Viral gene
Viral life cycle
Transformation and oncogenesis
Immune modulation
E1
DNA helicase activity. Regulates viral gene transcription
E2
Regulates early gene promoter
Knockout due to integration of viral genome can cause deregulation of E6/E7 expression
E4
Control of viral release and genome replication
Involved in viral genome amplification
E5
Stimulates mitogenic signals of growth factors, including EGFR
Involved in inhibition of apoptosis as well as triggering differentiation
Interferes with MHC class 1 complex presentation
E6
Viral oncogene. Inactivates p53. Regulates many other cellular components
Significant. Prevents cell cycle arrest and apoptosis triggered by E7 activities
Inhibits cytokines including interferon
E7
Viral oncogene. Inactivates Rb. Regulates many other cellular components
Significant. Triggers reentry into the cell cycle and continued cell division
Inhibits cytokines including interferon
L1
Major capsid protein
HPV vaccine target
L2
Minor capsid protein
1.2.2 HPV Taxonomy
The L1 open reading frame is the most conserved region in the HPV genome and has, therefore, been used to define papillomavirus types. Distinct HPV types are characterized by greater than 10% dissimilarity to the closest known type within the L1 open reading frame (De Villiers et al. 2004). A great deal of sequence similarity also exists in the E1, E2, and L2 open reading frames, with somewhat less conservation of the E4, E5, E6, and E7 open reading frames (De Villiers et al. 2004).
HPV types are categorized by genus into five main groups: alpha, beta, gamma, mu, and nu (Fig. 1.1). Members of the same genus harbor greater than 60% nucleotide sequence identity within the L1 open reading frame and greater than 45% identity across the entire genome (De Villiers et al. 2004). Within each genus of HPV types are groupings referred to as species or clade. To be within the same species, HPV types must share between 60% and 70% of their nucleotide sequence (De Villiers et al. 2004). As mentioned above, HPV types are at least 10% dissimilar from one another, and additional subtypes may exist with nucleotide sequence dissimilarity of 2–5% (noted, e.g., as HPV6c and HPV6d) (Donne et al. 2010).


Fig. 1.1
Phylogeny of HPV types. HPV genuses are shown at the first division level. Most studied HPV subtypes exist within the alpha or beta genus. HPV types can either be mucosal or cutaneous, and mucosal subtypes are further subdivided into high and low risk based on potential for malignant transformation. Representative HPV types are shown below each subcategory, along with most common malignancies associated with those types
The division of HPV types by genetic similarity does not necessarily indicate functional similarity. For example, HPV-6 and HPV-11, which are the major contributing factors to RRP, share the same pathology as well as the same phylogenic subtype. In contrast, HPV-2 and HPV-4 are unrelated genetically, but both result in similar cutaneous papillomas (De Villiers et al. 2004).
In addition to the genetically determined phylogeny described above, HPV types are characterized by their predilection for infecting mucosal or cutaneous squamous epithelia. All HPV types infect stratified squamous epithelial tissues. Most of the symptomatic diseases result from types that colonize mucosal squamous epithelia, including high-risk HPV types that cause anogenital and oropharyngeal carcinomas as well as low-risk HPV types responsible for condylomata acuminata and RRP (Fernandes 2013). Alternatively, some HPV types such as HPV-2 and HPV-4 cause cutaneous lesions that infect the keratinized skin surface and are thought to be a risk factor for nonmelanoma skin cancer (Weissenborn et al. 2005). Most mucosal HPV types are of the alpha genus, and most cutaneous types are of the beta genus, although some overlap exists. In comparison to these types, relatively little is known regarding HPV types in the gamma, mu, and nu genera, from which most infections are asymptomatic (Tommasino 2014). These differences in disease states are possibly linked to variation in transmission and propagation of the virus, as well as differences in immune recognition and clearance (Doorbar et al. 2015). These significant divisions of HPV types, as well as the common malignancies seen with each division, are highlighted in Fig. 1.1.
1.3 Virus Life Cycle
HPV infects stratified squamous epithelial tissues, and its life cycle is closely tied to the biology of cellular differentiation within such tissues. The reasons for this particular tropism are not entirely known, although most evidence points toward tissue-specific transcription factors as opposed to cell surface receptors as the primary restrictive factor (Doorbar et al. 2015). Once the virus gains entry into epithelial cells, it embarks upon a well-characterized life cycle that is non-lytic, which in part explains its relative ubiquity and comparatively infrequent symptoms. Immune-mediated clearance and the function of putative viral oncogenes are what differentiate transient infections that go unnoticed from benign papillomas and metaplastic lesions that can progress to invasive carcinomas.
1.3.1 Viral Entry
The HPV virus initially infects cells within the basal layer of the stratified squamous epithelia at the site of infection (Moody and Laimins 2010; Groves and Coleman 2015). The infective viral particle resembles an icosahedral capsid, composed of the late region proteins, L1 and L2 (Doorbar et al. 2012). These proteins are able to interact with heparin sulfate proteoglycans, as well as possibly laminin or integrin α6 to facilitate cell entry (Giroglou et al. 2001). Micro-wounds within the epithelia allow viral entry and replication. As epithelial cells divide during wound healing, viral episomes gain entry to the nucleus (Groves and Coleman 2015). Low-risk HPV types are particularly dependent on the signaling produced by healing cells to facilitate viral episome maintenance, gene expression, and persistence of infection (Doorbar et al. 2012). Conversely, high-risk HPV subtypes, which can drive cell proliferation, may engage in alternative mechanisms for cell entry and persistence of infection (Doorbar et al. 2012; Doorbar et al. 2015).
1.3.2 Viral Maintenance and Genome Amplification
Upon nuclear entry, transcription of early genes is initiated. Most early genes (excluding E4) induce cell proliferation, leading to an increase of cells in the area that contain the viral genome (Fernandes 2013). At this time, there is very low expression of viral proteins and genetic material, allowing the virus to evade immune detection (Doorbar et al. 2015). The HPV genome does not encode polymerases or most of the other machinery necessary for DNA replication and relies solely on the host proteins for viral DNA synthesis. The lone exception is the E1 protein, which functions similarly to human DNA helicase (Moody and Laimins 2010; Satsuka et al. 2015).The viral E1 and E2 proteins bind at the origin of replication, which allows the recruitment of cellular polymerases and other cellular proteins that are required for replication (Moody and Laimins 2010; Kajitani et al. 2012).
Many transient viral infections may affect epithelial cells that are subsequently shed or cleared by the immune system. Persistent infection is thought to occur as a result of colonization of stem cells in the basal compartment of the epithelium (Doorbar et al. 2012). These cells by definition have the capacity for self-renewal and can give rise to differentiating cells within the stratified squamous epithelium. By infecting basal stem cells, HPV can persist in the long-lived stem cell compartment and also be passed on to differentiating daughter cells.
As the basal cells give rise to differentiating progeny within the stratified squamous epithelium, HPV episomes are maintained in both cell compartments. Viral genome replication is coupled to cell division; ensuring sufficient genetic material is passed on to the mother and daughter cells. Within differentiating daughter cells, expression of E1, E2, E6, and E7 is maintained, and expression of E4 increases. The E4 protein promotes a dramatic acceleration in viral genome replication (Fernandes 2013). Meanwhile, E6 and E7 act together to ensure that cells reenter S phase of the cell cycle. The daughter cells travel upward in the stratified epithelium throughout the amplification process, as shown in Fig. 1.2 (Fernandes 2013).


Fig. 1.2
Visualization of viral life cycle and amplification. The HPV virus initially infects cells in the basal layer, entering through micro-wounds. Wound healing promotes cell replication, and the virus is able to further induce cell division, resulting in the growth of the number of infected cells within the area. During this process, the viral genome is kept at a relatively low copy number to help evade immune detection. As the infected cells differentiate and reach the upper layers of the epithelia, viral gene replication is triggered and viral copy number significantly increases. Viral late gene expression leads to the production of functional virions that are shed with the keratinocytes, where they can infect new hosts
Upon reaching the superficial layers of the epithelium, the activity of the late promoter is stimulated (Doorbar 2005). This activation results in expression of viral capsid proteins (late proteins L1 and L2) as well as an upregulation of viral genes that are involved in DNA replication, without altering the activity of E6 and E7 that are required for maintaining the proliferative action of the host cell (Doorbar 2005). As a result, the viral genome copy number increases from 50–200 to several thousand copies per cell (Fernandes 2013; Doorbar et al. 2015). At the terminal stages of cell differentiation, E2 mediates the downregulation of E6 and E7, and the cell exits the cell cycle upon terminal differentiation (Fernandes 2013).
1.3.2.1 Specific Activities of E6 and E7
The E6 and E7 proteins are expressed from a single mRNA transcript. Expression of E6 and E7 is under the control of E2, which represses transcription upon binding to the LCR (Bernard et al. 1989). The primary influence of E6 and E7 proteins on the viral life cycle stems from inhibition of the G1/S cell cycle checkpoint. This causes the transition into S phase in cells that would otherwise not be dividing (Doorbar et al. 2012), which allows the viral genome to replicate, amplify, and eventually be packaged and released. Thus, cell proliferation is a secondary effect that results from the release of the G1/S checkpoint in infected cells.
The E6 and E7 proteins interact primarily with p53 and Rb, respectively. Key downstream cellular processes affected by the E6 and E7 proteins are highlighted in Fig. 1.3. The phenotypic differences seen between the high- and low-risk types relate to the efficiency by which binding and inactivation occur. Key differences in the actions of high- and low-risk protein effects are outlined below.


Fig. 1.3
Significant cell signaling pathways of HPV E6 and E7 proteins . E6 and E7 proteins inhibit tumor suppressor proteins p53 and pRb, respectively. The E6 protein associated with the E6-associated protein (E6AP) degrades p53 via an E3 ubiquitin ligase in high-risk HPV types. This association is seen in low-risk types, but does not lead to the degradation of p53; instead a loss of function is seen. The lack of p53 activity results in a loss of the G1/S cell cycle checkpoint arrest. Via a PDZ domain, E6 is able to inhibit DLG and associated proteins, preventing the complex from exerting its function: inhibiting viral growth rates and eliminating viral episomes. E6 can interact with other proteins to enhance cell proliferation and prevent cell senescence. The E7 protein inhibits Rb, which under normal cellular conditions prevents the cell from reentering the cell cycle via the G1 to S phase transition. With Rb activation, E2F is inhibited, preventing cyclin A/E and CDK2 activation. E7 has also been shown to inhibit other proteins that ultimately result in CDK2 deactivation. Finally, E7 binds HDAC proteins, which contributes to unrestrained cell growth through aberrant chromatin remodeling
The E7 protein is structurally similar to the adenovirus E1A protein and shares its 3-domain structure. It was this similarity that leads to the efficient determination that the retinoblastoma (Rb) tumor suppressor was the target of the E7 protein (Dyson et al. 1989; Klingelhutz and Roman 2012). The second conserved domain of E7 (as well as E1A) contains an LXCXE motif, which interacts directly with Rb and its two related proteins, p107 and p130 (Oh et al. 2004). Rb is a critical gene in regulating the cell cycle. Rb negatively regulates the E2F family proteins, resulting in their inactivation during Go and G1 phases of the cell cycle. Under normal conditions, the E2F transcription factors are the regulators of the G1 exit and progression to S phase (McLaughlin-Drubin and Munger 2009). Upon activation of E2F family proteins, transcription of cyclin A and E occurs, leading to expression of CDK2 and entry into S phase (Tommasino 2014) (Fig. 1.3). High-risk E7 protein binds to Rb with tenfold greater affinity (White et al. 1994). E7 binding to Rb leads to its inhibition in low-risk HPV types and proteasomal degradation in high-risk types (Klingelhutz and Roman 2012). E7 has also been shown to bind directly to the CDK inhibitors, p21WAFI, CIPI, and p27KIPI, neutralizing their cell cycle inhibitory effects (Moody and Laimins 2010; Tommasino 2014). Finally, the E7 protein alters chromatin structure within host cells as a result of Rb inhibition. Specifically, E7 counteracts Rb-dependent histone deacetylase (HDAC) regulation of E2F-regulated genes. Moreover, Rb-independent interaction of E7 with HDAC has been shown to promote cell growth (Brehm et al. 1999).
The deregulation of the cell cycle induced by E7 function causes levels of the G1/S cell cycle checkpoint protein, p53, to increase which leads to cell cycle arrest (Moody and Laimins 2010). Both high- and low-risk HPV E6 proteins inactivate p53 function to relieve this cell cycle arrest, but only high-risk E6 is able to incite the ubiquitination and degradation of the protein (Klingelhutz and Roman 2012). Specifically, high-risk E6 proteins interact with E6-associated protein (E6AP), forming a complex that binds to the DNA binding domain of p53 and leading to its degradation via E3 ubiquitin ligase (Fig. 1.3) (Scheffner et al. 1993). While low-risk E6 proteins can also form a complex with E6AP, they do not incite ubiquitination, suggesting that alternate mechanisms of p53 inhibition are more relevant, and ubiquitination targets of the E6 and E6AP complex are unclear (Brimer et al. 2007). The E6 protein itself can also bind to p53 to inhibit function in both high- and low-risk types (Lechner and Laimins 1994), and this is believed to be the main mechanism by which low-risk HPV types inhibit p53 activity (Moody and Laimins 2010).
E6 can also inhibit the activities of p300 and CREB-binding protein (CBP) , which are transcriptional co-activators involved in many pathways relevant to cellular differentiation and proliferation (Patel et al. 1999; Moody and Laimins 2010). One of the downstream transcriptional targets of p300/CBP signaling is p53 itself. E6 proteins from both high- and low-risk HPV types bind to p300/CBP; however the inhibition by the low-risk HPV6 E6 was only 50% as efficient as that by the high-risk HPV16 E6 (Patel et al. 1999).
An additional mechanism by which high-risk HPV E6 proteins promote cell cycle continuation and progression is through inhibition and degradation of the tumor suppressor, DLG. High-risk HPV E6 proteins harbor a PDZ binding motif (PDM) located at the C terminus of the protein (Doorbar et al. 2015), which facilitates binding to the PDZ domain within DLG. This binding results in the degradation of DLG and an augmentation in cellular growth rate (Pim et al. 2000; Pim and Banks 2010). Blocking the interaction between HPV16 E6 and DLG was shown to reduce the cellular growth rate and lead to a loss of viral episomes (Brimer et al. 2007; Nicolaides et al. 2011). While low-risk HPV E6 proteins are also critical for maintenance of viral episomes (Oh et al. 2004), unlike high-risk HPV E6 proteins, they are apparently unable to induce the degradation of DLG (Brimer et al. 2007).
The E6 protein has also been shown to interact with telomerase-related enzymes, leading to an increase in TERT transcription. This allows indefinite proliferation and the maintenance of cellular immortality, although this is only seen in high-risk HPV types (Klingelhutz and Roman 2012; Tommasino 2014). There are many additional known targets of the E6 protein; however their exact cellular functions are unknown (Tommasino 2014).
1.3.3 Virus Synthesis and Release
When the basal daughter cell approaches the surface of the stratified epithelium, it reaches terminal differentiation, and encapsulation of the viral genome is triggered (Kajitani et al. 2012; Doorbar et al. 2015). Alternative RNA splicing facilitates the turnover from early to late gene translation (Kajitani et al. 2012). The capsid proteins, L1 and L2, are expressed and transported into the nucleus where capsid assembly takes place. L2 is guided into the nucleus through its association with host protein, DAXX. PML bodies attract high levels of L2 protein and act as a scaffold to facilitate viral capsid assembly. L1 is then recruited to these sites. L1 is sufficient for viral capsid assembly, but this process is more efficient in the presence of L2 (Doorbar 2005). On the surface layer, dying keratinocytes undergo a change lose their mitochondrial oxidative phosphorylation capability, decreasing the overall pH of the viral environment. As a result, disulfide bonds form between L1 proteins, which stabilizes the capsid structure (Buck et al. 2005; Doorbar et al. 2015). Virus maturation occurs as keratinocytes become cornified cells that are shed along with mature packaged virions (Bryan and Brown 2001). Encapsulated virions are then released from the shedding cells into the environment, where they can survive for greater than 1 week prior to reinfection into a new host (Roden et al. 1997).
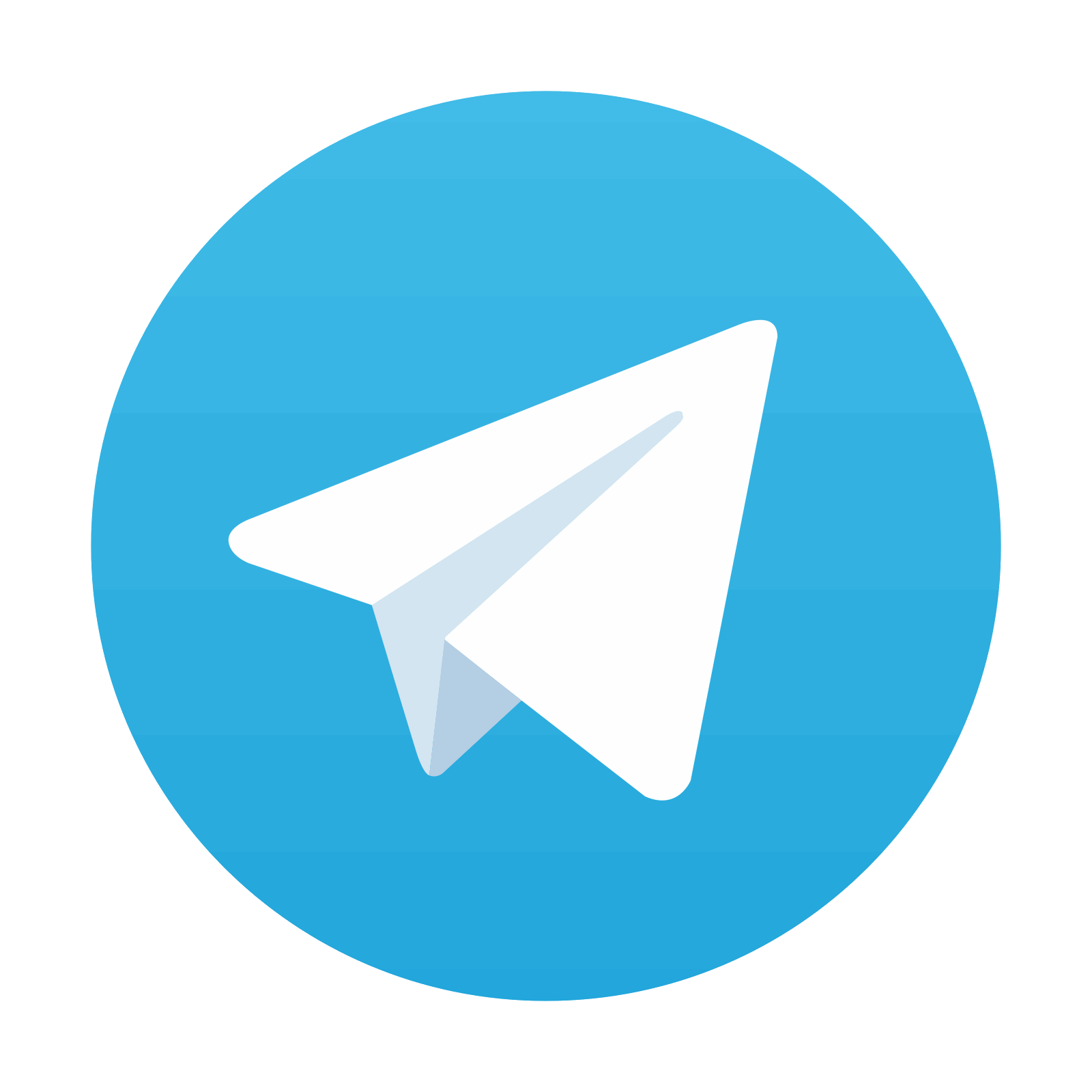
Stay updated, free articles. Join our Telegram channel

Full access? Get Clinical Tree
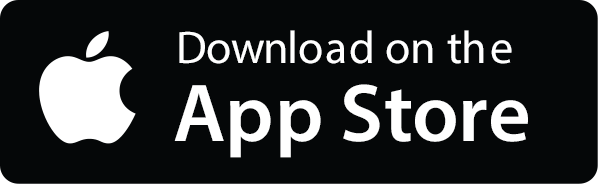
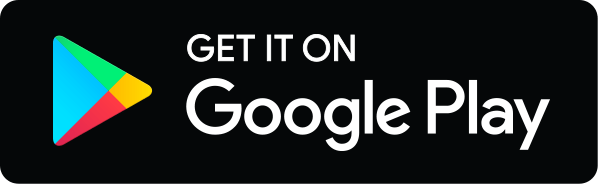