Gram-Positive Bacterial Keratitis
Mary Marquart
Richard O’Callaghan
Ocular infections are commonly caused by gram-positive bacteria. This is true for the eyelid, conjunctiva, cornea, and posterior portions of the eye. The organism most commonly isolated from infected eyes is Staphylococcus, but other gram-positive pathogens are also frequently encountered, including Streptococcus pneumoniae and Streptococcus viridans. Because of the overall importance of Staphylococcus, much of this chapter illustrates the host defense and pathogenic mechanisms related to this organism.
Classification
Based on recent classifications, 17 genera of gram-positive cocci are recognized, but only four of them are important corneal pathogens. The clinically important organisms include Staphylococcus aureus, Staphylococcus epidermidis, Streptococcus pneumoniae, and members of the viridans group of Streptococcus. Both classical bacteriologic staining and culturing methods as well as molecular techniques, such as the sequencing of the gene for the 16S RNA of ribosomes, commonly are used to identify and classify these bacteria.
Staphylococcus
Staphylococcus is a gram-positive coccus, about 1 μm in diameter, that divides in multiple planes to create grape-like clusters. The “grape-like clusters” predominate in cultures grown on solid medium, whereas in broth cultures, the organisms appear in short chains and in pairs as well as small clusters. Staphylococcus is a facultative anaerobe that produces mainly acetic acid under aerobic conditions and lactic acid under anaerobic conditions. More than 20 species are known, and all produce catalase, a distinction from the streptococci. Coagulase is a readily assayed product made by S. aureus, a major pathogen of man responsible for a great number of eye infections, and by S. intermedius, a pathogen mainly of animals (including canine keratitis). The numerous coagulase-negative species, other than S. epidermidis and S. saprophyticus, are of lesser importance relative to human infections. S. epidermidis is a major component of the human skin flora and a commonly encountered opportunistic pathogen. Relative to ocular infections, S. epidermidis is a cause of conjunctivitis, blepharitis, a major cause of keratitis, and the leading cause of postsurgical endophthalmitis. S. saprophyticus causes about 5% to 10% of cases of lower urinary tract infections.
Streptococcus
Streptococci are gram-positive cocci, 2 μm in diameter, that are catalase-negative and occur in chains or pairs. They are facultative anaerobes whose fermentation product is mainly lactic acid. The term “streptococci” includes at least three genera, Streptococcus, Enterococcus, and Abiotrophia.
Abiotrophia could be considered a nutritional variant of Streptococcus; it is a cause of rare cases of endocarditis. The genus Streptococcus includes over 50 species. of which S. pyogenes, S. agalactiae, and S. pneumoniae are the prime human pathogens.
Similar to the Streptococcus is the genus Enterococcus, which is also a major cause of human infections, including endocarditis, urinary tract infections, and wound infections. S. pneumoniae and some members of the viridans streptococci are important corneal pathogens.
Streptococcus species are initially identified in most cases by their hemolytic reactions on blood agar plates. The streptococci generally are divided into three categories: those that are β-hemolytic, those that are α-hemolytic, and those that are nonhemolytic. Many species of streptococci can be divided further into Lancefield groups, based on cell-wall–associated carbohydrate antigens specific to the particular species. The α-hemolytic species include S. pneumoniae and the multiple species of Streptococcus that are referred to as the strep viridans group or “green strep.” The term viridans refers to the color of the erythrocytes undergoing alpha-hemolysis. The viridans streptococci are further subdivided into groups such as the mutans group or the mitis group.
The β-hemolytic streptococci cause complete lysis of erythrocytes around bacterial colonies on sheep-blood agar. The β-hemolytic organisms include S. pyogenes, S. agalactiae, and S. equi, which are from Lancefield groups A, B, and C, respectively. Other β-hemolytic streptococci, mainly in group G, are infrequent causes of human infection. S. pyogenes is a major pathogen of humans, causing pharyngitis, impetigo, and wound infections. S. agalactiae is found in the vaginal tract and causes infections in neonates, especially meningitis.
The nonhemolytic streptococci were once called γ-hemolytic, a term that implies the lack of hemolysis. The term “γ-hemolytic” is confusing and should no longer be used. The nonhemolytic streptococci include the members of Lancefield group D streptococci. The group D organisms are Enterococcus (E. faecalis, E. faecium, and E. durans) and the Streptococcus species, S. equinus and S. bovis. These organisms share a group D antigen composed of a teichoic acid and a positive reaction on bile esculin agar medium due to the hydrolysis of esculin to a darkly colored product. Of these nonhemolytic organisms, Enterococcus is the most important pathogen of group D streptococci and is an important agent of endophthalmitis.
Structure and Function
The structure of the bacterial cell helps determine its resistance to host defenses and its stability in the environment. All bacteria have a circular genome surrounded by cytoplasm (no nuclear membrane) that is rich in ribosomes and carbohydrates for energy production. The cytoplasm is enclosed by a semipermeable cytoplasmic membrane composed of a lipid bilayer. The genomes of these gram-positive bacteria are capable of coding for up to 3,000 proteins. This double-stranded DNA molecule resides free in the cytoplasm. Bacterial genes whose products function in a related fashion (e.g., operate a particular metabolic pathway) are arranged into operons, the expression of which is controlled by a variety of factors. The synthesis of mRNA from an operon can be controlled by the availability of a specific molecule that is a product or target of the molecules produced by that operon. Such operon-specific regulation is important in expressing resistance to some antibiotics (e.g., erythromycin and clindamycin)1,2. Additionally, an operon can be controlled by regulatory molecules that respond to the overall metabolic state of the organism. Regulatory molecules include cyclic AMP, a molecule whose concentration indirectly varies with the amount of carbohydrates in the cell for energy production.3,4 When a bacterium is starved for its energy source, the cyclic AMP concentration increases, thus allowing the expression of multiple pathways as a means of employing a wider variety of molecules for energy. The broadest control of gene expression is quorum sensing or global regulation, a control system well illustrated in Staphylococcus.5,6 This broad regulation involves the synthesis of a small secreted peptide, the octapeptide. When the culture is in log phase (young), the octapeptide concentration is very low, and those genes involved in the production of cell-associated proteins are allowed to be expressed. When the octapeptide concentration increases as the culture approaches a stationary phase of growth (full quorum), the octapeptide reacts with a receptor in the bacterial cell membrane. This alters, in a global fashion, gene expression in the bacteria. In Staphylococcus, high concentrations of the octapeptide inhibit the expression of genes for cell-wall–associated proteins and allow the expression of genes for secreted proteins (e.g., toxins).
The predominant feature of gram-positive bacteria is the cell wall. The wall is composed of peptidoglycan, which includes numerous fibrous carbohydrate polymers of N-acetyl glucosamine and N-acetyl muramic acid linked together by short peptides. The wall is extremely rigid and provides the structural integrity of the cell. Without an intact cell wall, the cell cytoplasm in most environments would acquire water and swell to such a degree that the cell would lyse. Agents that directly damage the cell wall, such as lysozyme (or lysostaphin for Staphylococcus), directly cleave the peptidoglycan layer, allowing water pressure to lyse the bacterial cell. Cell-wall–specific antibiotics, such as penicillins, cephalosporins, or vancomycin, inhibit cell wall synthesis, allow bacterial autolytic enzymes to weaken the wall, and permit water pressure to lyse the bacterial cell. The peptidoglycan must be well constructed for the benefit of a proper reaction in a Gram stain. Aged bacterial cells often lose their gram-positive reactivity due to age-related changes in the peptidoglycan.
Carbohydrates on the bacterial surface can be responsible for part of the bacterium’s virulence. The peptidoglycan can be inflammatory, by activating complement via the alternate pathway. Teichoic acid is among the other carbohydrates found on gram-positive bacteria. Teichoic acids are linked to the cell wall or to the cell membrane and are composed of a glycerol phosphate or ribose phosphate backbone with side chains of repeating carbohydrates. Teichoic acids are sufficiently complex to be immunogenic as well as inflammatory via complement activation. Antibody to teichoic acid has been implicated in triggering harmful immune reactions in both the anterior and posterior portions of the eye.7,8,9 The capsule is another carbohydrate produced by some gram-positive bacteria; it is a structural layer that protects the bacterium from phagocytosis.10 Additionally, the ability to produce a biofilm is related to the production of large quantities of carbohydrate.11,12 However, not all biofilms are based on carbohydrates; protein-based biofilms do occur. The biofilm is composed of mushroom-shaped, three-dimensional structures that are designed to allow nutrient penetration around the mass of bacteria within the mushroom. Pieces of the biofilm containing bacteria can break away and “seed” other locations, thus allowing expansion and spread of the biofilm. A biofilm is a critically important structure for infections because bacteria in the biofilm resist removal by the flushing or cleansing actions of the body, are unavailable to the action of many host defense molecules and cells, and resist antibiotic action. Biofilm formation on a contact lens provides organisms a niche in proximity to the corneal surface.
The cell-wall–associated proteins are other important structures on a bacterium. These proteins have a variety of functions, but many are involved in adherence to the host. Proteins for binding to mammalian glycoproteins are common on bacteria. In Staphylococcus, proteins are found that bind to collagen, laminin, and fibronectin. Binding proteins are available in redundant forms; that is, several types of binding proteins are expressed, often with overlapping functions.13,14 The most unusual protein of S. aureus is the protein A molecule. This protein binds to the Fc region of certain subclasses of IgG immunoglobulins, thus preventing the Fc region from binding to phagocytic cells. Protein A also binds to the cell receptor for tumor necrosis factor α (TNFα), causing an inflammatory response.
Susceptibility to Antimicrobial Agents
The susceptibility of gram-positive ocular pathogens can be determined through a variety of laboratory tests.15 Those methods dependent on antibiotic discs (e.g., the Kirby-Bauer assay) provide a determination of sensitive, intermediate, or resistant. This method relates the zone of inhibition of bacterial growth around an antibiotic-containing disc to the likelihood of successful treatment of a systemic infection using that antibiotic. Other methods for determining antibiotic susceptibility provide a more quantitative determination that is expressed as the minimal inhibitory concentration (MIC); that is, the lowest concentration of the antibiotic that suppresses the growth of an inoculum (about 10,000 bacteria) to a high concentration (about 1 billion bacteria). The MIC is considered to be the amount of antibiotic needed in bodily fluids to inhibit the organism’s growth. The MIC has been traditionally measured by measuring the growth of bacteria in various dilutions of antibiotic; however, an important new alternative method involves the use of strips (called E strips) impregnated with a gradient of antibiotic that can produce a MIC determination directly from an agar plate growth of bacteria.
Other measurements of the interaction of antibiotic with bacteria are sometimes of importance. Occasionally, particularly in cases of endocarditis, the minimum bactericidal concentration (MBC) of an antibiotic is measured. The MBC is the amount of drug that kills a standard population of bacteria. Two other terms used occasionally in reference to antibiotic interaction with bacteria are the postantibiotic effect (PAE) and the mutant prevention concentration (MPC). The PAE refers to the time delay before growth resumes after a bacterial culture has been treated with a bacteriostatic antibiotic. The PAE occurs because the drug, while in adequate concentration, disrupts normal metabolic functions; after removal of the drug, however, the organisms need time to restore such functions before resuming growth. The MPC is the amount of antibiotic needed to prevent the growth of wild-type bacteria up to the concentration required to inhibit the growth of the least susceptible, single-step mutant. For the MPC determination, the antibiotic is challenged with a much higher number of bacteria than is used in the MIC determination (about one million-fold higher). The MPC is an important concept in preventing the growth of organisms that have some level of drug resistance prior to therapy. The concentration of antibiotic should be maintained above the MPC to avoid the selection of mutants that would give rise to a population of organisms that are not susceptible to the antibiotic.
One key point to consider is that the terms intermediately resistant and antibiotic resistant, as derived from standard laboratory assays, have direct application to nonocular infections, yet may not be accurate guides for ocular infections. This is true because topical antibiotic therapy can deliver more antibiotic to the site of an ocular infection than systemic therapy can deliver to infecting bacteria. If an infection is reported as containing an organism susceptible to an antibiotic by standard assays, then an ocular infection with that organism should be treatable with that drug. On the other hand, animal experiments have shown that if an organism is reported by standard assays to be resistant to a specific antibiotic, then repeated topical drops of that antibiotic might still be effective for the ocular infection caused by that organism.
The antibiotics of most importance for treating ocular infections are those effective for Staphylococcus, the most commonly encountered pathogen. This organism has been routinely susceptible to first-generation cephalosporins and to aminoglycosides. However, since the 1960s, hospital-acquired infections often have involved methicillin-resistant S. aureus (MRSA) strains. Unfortunately, since the late 1990s, MRSA infections also have become community-acquired problems16,17,18,19. MRSA strains, contrary to what their name implies, are resistant to multiple antibiotics. All MRSA strains contain a genetic element in each of their chromosomes that codes for a novel transpeptidase, an enzyme that achieves crosslinking reactions among those peptides that link cell wall carbohydrate polymers. This new enzyme of MRSA strains, unlike other transpeptidases, is resistant to binding by β-lactam antibiotics; thus, this one gene codes for resistance to the penicillins, cephalosporins, penems, and monobactam antibiotics. Flanking the gene for the transpeptidase are other genes that contribute to additional antibiotic resistances (e.g., aminoglycoside, macrolide, and tetracycline resistance). Hospital strains may remain susceptible to only vancomycin and/or to the new drugs developed specifically for highly resistant gram-positive bacteria (e.g., daptomycin, Synercid, and linezolid). MRSA strains isolated from community-acquired infections have somewhat fewer resistance traits and generally are susceptible to clindamycin, rifampin, and sulfa-trimethoprim antibiotics. MRSA strains were highly susceptible to fluoroquinolones as of the early 1990s; however, since that time, a marked increase in resistance to these drugs has occurred, such that, in some locations, nearly all MRSA strains are also fluoroquinolone resistant.20,21 This is somewhat in contrast to methicillin-sensitive S. aureus (MSSA) strains that retain more susceptibility to fluoroquinolones. The more advanced fluoroquinolones of the fourth generation (e.g., moxifloxacin or gatifloxacin) have been shown to be more effective for Staphylococcus than are the earlier generations of the fluoroquinolones (e.g., ciprofloxacin, ofloxacin or levofloxacin).22,23 Because resistance to one fluoroquinolone implies resistance to all fluoroquinolones, there has been consideration of using only the advanced drugs on a routine basis, to limit the selection of strains resistant to fluoroquinolones.
The treatment of serious Staphylococcus infections involving methicillin-sensitive strains can involve a cephalosporin plus an aminoglycoside (e.g., cefazolin plus gentamicin) or a fluoroquinolone, preferably a fourth-generation drug (e.g., moxifloxacin). The MRSA strains remain typically susceptible to vancomycin, and this remains an important therapy for treating Staphylococcus infections.
For Streptococcus pneumoniae or a viridans Streptococcus, a cephalosporin (e.g., cefazolin) is often preferred. Fluoroquinolones have been shown to effectively treat pneumococcus keratitis in the rabbit24 and human,25 but their effectiveness is decreasing.26 Gentamicin has been shown to be effective against penicillin-resistant S. pneumoniae in the rabbit eye,27 and carbapenems are effective against penicillin-sensitive and -resistant S. pneumoniae in vitro.28
Epidemiology and Predisposing Factors
Corneal infections caused by gram-positive cocci are a worldwide concern. Although risk factors are associated with ocular bacterial infections, any person can become afflicted with infectious keratitis. The most common risk factors that predispose otherwise healthy individuals to bacterial keratitis are contact lens wear,29,30,31 ocular trauma,32,33 dry eye,32,34 corticosteroid use,33,35 and ocular surgery (Fig. 49.1).36,37 Pre-existing conditions such as diabetes,32,35 HIV,34 and other ocular diseases, such as herpes simplex virus (HSV) keratitis,38 also can cause increased susceptibility to bacterial keratitis. Less common predisposing conditions include alcoholism,39,40 Sjögren syndrome,41 and Stevens-Johnson syndrome.41
As a risk factor, the use of contact lenses is the most prevalent and well-known. Contact lens wear has been associated with gram-negative bacterial keratitis, especially Pseudomonas, but recently an increase has occurred in the number of cases mediated by Staphylococcus. Some studies have suggested that extended-wear soft contact lenses cause the highest incidences of bacterial keratitis; however, daily-wear lenses and even rigid gas-permeable lenses have been associated with ocular infections.31 Contact lenses reduce the amount of oxygen reaching the cornea, especially when worn overnight or for extended periods.31 Contact lenses also can cause epithelial defects, thus compromising the integrity of the cornea.42 Concerns also have been raised that tear fluid movement between the lens and the corneal surface is reduced relative to fluid movement across the surface of a cornea not covered with a lens. Such restricted tear movement under the lens could reduce the concentration of host factors that protect the cornea. Furthermore, poor contact lens hygiene 43 and contact lens–induced acute red eye (CLARE) 44 can lead to corneal infections.
Throughout the world, S. aureus and S. pneumoniae are the most commonly encountered gram-positive cocci that cause keratitis (Table 49.1). S. epidermidis is another species commonly isolated from corneal ulcers. Occasionally, Streptococcus species other than pneumococcus, such as the viridans streptococci, are encountered.
TABLE 49.1. Gram-positive cocci as top causes of bacterial keratitis around the world. | ||||||||||||||||||||||||||||||||||||||||||
---|---|---|---|---|---|---|---|---|---|---|---|---|---|---|---|---|---|---|---|---|---|---|---|---|---|---|---|---|---|---|---|---|---|---|---|---|---|---|---|---|---|---|
|
S. aureus is by far the most frequently encountered gram-positive bacterium causing keratitis in the United States. This species has been identified as a commonly isolated ocular pathogen in epidemiologic studies conducted in Atlanta,62 Baltimore,63,64 Boston,65 Los Angeles,35 Miami,66,67 Pittsburgh,68 and San Francisco.69 The range of incidences of S. aureus keratitis in these studies was 13% to 27% of the total cases.
S. pneumoniae is usually the second most encountered gram-positive organism in the United States, having been identified as a prevalent keratitis pathogen in Boston,65 Miami,66,67 New York City,39 Philadelphia,70 and San Francisco.69 S. pneumoniae was identified as the causative organism in 7% to 12% of the keratitis cases in these studies. S. epidermidis has been identified as a significant pathogen in Baltimore,63,64 Miami,66,67 and Philadelphia.70 In fact, S. epidermidis was the most frequent cause of bacterial keratitis in the Baltimore and Philadelphia studies, causing between 28% and 47% of the total incidences of bacterial keratitis.
Human Keratitis
S. aureus, S. pneumoniae, and S. epidermidis are the most common gram-positive bacteria that cause bacterial keratitis. S. aureus is a normal inhabitant of the skin and, like S. pneumoniae, also resides in the upper respiratory tract. S. epidermidis normally is found on the skin and ocular surface. Like S. aureus, S. epidermidis can become an opportunistic pathogen of the cornea, but information about its pathogenesis is limited.
Corneal infection by S. aureus is a major cause of bacterial keratitis in children and adults.66,67,70,71 S. aureus corneal infections occur primarily in patients with defects in their ocular immunity and in contact lens wearers.71,72,73 S. aureus can cause infections when epithelial cells are compromised. Keratitis caused by Staphylococcus can result in corneal edema, rapid loss of the corneal epithelium, and the infiltration of neutrophils at the infection site and in the tear film. Those pathologic effects have been attributed to the toxicity of S. aureus α-toxin,74 a cytolytic exoprotein produced by about 75% of S. aureus strains.75,76,77,78
Strains of MRSA79,80,81 are particularly dangerous ocular pathogens in medically compromised patients.82 MRSA strains are multiply resistant to many antibiotics, and both MRSA and non-MRSA strains of S. aureus can be resistant to fluoroquinolones.68,82
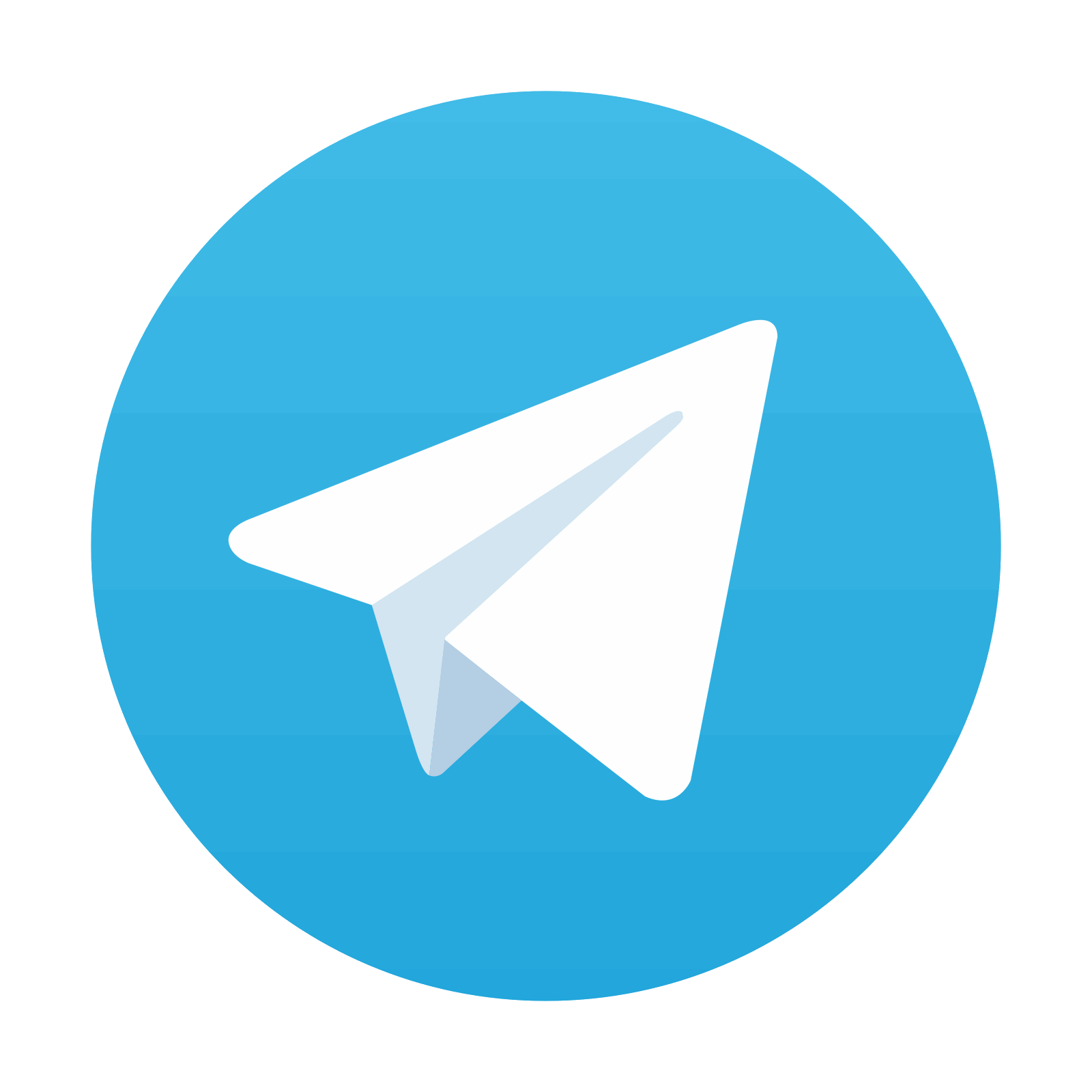
Stay updated, free articles. Join our Telegram channel

Full access? Get Clinical Tree
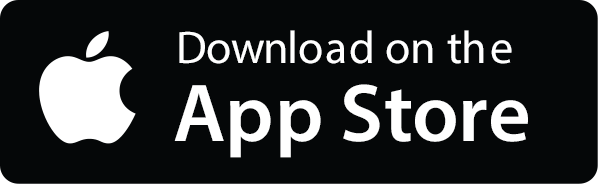
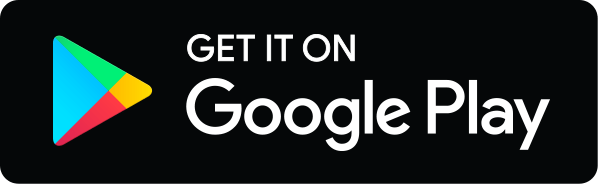