Gardasil
Gardasil 9
Cervarix
Gender
Females and males in the USA
Females and males in the USA
Females
Age (years)
9–26
Females 9–26 and males 9–21
9–25
Recommended age (years) at vaccination
11–12 in the USA
11–12 in the USA
11–12 in the USA
HPV types covered
HPV 6, 11, 16, and 18
HPV 6, 11, 16, 18, 31, 33, 45, 52, and 58
HPV 16 and 18
Dosing regimen
3 over 6-month period
3 over 6-month period
3 over 6-month period
The current L1 vaccines are designed to be given in a series of three injections over a 6-month period. Many countries, however, are studying two-dose regimens, rather than three doses, to reduce cost and improve compliance. In such a study of Cervarix, women that received two doses of Cervarix had as much protection from HPV 16/18 as women that received three doses, over a 4-year study period (Kreimer et al. 2011). Other studies have also shown that the protective efficacy of two doses of Gardasil (Dobson et al. 2013) or Cervarix (Romanowski et al. 2011) was comparable to that of three doses in young adolescents.
The number of doses recommended and vaccination requirements (gender, target age, etc.) vary around the world. Although the World Health Organization recommends two doses of Gardasil, a three-dose regimen is still recommended in the USA. The specifics of HPV vaccination protocols also vary state by state in the USA (State Vaccination Requirements 2016). For example, the District of Columbia and Virginia require HPV vaccination for girls entering sixth grade, but parents may opt out. Rhode Island requires all boys and girls entering seventh grade to be vaccinated against HPV infection. School-based immunization programs are in effect in many countries including Canada, Malaysia, Indonesia, and several European countries.
4.3.3 Efficacy
During FDA trials of Gardasil and Cervarix, both vaccines were shown to be effective in preventing almost 100% of cervical infections with HPV 16 and 18. Gardasil 9 is about 97% effective in preventing cervical, vulvar, and vaginal disease caused by the five additional HPV types (Chatterjee 2014). All three L1 vaccines protect against HPV 16 and 18 infections, which cause about 70% of cervical cancers and 86–95% of HPV-related non-cervical cancers (Gillison et al. 2008). Gardasil also protects against HPV 6/11 infections – the subtypes responsible for about 90% of genital warts (Koutsky et al. 2002) as well as RRP.
The efficacy of L1 vaccines has been studied in multiple countries, and studies of long-term efficacy are still ongoing. In Denmark, vaccination was associated with a 45% reduction in genital warts in girls 16–17 years of age (Ferris et al. 2014). In the aforementioned Australian HPV vaccination program, a high proportion of girls are vaccinated with Gardasil. As a result, the incidence of genital warts fell in vaccinated females and even in unvaccinated males (Fairley et al. 2009). This is an example of herd immunity, where genital wart cases decreased in heterosexual men after the introduction of HPV vaccination in women.
HPV vaccination in males has also been well studied, not only because they are at risk of HPV infections, but because sexual behavior and HPV infection significantly affect their female or male sexual partners. A HPV study of men conducted in Brazil, Mexico, and the USA found that the overall HPV infection prevalence was 65.2% in this male study population (Giuliano et al. 2008a). One study on HPV in males showed a reduced risk of cervical cancer in women whose male partners had multiple sexual partners and were circumcised compared to women whose male partners were uncircumcised (Castellsague et al. 2002). This difference was due to an almost four-fold lower rate of HPV infection in the circumcised group of men. HPV prevention in both men and women will reduce the probability of viral transmission and will thus reduce the risk of HPV-related diseases. Studies of HPV vaccination in the male population demonstrate protective effects against HPV-associated diseases of genital warts (Giuliano et al. 2011) and anal intraepithelial neoplasia (Palefsky et al. 2011), even though males seem to have a lower immune response to HPV infections (Giuliano et al. 2008b; Dunne et al. 2006) compared to women.
As of yet, there is no conclusive data about the minimum antibody levels necessary for HPV VLP protection against infection or about the role of memory B cells if antibody levels decrease. It is encouraging that in animal models vaccinated with commercial L1 vaccines, very low concentrations of antibody have been found to be protective (Day et al. 2010). In humans, long-term data have shown that Gardasil protects at least 8 years (Lowy and Schiller 2012) and Cervarix at least 9 years (Centers for Disease Control and Prevention 2012). A study in Denmark, Iceland, Norway, and Sweden is evaluating the long-term effectiveness and safety of the HPV vaccine in young women (Nygård et al. 2013). The long-term efficacy for the newer Gardasil 9 is not yet known.
4.3.4 Limitations
These highly effective commercially available prophylactic HPV L1 vaccines are very expensive. Gardasil and Cervarix each cost more than $100 per dose, and these vaccines require refrigeration. The VLPs for both Gardasil and Cervarix are complexed with aluminum salts and need to be refrigerated in a liquid state, making transportation difficult and costly. Although many insurance plans and programs now cover HPV vaccination in the USA, distribution and implementation in developing countries is very difficult.
Vaccine acceptance and compliance are hampered by high cost, the need for multiple injections to complete the full immunization course, and the sporadic and sometimes limited access of most adolescents to health care. In the USA, the vaccination rate for girls aged 13–17 years for at least one dose was 54% and for the completion of all three doses was only 33% ((CDC) CfDCaP 2013). School-based programs in the UK, Australia, and some parts of Europe have ≥80% vaccination rates. Many countries are therefore trying to implement a two-dose protocol. The European Medicines Agency approved a two-dose Cervarix schedule for girls aged 9–14 years, but three doses are recommended for girls older than 14 years.
HPV L1 vaccines are ineffective at protecting against non-vaccine HPV types. The neutralizing epitopes in the L1 protein are not cross-reactive among subtypes. There is also no therapeutic activity of vaccines against established HPV infection (Hildesheim et al. 2007; Schiller et al. 2012). The HPV-infected basal epithelial cells do not express detectable levels of L1 and/or L2 and therefore cannot be targeted by the immune response from these vaccines (Schiller et al. 2008).
Adverse reactions to Gardasil and Cervarix are unusual. Pain or swelling at the injection site is a common side effect. Other reactions include fatigue, fever, gastrointestinal symptoms, headaches, and anaphylaxis (Bayas et al. 2008). The National Vaccine Injury Compensation Program provides compensation for people that require medical care as a result of vaccination.
The presumed sexual implications of administration of HPV vaccines to teens and preteens have caused some parents and a number of religious and conservative groups to oppose routine HPV immunization. A study on college-aged women reported that HPV vaccination did not affect their decisions on condom use or other birth control (Ports et al. 2014). Nevertheless, vaccine critics continue to report public concerns regarding about encouragement of risky sexual practices by such vaccination (Constantine and Jerman 2007; Marlow et al. 2009).
4.3.5 Future Directions
The search for a more cost-effective, stable vaccine that provides broader protection against all oncogenic HPV types is ongoing. The current L1 vaccines are VLP based and require 360 copies of the L1 protein, which is one of the costly steps in HPV vaccine production. A capsomere-based vaccine may be a more cost-effective alternative, with efficacy similar to the VLP-based vaccines. Only five copies of the L1 protein are required, and the vaccine can be produced in bacteria, with reduced cost (Fraillery et al. 2007).
4.4 L2 Vaccines
4.4.1 Biology
The type-specific nature of L1 vaccines makes it economically and biologically unfeasible to keep adding more and more subtypes to prophylactic L1 vaccines. A pan-HPV type vaccine would therefore be ideal, a concept that has led to research into L2 vaccines. L2 is a minor papillomavirus capsid protein that is required for infection and is highly conserved among HPV types. Unlike L1, the major determinants of L2 that neutralizing antibodies can recognize are not exposed when virions are free in solution. However, they are exposed after the virion binds to the basement membrane, where furin cleavage of the N-terminus of L2 occurs. The N-terminal region is highly conserved among HPV types and can induce protective immunity in animal papillomavirus models.
4.4.2 Indications/Implementation
Studies have shown that there are conserved protective epitopes between residues of L2, suggesting that L2 can provide broad protection against many HPV subtypes (Wu et al. 2015). Part of the L2 protein, between amino acids 20 and 38, is conserved in many high-risk HPV subtypes. Broad-spectrum neutralizing antibodies are induced, and similar to HPV L1 vaccines, L2 vaccines could be protective against potential infection. Therefore, the target populations for L2 vaccines are the same as for L1 vaccines, children and adolescents prior to HPV exposure that can occur during sexual activity.
4.4.3 Efficacy
Although there are no commercially available HPV L2 vaccines and human L2 vaccine trials have not been conducted, animal studies have demonstrated protective immunity of L2 against HPV infection (Karanam et al. 2009a; Schellenbacher et al. 2013; Jagu et al. 2013). In a mouse model, the oral administration of L2 displayed on L. casei induced systemic and mucosal cross-neutralizing effects (Yoon et al. 2012). In a cervicovaginal mouse model, L2 showed in vivo neutralization (Roberts et al. 2007). Mice vaccinated with the L2 vaccine synthesized with L. casei induced neutralizing antibodies against multiple oncogenic HPV types, including 16, 18, 45, and 58. The ability to mass-produce L2-based vaccines in bacteria would reduce production costs (Karanam et al. 2009b).
4.4.4 Limitations
Unfortunately, L2 is weakly immunogenic compared to L1 VLPs (Roden et al. 2000). L2-induced titers are at least ten times lower than the titers induced by L1 (Pastrana et al. 2005). The weak immunogenicity of L2 can be overcome by linking together short amino acid sequences of L2 from different oncogenic HPV types or displaying L2 peptides on a more immunogenic carrier (Jagu et al. 2009; Tumban et al. 2012). In addition, adenovirus types can be used as platforms for capsid display of foreign antigens in order to induce protective immunity (Fraillery et al. 2007; Wang and Roden 2013; Sharma et al. 2013; Farrow et al. 2014).
4.4.5 Future Directions
Several groups are researching various L2-based vaccines, often in conjunction with therapeutic vaccines that will treat established disease and while the L2 component will prevent new infections. TA-CIN/GPI-0100 and pNGVL4a-hCRTE6E7L2 DNA vaccine are some of the current L2 vaccines that show promise in protection against cervical cancer. These studies are being conducted in both animals and humans. A study of the pNGVL4a-hCRTE6E7L2 DNA vaccine in mice demonstrated a strong E6- and E7-specific CD8+ T-cell response after vaccination with electroporation as well as eliciting a strong L2 response that would prevent against pan-HPV infections (Peng et al. 2014).
4.5 Therapeutic Vaccine Technology
4.5.1 Biology
One of the major limitations of L1 and L2 vaccines is that they lack a therapeutic role; that is, they will not treat established HPV-related disease. An effective therapeutic vaccine would clear viral infections by directing the immune system to produce cytotoxic T cells primed against the foreign HPV antigens that target the infected tissue. A therapeutic DNA vaccine therefore contains a foreign antigen, which for HPV-associated diseases is usually the oncogenic proteins E6 or E7, as well as a mechanism for expressing that foreign antigen in native tissue using mammalian promotors encoded in the DNA vaccine plasmid. The DNA vaccine is injected and expressed by native tissue, driving foreign antigen expression in antigen-presenting cells (APCs) , which stimulate a CD4+ and CD8+ T-cell response against the target antigen. The generation of cytotoxic T cells specific for the target antigen will then clear HPV-expressing cells which contain this foreign antigen. Because the entire E6 or E7 protein is delivered via the DNA vaccine, major histocompatibility complex (MHC) restriction and antigen length is not a limitation with DNA vaccines, since each patient will process these proteins in a different way and present them to the immune system in their own unique immunologic background.
Peptide vaccines function by identifying the major immunologic epitopes for the target protein, again usually HPV E6 or E7, and directly delivering these short, optimized, and immunologically stimulating proteins by injection. The HPV antigenic proteins are directly taken up by dendritic cells and are presented in association with MHC pathways on HLA molecules. This presentation will again generate cytotoxic CD8+ T cells that will eliminate the virally infected cells. In this approach, the proteins are designed and optimized for certain MHC molecules, restricting their use to patients with that particular MHC class (Gérard et al. 2001).
With either approach, HPV-induced immunosuppression in the tumor microenvironment is a concern for many patients with HPV-associated diseases, and finding ways to overcome this local immunologic suppression is a key challenge.
4.5.2 Indications/Implementation
Therapeutic vaccines, unlike the prophylactic L1 and L2 vaccines, can potentially treat patients with active HPV-associated diseases and therefore have broad theoretic applicability to any HPV-associated disease. Because peptide or DNA plasmid administration alone is usually weakly immunogenic, therapeutic vaccines are delivered with electroporation or gene gun. Electroporation in particular has been used in human trials and functions by increasing the permeability of the plasma membrane using an electrical current at the site of the DNA vaccine delivery. This allows robust entry of DNA plasmid into native cells with resultant high levels of antigen expression. Gene gun delivers DNA vaccine-coated gold particles to the dendritic cells in the dermis by using an air-powered needless system. These techniques significantly enhance the levels of antigen expression within the cells (Best et al. 2009).
4.5.3 Efficacy
While there are no commercially available therapeutic vaccines at present, the efficacy of therapeutic vaccines in humans and animal models has been studied. In several animal models, calreticulin (CRT) DNA vaccines induce potential antitumor effects against HPV cell lines by targeting the E7 protein (Peng et al. 2006). Therapeutic vaccines are also undergoing testing in humans. In a recent landmark study, VGX-3100 is the first therapeutic vaccine to be effective against cervical intraepithelial neoplasia grade 2/3 (CIN2/3) associated with HPV 16 and 18 (Trimble et al. 2015). It is composed of synthetic plasmids that target HPV 16 and 18 E6 and E7. VGX-3100 is given intramuscularly by electroporation at 0, 4, and 12 weeks. In a randomized, placebo-controlled trial, almost 50% of vaccinated patients had histopathological regression as compared to 30% of patients who received placebo. Most patients who received vaccine experienced local injection reactions, but there were no serious adverse events.
Peptide vaccines have also been tested in humans. Kenter et al. demonstrated the therapeutic efficacy of a peptide vaccine against HPV 16 for vulvar intraepithelial neoplasia (Kenter et al. 2009). Vaccination with synthetic peptides for E6 and E7 of HPV 16 is effective for 12–24 months for treatment of vulvar intraepithelial neoplasia. The vaccine most likely induces a T-cell response.
4.5.4 Limitations
Therapeutic vaccines cannot provide broad treatment against multiple HPV types as the targeted E6 and E7 antigens are type specific. However, since treatment of specific patients with established disease is the goal of therapeutic vaccination (rather than broad protection of an uninfected population), this is less of a downside than it is for prophylactic vaccination with L1 vaccines. Generating a robust immune response with DNA vaccines or peptide vaccines is the major challenge in humans, and strategies to increase immunogenicity include vaccination delivery techniques or the use of adjuvants like cytokines, chemokines, or Toll-like receptor (TLR) ligands. An effective vaccine would likely require high levels of target gene expression in transfected cells for a prolonged period of time in order maintain a sustained immunologic response. However, it is important to note because DNA-based vaccines cause expression of potentially oncogenic proteins – HPV E6 and E7 – to prime the immune system; these DNA sequences need to be altered to reduce their oncogenic potential to avoid secondary malignancies.
4.5.5 Future Directions
With the recent favorable results of the first human trials of DNA vaccines for HPV-associated diseases, there is substantial interest in using this technology to treat the spectrum of HPV-related diseases. DNA vaccines can be used to target HPV 6 and 11, and preclinical work has already been performed in this area to demonstrate that an immune response can be elicited against viral proteins from these “low-risk” HPV types (Peng et al. 2016; Peng et al. 2010). As methods are developed to enhance the immunogenicity of these therapeutic vaccines, DNA vaccine technology will likely play a role in future treatment of a broad array of HPV-associated diseases, including RRP.
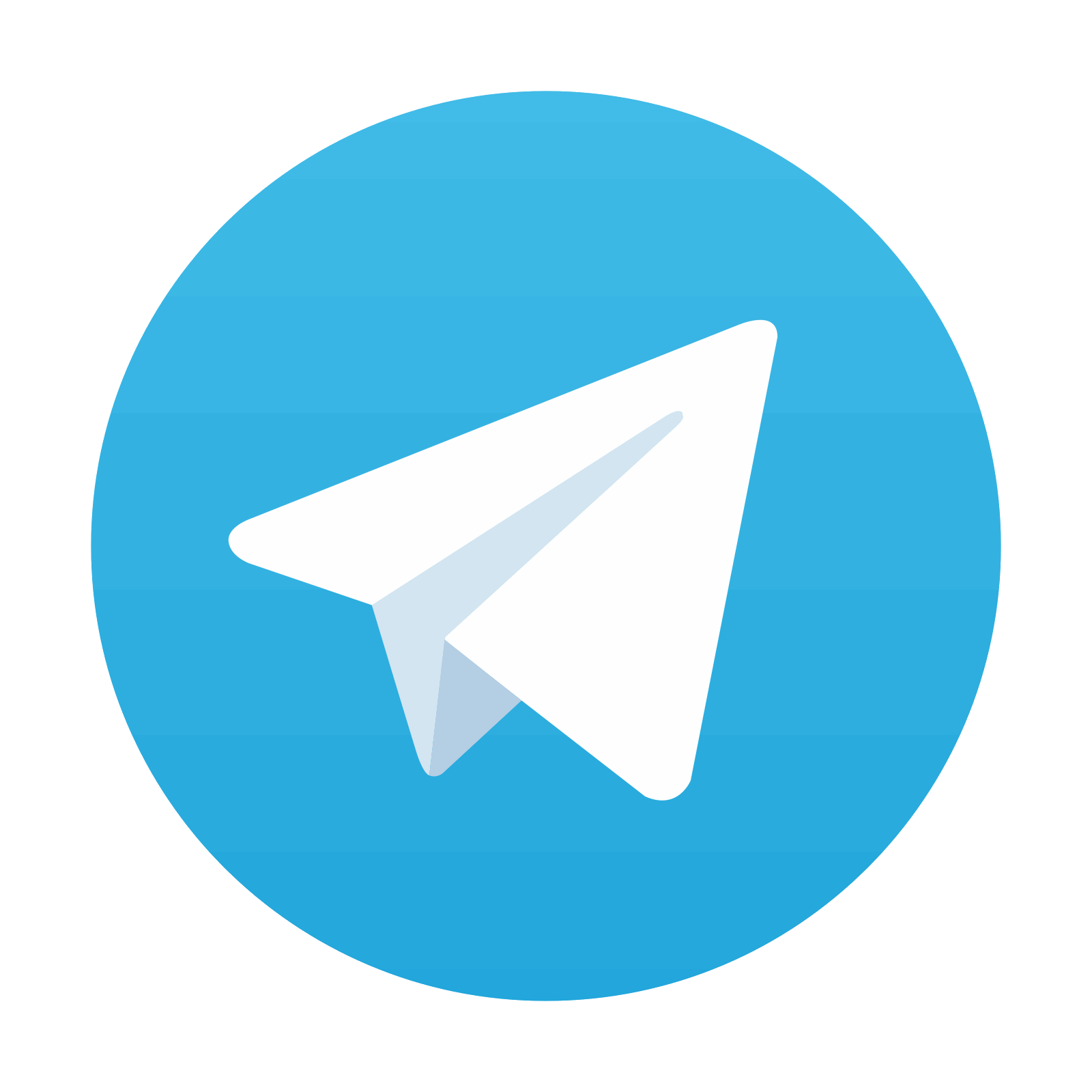
Stay updated, free articles. Join our Telegram channel

Full access? Get Clinical Tree
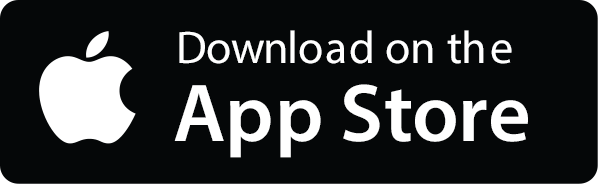
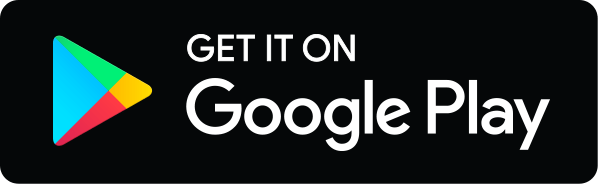