Fig. 19.1
Structure of inner ear sensory epitheria (modified from [1]). (a) Mammalian organ of Corti. (b) Avian basilar papilla. (c) Avian and mammalian vestibular epitheria
Another typical point of the structure for avian sensory epithelia is the weak cellular junction throughout the life, compared with the thick F-actin belts of the murine supporting cell from postnatal to adulthood [6, 7]. Generally adherens junction molecules associated with surrounding belts (E-cadherin, beta-catenin, or p120 catenin) translocate to the nucleus and alter transcription which regulate proliferation and differentiation in other epithelial cells [8–11]. It is therefore expectable in mammalian sensory epithelia that belt stiffness may restrict junctional molecules from transport to the nucleus, thereby limiting their ability to bind transcriptional elements that induce cell cycle entry and cell fate changes [12, 13].
19.2 Hair Cell Regeneration in the Avian Inner Ear
In contrast with mammals, mature birds can regenerate lost hair cell following sound exposure, aminoglycoside treatment, laser ablation, or genetic mutation [14–22]. Data from these groups strongly suggested that new hair cells were being produced in the damaged mature basilar papilla to replace those destroyed. In most cases, the hair cell loss induces non-sensory supporting cells to undergo either “proliferative” or “nonproliferative (direct transdifferentiation or self-repair)” mechanism for making new hair cells [21] (Fig. 19.2). On that time, the hair cell death and ejection from the sensory epithelium regulate the onset and progression of supporting cells producing hair cells [23–28], which was testified through the reduce of supporting cell mitosis after the rescues of damaged hair cells by apoptotic inhibitors [29].
In the first step of regenerative response, most of the supporting cells begin to reexpress developmental genes that are found in prosensory progenitors within a day after induced hair cell loss [26, 30, 31] (Fig. 19.2). It has been hypothesized that this process, which is also referred to as direct transdifferentiation, triggers a second response phase in which remaining supporting cells reenter the cell cycle and refill the supporting cells lost due to their transdifferentiation [32]. And in this second step, supporting cells are also able to respond to hair cell loss in parallel by asymmetric division, giving rise to pairs of replacement hair and supporting cells [33, 34]. The regenerative process in vitro is variable among culture conditions and degrees of ototoxic damage [35, 36]. Although it is still unclear which regenerative process is the dominating one, there is the strong evidence that both processes, “proliferative” and “nonproliferative,” happen in in vivo damaged avian basilar papilla, where supporting cell mitotic proliferation is activated not only to make new hair cells but also to replace the supporting cells lost for direct transdifferentiation [28, 31, 32, 37–40]. Compared to the basilar papilla, asymmetric supporting cell division occurred in both damaged and undamaged avian vestibular epithelium [26]. The cause of spontaneous hair cell death remains a mystery; however, turnover of undamaged vestibular hair cells is quite widespread among vertebrates which strongly hallmarks an existence of “stem cell” population in these tissues. Further study for the clarification of the causes of hair cell death with high turnover rates and characterization of “stem/stem cell-like cell” population in vestibular epithelium may provide clues into the regeneration in mammalian inner ear [41].
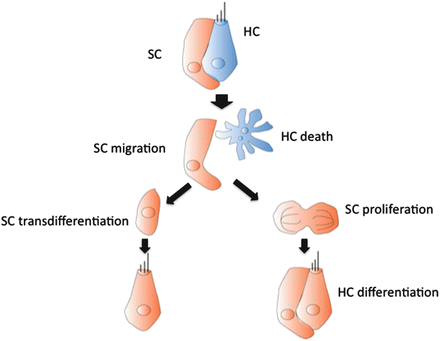
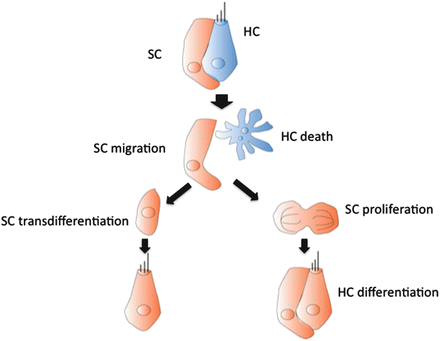
Fig. 19.2
Proposed mechanisms, proliferation and nonproliferation (transdifferentiation), of hair cell regeneration in the avian inner ear
19.3 Molecular Mechanisms of Avian Hair Cell Regeneration
Although the mechanisms of hair cell regeneration are not completely understood yet, some researchers’ approaches led to the identification of several molecules with the capacity to stimulate or block hair cell regeneration in avian sensory epithelium as follows.
It was found in the coculture experiments with chick damaged vestibular epithelium or normal ones that a soluble factor released from the damaged vestibular epithelium stimulated proliferation and factor from normal tissue suppressed mitotic activity [42]. From the subsequent experiments, this group suggests that robust regenerative proliferation of stem/stem cell-like cells in mature sensory epithelium requires a simultaneous release from negative regulation coupled with mitogenic signaling for epidermal growth factor (EGF) and/or insulin-like growth factor 1 (IGF-1)/insulin, but not with fibroblast growth factor 2 (FGF-2) [43–45]. Large-scale microarray analysis has performed for different damaging condition in chick sensory epithelium combined with the RNA interference (RNAi)-based method [30, 46]. Several distinct signaling pathways were identified at the regeneration stage by the use of the gene array, transforming growth factor beta (TGF-beta), Notch, Wnt, nuclear factor kappa-light-chain-enhancer of activated B cells (NF-kappaB), IGF-1/insulin, and activator protein 1 (AP-1). Small interfering RNA (siRNA) of Cutl1 resulted in inhibition of supporting cell proliferation [46], which molecule is the downstream targets of TGF-beta and suppresses cyclin-dependent kinase inhibitor p27Kip1. Cyclin-dependent kinase inhibitors control cell cycle entry, progression, and exit, so regulating cell cycle seems to be an important pathway to induce hair cell regeneration more directly [47, 48]. Other molecules including AP-1 (CCAAT enhancer binding protein (CEBPG), Jun-D, low-density lipoprotein receptor-related protein 5 (LRP5)), Pax (Pax2 and Pax5), and Wnt (Wnt4) were also suggested to regulate the supporting cell proliferation during chick hair cell regeneration. In addition to these secreted factors, cell–cell signaling mediated by Notch pathway or extracellular adherence proteins has been considerable. During hair cell regeneration Notch drives again lateral inhibition and the selection between hair cell and supporting cell fates [49–51]. As a consequence, the blockade of Notch signaling during regeneration results in the overproduction of hair cells at the expense of supporting cells [51, 52]. Notch1 and Jagged1 are expressed in the supporting cells of the adult basilar papilla [49, 51]. During regeneration Atoh1 is rapidly induced in the supporting cells (hours) [31, 52] and followed by Delta1 (days). Thereafter, hair cell differentiation markers are expressed and both Atoh1 and Delta1 downregulated [49]. Atoh1 is upregulated in the avian cochlear during hair cell regeneration [31, 51], suggesting that the regenerative mechanism in birds at least partially recapitulates aspects of embryonic hair cell development. Other pathways also have been implicated to trigger cell proliferative response in damaged and/or undamaged chick auditory epithelium and are mediated by cyclic AMP (and its regulated protein kinase A (PKA)), fibroblast growth factor receptor 3 (FGFR3), and Prox1 [53–55].
Because of the difficulty of gene manipulating for birds like transgenic chick, there is somewhat limitation to confirm the mechanism for regenerative response.
19.4 Functional Recovery of Hair Cell Regeneration
It is important to note that regeneration has been examined in several avian species (Gallus, Galliformes, Columbiformes, Passeriformes, and Psittaciformes) and appears to be essentially identical in all cases. Also for the function of regenerated avian ear, both hearing and vestibular sensitivities return to near-normal levels after sufficient recovery times [56]. Such findings confirm that afferent neurons can reestablish functional synaptic contacts with regenerated hair cells. Studies of vocalizations in songbirds also suggest that higher level auditory functions are also restored after hair cell regeneration [57, 58]. In addition, regenerated hair cells develop with morphologies that are consistent with their position along the proximal-to-distal axis [59]. And tuning curves, while broad during the initial period of regeneration, ultimately sharpen to degrees that are close to their pre-damage levels [60]. These results suggest that positional information like tonotopic axis could be supplied by hair cells located adjacent to the region of damage or by the supporting cells that remain. Ultimately, the regenerating sensory epithelium must produce enough new hair cells and supporting cells to structurally and functionally repopulate the damaged region.
References
1.
Ushakov K, Rudnicki A, Avraham KB. MicroRNAs in sensorineural diseases of the ear. Front Mol Neurosci. 2013;6:52.PubMedCentralPubMedCrossRef
2.
Brignull HR, Raible DW, Stone JS. Feathers and fins: non-mammalian models for hair cell regeneration. Brain Res. 2009;1277:12–23. doi:10.1016/j.brainres.2009.02.028.PubMedCentralPubMedCrossRef
3.
Lim DJ, Anniko M. Developmental morphology of the mouse inner ear. A scanning electron microscopic observation. Acta Otolaryngol Suppl. 1985;422:1–69.PubMed
4.
Driver EC, Kelley MW. Specification of cell fate in the mammalian cochlea. Birth Defects Res C Embryo Today. 2009;87:212–21. doi:10.1002/bdrc.20154. Review.PubMedCentralPubMedCrossRef
5.
Sinkkonen ST, Chai R, Jan TA, Hartman BH, Laske RD, Gahlen F, Sinkkonen W, Cheng AG, Oshima K, Heller S. Intrinsic regenerative potential of murine cochlear supporting cells. Sci Rep. 2011;1:26. doi:10.1038/srep00026.PubMedCentralPubMedCrossRef
6.
7.
Burns JC, Christophel JJ, Collado MS, Magnus C, Carfrae M, Corwin JT. Reinforcement of cell junctions correlates with the absence of hair cell regeneration in mammals and its occurrence in birds. J Comp Neurol. 2008;511:396–414. doi:10.1002/cne.21849.PubMedCentralPubMedCrossRef
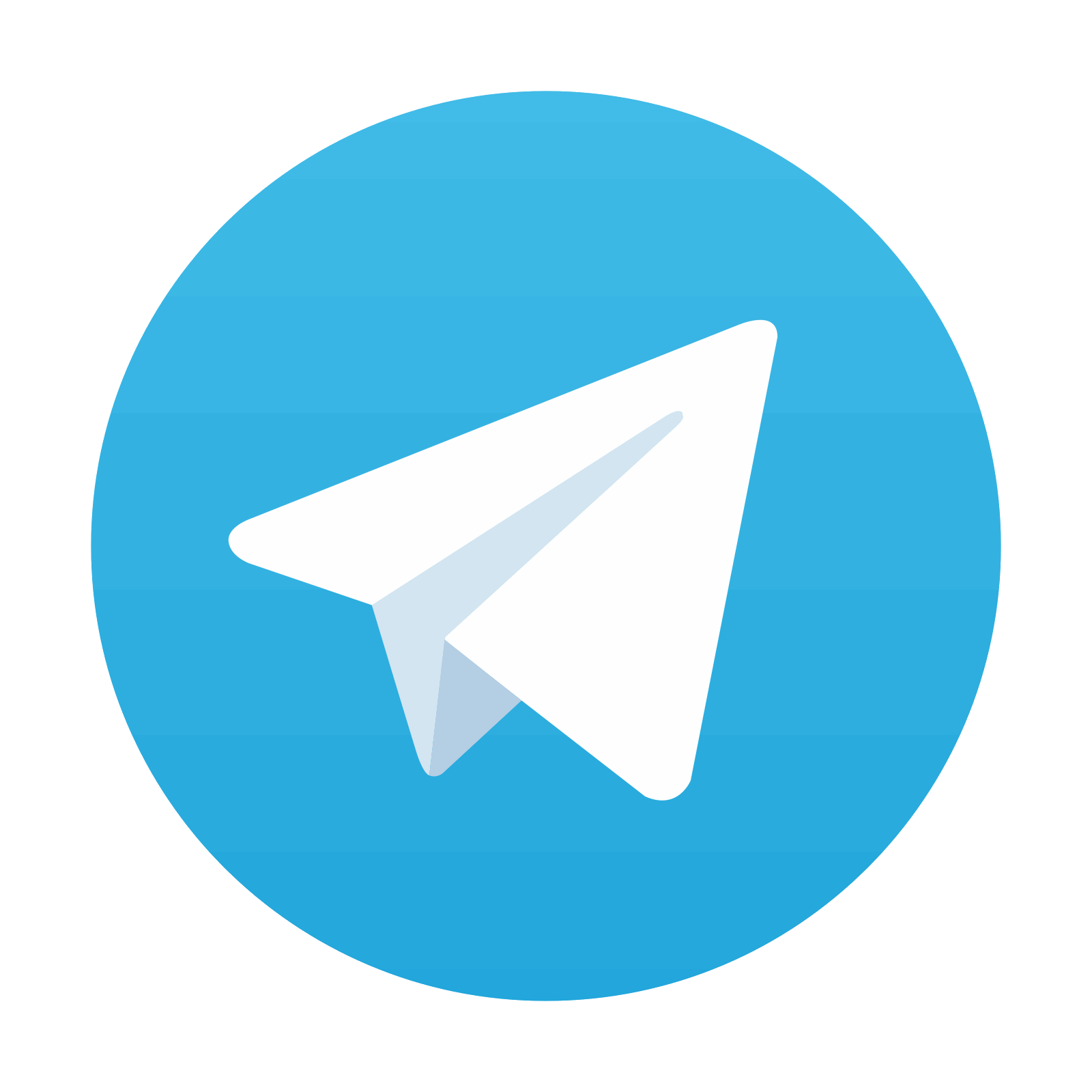
Stay updated, free articles. Join our Telegram channel

Full access? Get Clinical Tree
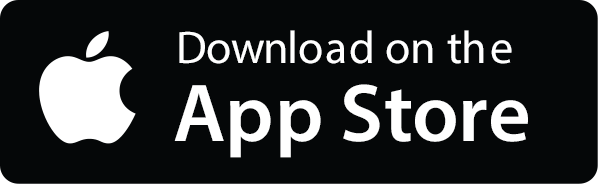
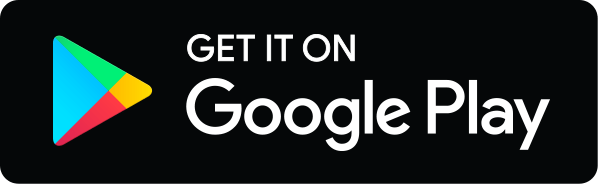