatially localizes it using gradient magnetic fields. A computer then applies complex mathematical algorithms to reconstruct the radiosignals into a final image. The appearance of the soft tissue contrast can be manipulated by changing the pulse sequence parameters. Most clinical MRI units use a field strength of 1.5 tesla (T) or 3 T. Lower field strengths are more commonly employed in open bore magnets. The greater the field strength, the better the signal-to-noise ratio, which can result in better image quality and/or throughput, although artifacts may be greater on 3 T units.
Technical advantages of MRI include good soft tissue contrast, multiplanar imaging capabilities, and lack of ionizing radiation. Disadvantages include motion artifact, pulsation artifact (from physiologic vascular motion and swallowing), and metal artifact. The longer the pulse sequence or overall time of the study, the greater the motion artifact. Voluntary swallowing and heavy breathing can cause substantial motion artifact. This can be particularly problematic in dyspneic patients with laryngeal pathology or chronic obstructive pulmonary disease. Dental amalgam artifact varies with the type and extent of amalgam, the scanner strength, and the sequences employed. There are also numerous contraindications including cardiac pacemaker, cochlear implant, metallic orbital foreign body, and ferromagnetic aneurysm clips, to name a few. Manufacturer recommendations regarding MRI safety for implanted medical devices must be sought before placing a patient in the scanner. Careful use of gadolinium-based contrast in patients with renal insufficiency must be employed due to the small but increased risk of developing nephrogenic systemic fibrosis (a systemic disease characterized by progressive multiorgan fibrosis following exposure to gadolinium-based contrast agents) in this population (10,11). Gadolinium use is contraindicated in pregnant patients.
Technical Considerations
Judicious use of MRI is required because of the relatively greater cost, reduced availability, and longer scan times as compared to CT. Scan time particularly influences image quality and excessively long imaging protocols or inappropriate use of MRI as a screening modality frequently results in motion degraded images with loss of diagnostic information. It is often complimentary to CT in the evaluation of head and neck pathology and protocols are usually focused to answer a specific clinical question (12).
Useful applications of MRI include evaluation of skull base pathology, perineural spread of neoplasm, neoplastic marrow space involvement, neoplastic cartilage invasion in the larynx and evaluation of oral cavity pathology, particularly when compromised by dental amalgam artifact on CT. Furthermore, when ultrasound is not adequate, MRI is the preferred modality in pediatric imaging to minimize radiation exposure in this population.
Most basic MRI protocols of the neck and/or skull base include axial and coronal T1-weighted pre- and postcontrast images and T2-weighted sequences. T1-weighted images provide good anatomic detail and soft tissue contrast. On T1-weighted sequences, fat is high signal intensity, displayed as bright/white. Fluid, including normal cerebrospinal fluid (CSF) and vitreous, is low signal intensity, displayed as dark/black. On T2-weighted fast spin-echo (FSE) images, the most commonly employed T2-weighted sequence, CSF is displayed as bright/white signal and fat is also bright/white (Fig. 11.11). Muscle is intermediate on both T1- and T2-weighted sequences, displayed as gray. Contrast-enhanced T1-weighted images are used to delineate cystic from solid lesions.
Fat suppression techniques that null the bright/white fat signal are frequently applied to T2-weighted FSE and contrast enhanced T1-weighted sequences to improve tissue contrast of T2 hyperintense lesions and enhancing lesions surrounded by or distorting adjacent fat. Fat suppression techniques are also applied in orbital imaging where suppression of orbital fat signal increases conspicuity of orbital pathology, particularly evaluation of the optic nerves. The most common fat suppression techniques employed include short T1 inversion recovery (STIR) in which fat is placed at the null point of signal intensity and frequency selective presaturation spin-echo sequences in which fat signal is selectively suppressed (example sequences include T1-weighted postcontrast with fat suppression/fat saturation or T2-weighted with fat suppression/fat saturation) (Fig. 11.12).
Slice thickness varies from 3 to 5 mm. Focused thin section volumetric acquisitions can be employed to evaluate a specific area of interest such as the cavernous sinus or the facial nerve. The slice thickness is limited by the sequences employed. Unlike CT, imaging the entire neck from the skull base through the superior mediastinum typically requires two separate acquisitions. Additional sequences can be added to protocols in order to evaluate specific structures or answer-specific clinical questions as described in the following section.
Applications and Special Considerations by Region of Interest
Soft Tissue Neck, Aerodigestive Tract, and Larynx
For most pathology in the neck, CT is preferable to MRI because of its speed, accessibility, and lower cost. In the era of multidetector helical CT, the ability to create multiplanar reformats negates the traditional MRI advantage of multiplanar imaging capability. In many cases, the choice to use MRI versus CT in the evaluation
of neck pathology is user preference or convenience though there are some instances where MRI offers distinct advantages.
of neck pathology is user preference or convenience though there are some instances where MRI offers distinct advantages.
In patients with laryngeal squamous cell carcinoma, MRI offers better soft tissue contrast and is more sensitive for the detection of cartilage invasion (13) (Fig. 11.13). Thin-cut or volumetric imaging to improve staging accuracy should be employed (14). With a high negative predictive value in the range of 94% to 96%, MRI is useful to exclude cartilage invasion. Unfortunately, MRI features of neoplastic cartilage invasion overlap with peritumoral inflammation, resulting in a less than optimal positive predictive value. Comparing the signal characteristics of the cartilage with the primary tumor may be helpful in improving specificity (15).
Additional useful applications of MRI in the head and neck include the following: evaluating cranial nerve palsies, excluding prevertebral invasion in patients with extensive mucosal space neoplasm, characterizing oral tongue and hard palate lesions, assessing oral cavity lesions obscured by dental amalgam, predicting resectability of squamous cell carcinoma involving the carotid artery, assessing preepiglottic fat invasion in head and neck neoplasms, and evaluating perineural spread of neoplasm associated with oral cavity and oropharyngeal tumors (16). While MRI is sensitive for the detection of mandibular marrow space involvement in patients with oral cavity and oropharyngeal cancer, it lacks specificity due to false positives from dental disease and sequela of radiation therapy (16). Traditionally, CT was considered more sensitive than MRI for the detection of nodal metastasis (17,18). With advances in MRI imaging, the sensitivity of MRI for detecting abnormal lymph nodes may be comparable to CT but for practical purposes, CT is the preferred modality for evaluating lymph node pathology in the neck (19,20). MRI should be reserved for problem solving or targeted imaging.
Temporal Bone
Patients presenting with facial nerve symptoms and adults presenting with sensorineural hearing loss are initially best evaluated with MRI. It evaluates for pathology affecting the brainstem (ischemic or demyelinating disease), the 7th and 8th cranial nerve complexes and the membranous labyrinth. Facial neuritis, schwannoma, hemangioma, and neoplastic processes affecting the 7th and 8th cranial nerves, inner ear and cerebellopontine angle are well demonstrated with high-resolution MRI.
Neoplastic, inflammatory, and infectious processes occurring in the petrous temporal bone frequently have a soft tissue component that affects the osseous integrity of the bone. MRI and CT are complimentary in evaluating such lesions. MRI characterizes the soft tissue component of the lesion and helps to differentiate inflammatory processes (petrous apicitis) or sequela of inflammatory processes
(cholesterol granuloma and mucocele) from neoplastic processes (metastasis, eosinophilic granuloma, chondrosarcoma). It also delineates the intracranial extent of disease and its effect on the adjacent brain parenchyma. CT better demonstrates associated osseous abnormalities.
(cholesterol granuloma and mucocele) from neoplastic processes (metastasis, eosinophilic granuloma, chondrosarcoma). It also delineates the intracranial extent of disease and its effect on the adjacent brain parenchyma. CT better demonstrates associated osseous abnormalities.
Most temporal bone MRI protocols will include thin-cut precontrast T1-weighted images in the sagittal and axial planes to evaluate for high signal lesions and demonstrate anatomy. Contrast-enhanced T1-weighted images with fat suppression in the coronal and axial plane are used to look for enhancing lesions in the temporal bone or along the course of the 7th and 8th cranial nerve complexes. Various high-resolution T2 sequences (such as “CISS” or “FIESTA”) can be used to delineate the 7th and 8th cranial nerves in their canalicular and cisternal segments where they appear dark against the background bright signal of the CSF resulting in a “cisternogram” effect (Fig. 11.14) (21,22,23). Many of the other cranial nerves, most notably the 5th cranial nerve, can be imaged in a similar manner. This technique can be useful to evaluate for tiny vestibular schwannomas (particularly if contrast is contraindicated) or compression of the 7th nerve root entry zone which can contribute to hemifacial spasm. Diffusion-weighted imaging can be useful in evaluation of petrous apex lesions and in postoperative temporal bone evaluation. Both epidermoid cyst of the petrous apex (congenital cholesteatoma) and recurrent acquired cholesteatoma in the postoperative temporal bone demonstrate high signal intensity compared to brain tissue on diffusion-weighted images. Recent research suggests nonecho planar-based diffusion-weighted sequences with thinner sections and decreased susceptibility artifacts are the most useful for helping distinguish scar tissue and granulation tissue from recurrent cholesteatoma in the postoperative temporal bone (24,25). In patients with dehiscence of the tegmen tympani or tegmen mastoideum, thin-cut high-resolution coronal T2-weighted images and contrast-enhanced T1-weighted images are used to evaluate for meningocele or encephalocele.
Nasopharynx and Skull Base
Imaging plays a critical role in the evaluation of the skull base and nasopharynx because this area is centrally located and difficult to evaluate clinically. Skull base pathology can be categorized into processes originating above the skull base, below the skull base, or centered within the skull base. Consequently, it is important to tailor imaging protocols to include the relevant adjacent intracranial compartment and/or extracranial soft tissues. The soft tissue component of skull base pathology and potential routes of perineural spread of aggressive neoplasms are best imaged with MRI (26,27). CT better demonstrates osseous erosion and matrix associated with lesions, so both modalities are often employed (Fig. 11.15).
In order to optimize evaluation for perineural spread of tumor, protocols should use a small field of view focused on the area of interest using thin-cut 3-mm slices. Possible routes of direct or perineural spread of neoplasm in the orbits, hard palate, pterygopalatine fossa, skull base foramina, cavernous sinus, and temporal bone extending back to
the 5th and 7th nerve root entry zones should be imaged. Fat suppressed T1 postcontrast images are useful to directly image the nerves traversing the skull base to evaluate for excessive enhancement along nerve branches or widening within the pterygopalatine fossa, skull base foramina, Meckel’s cave or cavernous sinus, all of which can be seen with perineural spread of neoplasm (Fig. 11.16). Thin-cut T1-weighted images without contrast are essential to evaluate for loss of the normal fat surrounding nerves in the pterygopalatine fossa, within the infratemporal fossa and as they enter neural and skull base foramina as this can also be a sign of perineural spread of tumor. In Adults, Axial T1-weighted images are important to evaluate for loss of the normal fatty skull base marrow signal, which can be seen with neoplastic marrow infiltration, skull base osteomyelitis, blood dyscrasias, and other primary bone lesions (28).
the 5th and 7th nerve root entry zones should be imaged. Fat suppressed T1 postcontrast images are useful to directly image the nerves traversing the skull base to evaluate for excessive enhancement along nerve branches or widening within the pterygopalatine fossa, skull base foramina, Meckel’s cave or cavernous sinus, all of which can be seen with perineural spread of neoplasm (Fig. 11.16). Thin-cut T1-weighted images without contrast are essential to evaluate for loss of the normal fat surrounding nerves in the pterygopalatine fossa, within the infratemporal fossa and as they enter neural and skull base foramina as this can also be a sign of perineural spread of tumor. In Adults, Axial T1-weighted images are important to evaluate for loss of the normal fatty skull base marrow signal, which can be seen with neoplastic marrow infiltration, skull base osteomyelitis, blood dyscrasias, and other primary bone lesions (28).
Paranasal Sinuses
CT and MRI are complimentary in the evaluation of sinonasal neoplasms (29). The main utility of MRI of the paranasal sinuses and maxillofacial structures is to evaluate the extent of sinonasal neoplasms and associated perineural spread of neoplasm that will influence surgical approach and treatment planning. MRI delineates the margins of the
mass from adjacent obstructed secretions and inflammatory mucosal disease. It directly images skull base invasion, perineural spread of neoplasm, intraorbital involvement and intracranial extension of neoplasm, including dural and brain parenchyma invasion. Similarly, in cases of invasive fungal disease, MRI better characterizes the intracranial and perineural spread of disease. CT evaluates the osseous integrity of the orbits, anterior skull base, and maxillofacial structures (Fig. 11.16). A protocol similar to the described skull base protocol should be used with the field of view tailored to include the relevant portions of the craniofacial structures, orbits, sinonasal cavity, skull base, and mandible.
mass from adjacent obstructed secretions and inflammatory mucosal disease. It directly images skull base invasion, perineural spread of neoplasm, intraorbital involvement and intracranial extension of neoplasm, including dural and brain parenchyma invasion. Similarly, in cases of invasive fungal disease, MRI better characterizes the intracranial and perineural spread of disease. CT evaluates the osseous integrity of the orbits, anterior skull base, and maxillofacial structures (Fig. 11.16). A protocol similar to the described skull base protocol should be used with the field of view tailored to include the relevant portions of the craniofacial structures, orbits, sinonasal cavity, skull base, and mandible.
Salivary Glands
MRI is the study of choice for evaluation of a painless salivary mass, particularly if it is accompanied by a cranial nerve deficit (30). It offers good special resolution with superior soft tissue contrast compared to CT. In patients with a suspected inflammatory or infectious process involving the salivary glands, CT is the preferred imaging modality because sialoliths are difficult to see with MRI.
Imaging should extend to the skull base and posterior fossa to include the course of 5th and 7th cranial nerves to adequately assess for extent of perineural spread of neoplasm. In adults, parotid masses are well delineated on T1-weighted images because of the fatty background of the gland, which serves as a natural contrast agent. The margins of a parotid mass including extension into the adjacent parapharyngeal fat, masticator space, and bone marrow are well delineated on this sequence. Fat suppressed contrast-enhanced T1-weighted images are used to distinguish cystic from solid masses and evaluate for perineural spread and marrow space invasion. Fat suppressed T2-weighted images can be helpful in suggesting a lesion is malignant as most high grade malignant neoplasms will have low to intermediate signal on T2-weighted images and most benign parotid masses are T2 hyperintense (Fig. 11.17). There is, however, overlap between the signal characteristics of benign and malignant lesions. Low grade mucoepidermoid and adenoid cystic carcinomas can be T2 hyperintense while benign Warthin tumors can be T2 intermediate or low signal. Imaging does not serve as a substitute for soft tissue sampling.
Orbits
MRI is frequently the study of choice for many neuroophthalmic conditions. It offers better soft tissue evaluation of the optic pathways, cavernous sinus region, sella turcica, and brain parenchyma, all of which can be affected when patients present with visual or neuro-ophthalmic symptoms. All orbital protocols should include imaging in two orthogonal planes, typically the axial and coronal plane to demonstrate the relationship of the extraocular muscles with the optic nerve, osseous orbital margins, and orbital apex. T1-weighted precontrast images should be included to evaluate for T1 hyperintense choroidal lesions, which can be seen with melanoma. Utilizing fat suppression when imaging the orbits is critical on T2 and contrast-enhanced T1-weighted sequences to increase tissue contrast of orbital mass lesions surrounded by fat in the orbit and detect optic nerve and nerve sheath enhancement (Fig. 11.18).
Many orbit protocols will include an axial fluid attenuated inversion recovery sequence of the brain to evaluate for any evidence of demyelinating disease, which supports a diagnosis of multiple sclerosis in patients with optic neuritis. Protocols can be modified to include MR angiogram, MR venogram, or MRI of the brain, skull base, or neck depending on the patient’s clinical presentation (optic neuropathy, visual field deficit, cranial nerve deficit, optic disc swelling, Horner syndrome) and the suspected pathology (31).
Temporomandibular Joint
MRI is the preferred modality to evaluate for internal derangement in patients with temporomandibular joint (TMJ) dysfunction (32,33). Proton density or T1-weighted images may be used to demonstrate the margins of the disk relative to the joint capsule and bone (Fig. 11.19). Small field of view high-resolution imaging is employed. A typical protocol includes proton density coronal images and sagittal oblique images in closed and open mouth views. Open mouth images are used to evaluate disk function. Coronal images in the closed mouth view are used to look for medial or lateral displacement of the disc. T2-weighted images in the oblique sagittal closed mouth view are used to look for marrow edema and joint effusion.
Dynamic and pseudodynamic MRI of the TMJ has been the subject of research in an attempt to better characterize joint range of motion and to demonstrate pathology that may only be seen during mouth opening and closing (34,35,36). Image noise, suboptimal disc visualization, and temporal resolution remain barriers to the routine use of dynamic TMJ MRI at this time and the technique still requires validation. Currently, dynamic TMJ MRI may be used as an adjunct to standard open and closed mouth TMJ MRI but is not a replacement for it (34). CT is complimentary to MRI and provides good bone detail in cases of neoplastic involvement of the TMJ, posttraumatic deformity, and inflammatory arthropathy.
Brachial Plexus
Because it is inaccessible to direct palpation, clinical evaluation of the brachial plexus is challenging and imaging plays a critical role. MRI is well suited to evaluate the complex anatomy and pathology of the brachial plexus due to its multiplanar imaging capability and excellent soft tissue contrast. T1-weighted images demonstrate the anatomy best as the fat surrounding the brachial plexus acts as a natural contrast to delineate the roots, trunks, divisions, and cords. T2-weighted FSE images with fat suppression are used to identify pathologic signal changes in the plexus. Postcontrast images are useful when neoplasm, inflammation, infection, or stretch injury is suspected (37). The sagittal plane is particularly useful to evaluate
the relationship of the brachial plexus to the adjacent subclavian vessels and clavicle (Fig. 11.20). While unilateral high-resolution imaging in at least two or three planes is preferred, bilateral coronal STIR or axial T1 postcontrast fat suppressed images may be helpful to compare with the unaffected side, particularly if the reader is inexperienced (38).
the relationship of the brachial plexus to the adjacent subclavian vessels and clavicle (Fig. 11.20). While unilateral high-resolution imaging in at least two or three planes is preferred, bilateral coronal STIR or axial T1 postcontrast fat suppressed images may be helpful to compare with the unaffected side, particularly if the reader is inexperienced (38).
ULTRASOUND
Imaging Principles
Ultrasound employs a pulse echo technique to generate images. The transducer applied to the patient’s skin generates a high-frequency sound wave that is partially transmitted and partially reflected at each interface where there is a difference of acoustic impedance. The same transducer detects the reflected sound energy (echo) from these interfaces, with the depth of each echo estimated by assuming an average speed of sound and using the time of flight. The data are displayed as 2D or 3D gray scale images of the tissues. Using the Doppler frequency shift generated by moving red blood cells, Doppler ultrasound can image the vasculature in the neck (color Doppler sonography) or display the velocities and direction of flow in a vessel (spectral Doppler sonography).
Advantages of ultrasound include its lack of ionizing radiation, relatively low cost, portability, and real time and multiplanar imaging capabilities. At the energies used for medical imaging, there are no known harmful effects. Disadvantages include operator dependence, small field of view in some instances, and difficulty in mastering the anatomy as presented in nonstandard planes.
Indications and Technique
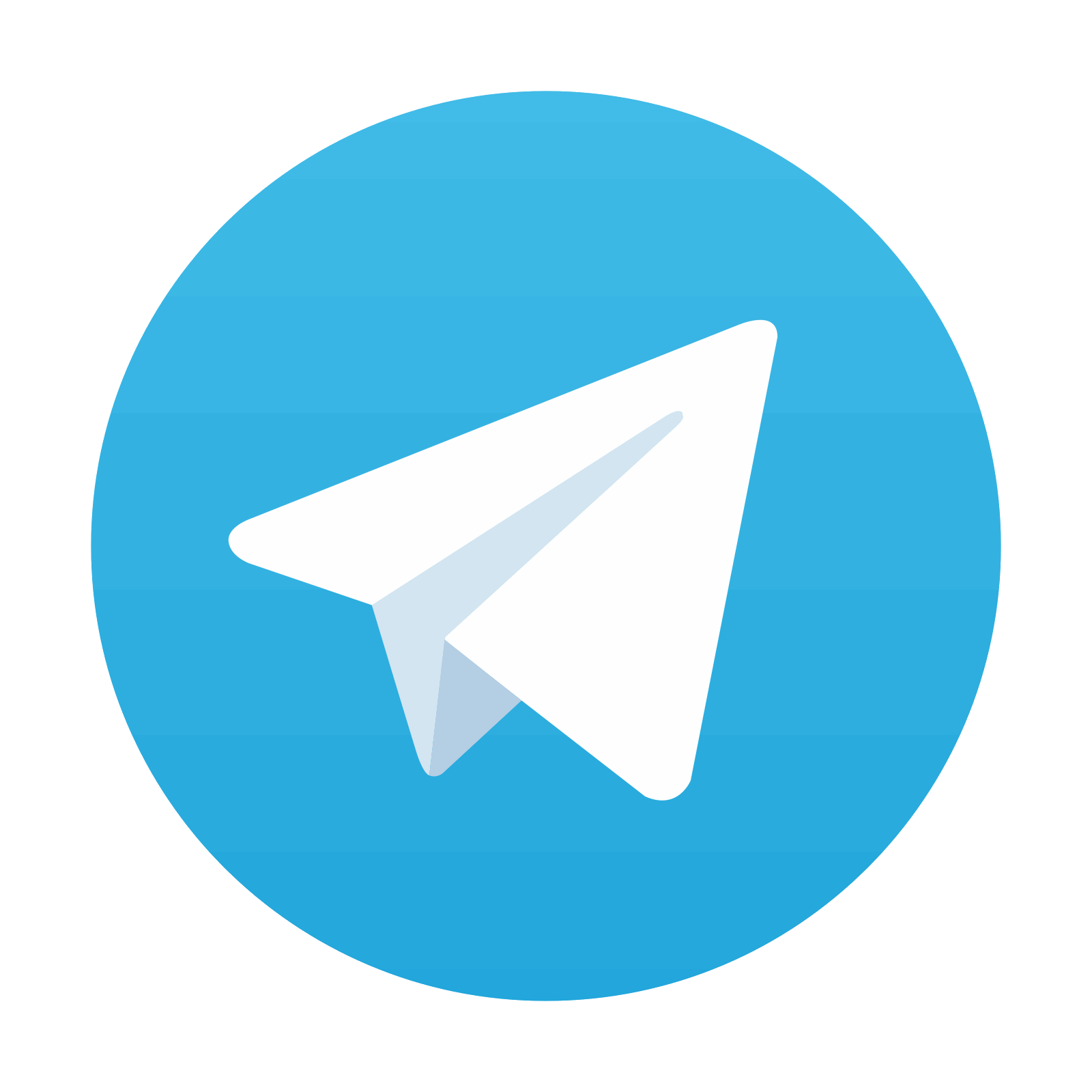
Stay updated, free articles. Join our Telegram channel

Full access? Get Clinical Tree
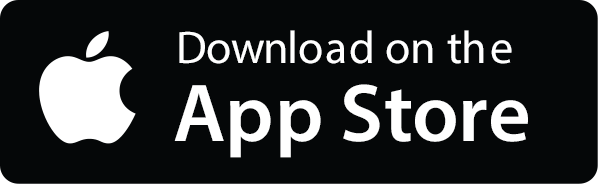
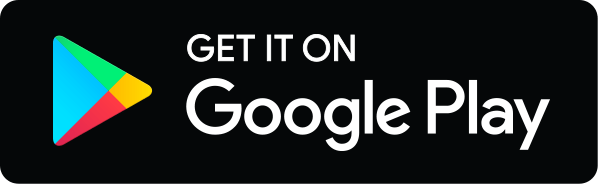