Functional Anatomy of the Extraocular Muscles
Howard M. Eggers
EMBRYOLOGY
The pattern of human eye muscle development is similar to that in elasmobranchs, reptiles, birds, marsupials, and the cat.1,2,3,4,5 A pattern of six extraocular muscles and motor nuclei is present in all vertebrates.6,7 The similarity in muscular architecture and neuronal organization of the extraocular muscles in all vertebrates reflects a highly conserved developmental pattern.
Functionally, the muscles are organized into three pairs that are closely aligned with the axes of the semicircular canals, allowing congruence of sensory and motor frames of reference.8,9 In mammals below primates, an additional retractor bulbi system translates the eye posteriorly to provide a protective reflex in conjunction with the nictitating membrane. This system is reduced to an accessory lateral rectus muscle in primates and is absent in humans.10
The extraocular muscles are composites of connective and muscle tissue that have embryologic origins in different locations. In the vertebrate head, connective tissue and smooth muscle is formed from the neural crest, an ectodermal structure, rather than from mesoderm, as occurs in the trunk. Neural crest cells migrate from their origin in the neural fold and some invade the paraxial mesoderm, where they appear to play a patterning role and contribute to the development of the condensations of mesoderm that give rise to the extraocular muscles.
Mammalian head paraxial mesoderm contains a series of seven hills, called somitomeres,11 demarcated on the surface by shallow furrows. The eighth somitomere is the first somite. Somitomeres give rise to both muscles and connective tissue for the entire head.12 In human embryos of 14 somites (25 days) a pair of premandibular mesodermal condensations may be seen that give rise to the eye muscles innervated by the oculomotor nerve (superior, medial, and inferior recti, and inferior oblique). Two separate tissue masses in the maxillomandibular mesoderm give rise to the lateral rectus and superior oblique. These three primordial condensations on each side of the head (premandibular and the two maxillomandibular) are homologous to the premandibular, mandibular, and hyoid head cavities of lower vertebrates.1 The eye muscle primordia cannot be assigned to segments or metameres, and there is little evidence that the vertebrate head has a metameric organization.
The connective tissue, and not the myogenic precursors, determines the exact site where the muscles form. The mesoderm that gives rise to the eye muscles must have both proper spatial positioning for recognition by the appropriate motor nerve and correct positioning with respect to the eye. It appears that periocular crest cells acquire patterning information appropriate for the site from the optic vesicle.
The myoblasts that give rise to the extraocular muscles are thought to arise from a population of cells that migrates from the somitomere to the presumptive eye muscle region before muscle fibers are formed. The eye muscles form from superior and inferior mesenchymal condensations.13,14 The superior condensation gives rise to the superior rectus, superior oblique, levator, and upper half of medial and lateral recti; the inferior condensation gives rise to the inferior rectus and oblique muscles and the lower half of the medial and lateral recti.
Primary fibers containing the different types of myosin heavy chain form in the absence of innervation. In chick limb muscle, fibers containing all three types of myosin heavy chain (fast, slow, and mixed fast and slow) exist in the muscle before innervation. The primary fibers are replaced with a new population of secondary myoblasts, containing the myosin type of the final muscle.15,16 There are thus two stages of myogenesis.17 In the first, primary myoblasts in populations containing different types of myosin heavy chain are guided to the muscle site by neural crestderived connective tissue. In the second phase, proper formation of secondary muscle fibers requires interaction between nerve and muscle.
The motor axon terminal induces the postjunctional membrane in the muscle to form aggregates of proteins: acetylcholine receptors and acetylcholinesterase. A protein, agrin, is synthesized in the cell body of motor neurons and is transported to the nerve terminals, where it is found in an active form bound to the basal lamina of the nerve terminal. Agrin is thought to mediate the motor neuron–induced aggregation of synaptic proteins at the neuromuscular junction.18
It has traditionally been said that the muscles differentiate from the apex of the orbit forward, whereas the sclera simultaneously differentiates in the opposite direction.3 An alternative view has been put forward that the individual muscles develop concurrently along their entire length.13 The differentiation of myoblasts to mature fibers is dependent on innervation by motor nerves.19 Motor neuron cytogenesis in the brain stem extraocular muscle nuclei undergoes a caudal to rostral gradient that is concurrent with, but independent of, muscle differentiation.20,21,22 At about 1 month, the nerves to the extraocular muscles reach their respective destinations in the sequence oculomotor, abducens, and trochlear. There is initially an overproduction of axons in the motor nerve, followed by a thinning out. This pattern may correlate with loss of polyneuronal innervation or death of excess motor neurons.23,24 An excess of nerve fibers in a small area containing primordia of several extraocular muscles may also relate to occasional anomalous innervation of muscles.25
The trochlea begins to form at 6 weeks of age. The levator palpebrae arises by delamination from the superior rectus during the seventh week, but separation is not complete until the end of the fourth month. Muscle cell striations are recognizable early in the second month; at the end of the second month, strands of long cells have formed.
At about 3 months, the muscle cells have become enveloped by collagen fibers, representing the earliest fascia, and condensations of vascular and perineural adventitia are seen. Connective tissue septa develop a little later; they are formed by the end of the fourth month and continue to enlarge through the second trimester along with the muscle fascia.
The orbital mesenchyma differentiates last.26 Islands of capillary beds develop in the areas of loose mesenchyme between connective tissue septa, and in the fifth month adipose cells arise about these beds, thus completing the overall structural plan of the orbit. By 6 months the muscles are in their final positions. During the last trimester, all the existing tissues and structures enlarge further.
The differentiation of the orbital connective tissue starts later than that of the orbital walls or eye muscles. It is linked with the development of mesenchymal condensations at about 3 months and with the formation of capillary beds at about 4 months, around which the orbital fat develops. The orbital connective tissue attains its adult configuration by 6 months.
Prenatal studies in monkeys have shown that the global mutiplyinnervated, tonically contracting muscle fiber type develops first.27 The chief proprioceptive sensory structure of the eye muscle (the palisade endings) involves this fiber type. Thus, early maturation would allow sensory feedback to be used in muscle development. The last fiber type to mature is the orbital, singly innervated twitch fiber. This fiber contains a rich supply of mitochondria and depends on aerobic metabolism. It is chronically active in its role of positioning the orbital pulley (vide infra).
The growth of the muscle tendons in width and location is linear with time during fetal development.28 The tendon insertions initially extend from the limbus to the equator. With time, the tissue underlying the tendon degenerates, freeing it from the sclera and more clearly defining the exact location of the insertion. The insertion moves farther posterior to the limbus and the anterior segment of the globe enlarges. The muscle insertions continue to change until 18 months to 2 years after birth.13 Infants with esotropia show no anomalies of the medial and lateral rectus tendons, and no correlation exists between the angle of strabismus and the locations of muscle insertion.13
Postnatally, there is further development and refinement in extraocular muscle anatomy as well as changes in the motor nerve (e.g., myelination and distribution of fiber diameters).29 In the rat, postnatal differentiation of fiber types in the orbital layer of the muscle precedes that in the global layer.30 In the cat, the order is reversed.31,32 The changes that occur involve fiber size, maturation of nerve terminals, vascularity, ultrastructural features, histochemistry, and the composition of myosin.
In the primate, the orbital layer twitch fiber shows continued postnatal development of mitochondrial aggregates for up to 6 months.30 This continued development parallels improvements in eye rotation range and fixation stability.
Postnatal muscle development can be disturbed by abnormal visual experience. In cats, lid-sutured eyes have shown decreases in muscle fiber cross-sectional area, oxidative enzyme activity, and capillary density compared with the normal side.34 The concomitant functional alterations are reduced speed of contraction and reduced fatigue resistance.35 In lid-sutured rats, a 15% reduction in the mean fiber size of the trochlear nerve has been found, suggesting an effect on the postnatal development of the extraocular motor neurons.36
In one study, expression of 13 known genes was examined using cDNA microarray in rats raised in the dark to postnatal age of 45 days.37 There was a higher expression level of genes involving tissue remodeling and lower for a gene marker for cellular de-differentiation. Isometric contractile properties were affected. Twitch and tetanic tension were maintained, but twitches were slowed and the muscles fatigued more easily. Reponse at higher stimulation frequencies (over 200 Hz) was significantly lower. Visual experience is thus necessary for development of the normal phenotype.
Extraocular muscle continues to express molecules that are normally expressed in development.38 Studies of rabbit extraocular muscle have shown that there is a population of activated satellite cells (muscle stem cells) that divide and provide daughter myoblasts that fuse with existing adult extraocular muscle myofibers. Labeled nuclei are randomly distributed along the muscle length and are located at the sarcolemmal periphery (characteristic of growth and repair). This may allow regulation of the total number of sarcomeres. Satellite cells in skeletal muscle are activated by injury or exercise. Extraocular muscle appears to have a constant level of satellite cell activation. Local control of satellite cell activation, movement, and turnover is likely.
GROSS ANATOMY
Each eye lies within a bony, roughly cone-shaped cavity in the head, called the orbit. The medial walls of the orbit are approximately parallel to the midsagittal plane, and the lateral walls form an angle of approximately 90 degrees with each other. The medial and lateral orbital walls thus form an angle of approximately 45 degrees with each other. Each orbital axis therefore diverges from the midline by about 22.5 degrees and from the axis of the other orbit by about 45 degrees (Fig. 1)39
![]() Fig. 1. Geometry of the orbits. (Modified from Duke-Elder S, Wybar KC: System of Ophthalmology. St. Louis, Mosby, 1961.) |
MUSCLES
There are six extrinsic extraocular muscles—four rectus and two oblique—that attach to and rotate the eye. They are arranged anatomically and functionally in three pairs.40,41,42,43,44,45 Each of the six muscles attaches at one end to the sclera and at the other end to the bony orbit. Five of these muscles have their origin at the orbital apex; the sixth (inferior oblique) originates at the front of the orbit. A seventh muscle, the levator palpebrae superioris, elevates the upper lid but plays no role in positioning the eye; it will not be directly discussed further. The four rectus muscles—superior, inferior, medial, and lateral—are approximately 40 mm in length and have a width six times their thickness. They arise from a fibrous ring at the orbital apex, the anulus of Zinn, and course forward, unconstrained by connective tissue, as progressively flat strips in a cone-shaped configuration, attaching to the sclera several millimeters posterior to the limbus.46,47,48,49,50,51,52,53,54,55,56,57,58,59 Exact insertion points have been measured in a number of studies; some of the results are excerpted in Table 1. More recently, results of a large and complete series were similar to those of the older studies for the parameters that were measured (see Fig. 3). It shows that the old series measured from the anterior limbal edge to the middle of the insertion. The tendons vary in length: medial 4 mm, inferior and superior 6 mm, and lateral 9 mm. The insertions vary somewhat in shape and location (Table 1, Fig. 2). The distance of the insertions behind the limbus increases in the sequence medial, inferior, lateral, and superior rectus. This progression is known as the spiral of Tillaux (Fig. 3)60
Table 1. Mensuration of Human Extraocular Muscles* | |||||||||||||||||||||||||||||||||||||||||||||||||||||||||||||||||||||||||||||||||||||||||||||||||||||||||||||||||||||||||||||||||||||||||||||||||||||||||||||||||||||||||||||||||||||||||||||||||||||||||||||||||||||||||||||||||||||||||||||||||||||||||||||||||||||||||||||||||||||||||||||||||||||||||||||||||||||||||||||||||||||||||||||||||||||||||||||||||||||||||||||||||||||||||||||||||||||||||||||||||||||||||||||||||||||||||||||||||||||||||||||||||||||||||||||||||||||||||||||||||||||||||||||||||||||||||||||||||||||||||||||||||||||||||||||||||||||||||||||||||||||||||||||||||||||||||||||||||||||||||||||||||||||||||||||||||||||||||||||||||||||||||||||||||||||||||||||||||||||||||||||||||||||
---|---|---|---|---|---|---|---|---|---|---|---|---|---|---|---|---|---|---|---|---|---|---|---|---|---|---|---|---|---|---|---|---|---|---|---|---|---|---|---|---|---|---|---|---|---|---|---|---|---|---|---|---|---|---|---|---|---|---|---|---|---|---|---|---|---|---|---|---|---|---|---|---|---|---|---|---|---|---|---|---|---|---|---|---|---|---|---|---|---|---|---|---|---|---|---|---|---|---|---|---|---|---|---|---|---|---|---|---|---|---|---|---|---|---|---|---|---|---|---|---|---|---|---|---|---|---|---|---|---|---|---|---|---|---|---|---|---|---|---|---|---|---|---|---|---|---|---|---|---|---|---|---|---|---|---|---|---|---|---|---|---|---|---|---|---|---|---|---|---|---|---|---|---|---|---|---|---|---|---|---|---|---|---|---|---|---|---|---|---|---|---|---|---|---|---|---|---|---|---|---|---|---|---|---|---|---|---|---|---|---|---|---|---|---|---|---|---|---|---|---|---|---|---|---|---|---|---|---|---|---|---|---|---|---|---|---|---|---|---|---|---|---|---|---|---|---|---|---|---|---|---|---|---|---|---|---|---|---|---|---|---|---|---|---|---|---|---|---|---|---|---|---|---|---|---|---|---|---|---|---|---|---|---|---|---|---|---|---|---|---|---|---|---|---|---|---|---|---|---|---|---|---|---|---|---|---|---|---|---|---|---|---|---|---|---|---|---|---|---|---|---|---|---|---|---|---|---|---|---|---|---|---|---|---|---|---|---|---|---|---|---|---|---|---|---|---|---|---|---|---|---|---|---|---|---|---|---|---|---|---|---|---|---|---|---|---|---|---|---|---|---|---|---|---|---|---|---|---|---|---|---|---|---|---|---|---|---|---|---|---|---|---|---|---|---|---|---|---|---|---|---|---|---|---|---|---|---|---|---|---|---|---|---|---|---|---|---|---|---|---|---|---|---|---|---|---|---|---|---|---|---|---|---|---|---|---|---|---|---|---|---|---|---|---|---|---|---|---|---|---|---|---|---|---|---|---|---|---|---|---|---|---|---|---|---|---|---|---|---|---|---|---|---|---|---|---|---|---|---|---|---|---|---|---|---|---|---|---|---|---|---|---|---|---|---|---|---|---|---|---|---|---|---|---|---|---|---|---|---|---|---|---|---|---|---|---|---|---|---|---|---|---|---|---|---|---|---|---|---|---|---|---|---|---|---|---|---|---|---|---|---|---|---|---|---|---|---|---|---|---|---|---|---|---|---|---|---|---|---|---|---|---|---|---|---|---|---|---|---|---|---|---|---|---|---|---|---|---|---|---|---|---|---|---|---|---|---|---|---|---|---|---|---|---|---|---|---|---|---|---|---|---|---|---|---|---|---|---|---|---|---|---|---|---|---|---|---|---|---|---|---|---|---|---|---|---|---|---|---|---|---|---|---|---|---|---|---|---|---|---|---|---|---|---|---|---|---|---|---|---|---|---|---|---|---|---|---|---|---|---|---|---|---|---|---|---|---|---|---|---|---|---|---|---|---|---|---|---|---|---|---|---|---|---|---|---|---|---|---|---|---|---|---|
|
The anulus of Zinn is oval in cross section and overlies the optic foramen and the medial portion of the superior orbital fissure (Fig. 4). The lower portion of the ring (tendon of Zinn) is attached to the inferior root of the lesser wing of the sphenoid below the optic foramen and gives rise to the inferior rectus and part of the medial and lateral recti. The attachment site may be developed into a small tubercle, the infraoptic tubercle. The upper portion of the ring (tendon of Lockwood) attaches to the body of the sphenoid and bridges over the superior orbital fissure; where it attaches to the greater wing of the sphenoid, a bony spur may occur (spina recti lateralis). Through the anulus pass the optic nerve, ophthalmic artery, abducens nerve, upper and lower divisions of the oculomotor nerve, and nasociliary branch of the ophthalmic nerve.
The two oblique muscles approach the eye from the front.61,62 The superior oblique muscle arises from the frontoethmoidal suture, superomedial to the anulus of Zinn and the origin of the medial rectus. It courses forward along the junction of the medial orbital wall and roof; after passing through the pulley, or trochlea (Fig. 5), it is redirected posteriorly and inserts in the lateral, posterior, superior octant of the globe (Fig. 6), passing between the superior rectus muscle and the globe. The superior oblique muscle is more rounded or fusiform than the other extrinsic muscles. The inferior oblique muscle arises behind the lower orbital margin on the orbital plate of the maxilla and proceeds in a direct path to its insertion in the lateral, posterior, inferior octant of the globe. It passes below the inferior rectus. The neurovascular bundle to the inferior oblique muscle courses forward along the lateral border of the inferior rectus and enters the oblique muscle at its midportion. Associated with it is strong connective tissue such that when the muscle is disinserted and transposed forward to insert next to the interior rectus, the neurovascular bundle appears to form a new point of origin for contraction of the inferior oblique, inducing a depressing action.63 Mensuration data for the six oculorotary human muscles are shown in Table 1. Table 2 lists the locations of the origins and insertions in a three-dimensional coordinate system. The x-axis is positive laterally, the y-axis positive anteriorly, and the z-axis positive superiorly. The origin is at the center of the eye. These measurements were made usingvarying techniques, and the differences among the sets of measurement are of interest. These coordinates are useful in quantitative calculations of the directions of muscle pull and the three-dimensional cooperation of the extraocular muscles. Figure 7 shows the positions of the extraocular muscles when plotted from such data. Orbital pulleys (vide infra) determine the functional origins of the muscles, and similar coordinates can be provided for each pulley. These coordinates can be determined by analysis of orbital magnetic resonance images obtained with the eye in specified directions. The pulley is at a point that is relatively invariant in position as the eye assumes various directions of gaze.
Table 2. Coordinates of the Points of Origin and Insertion of the Extraocular Muscles for Primary Position of the Eye | |||||||||||||||||||||||||||||||||||||||||||||||||||||||||||||||||||||||||||||||||||||||||||||||||||||||||||||||||||||||||||||||||||||||||||||||||||||||
---|---|---|---|---|---|---|---|---|---|---|---|---|---|---|---|---|---|---|---|---|---|---|---|---|---|---|---|---|---|---|---|---|---|---|---|---|---|---|---|---|---|---|---|---|---|---|---|---|---|---|---|---|---|---|---|---|---|---|---|---|---|---|---|---|---|---|---|---|---|---|---|---|---|---|---|---|---|---|---|---|---|---|---|---|---|---|---|---|---|---|---|---|---|---|---|---|---|---|---|---|---|---|---|---|---|---|---|---|---|---|---|---|---|---|---|---|---|---|---|---|---|---|---|---|---|---|---|---|---|---|---|---|---|---|---|---|---|---|---|---|---|---|---|---|---|---|---|---|---|---|---|
|
![]() Fig. 7. Projection of the positions of the extraocular muscles onto a horizontal plane. Dimensions, to scale, are from measurements in rectilinear three-dimensional coordinates (see Table 2, Ruete’s figures). The oblique muscles have nearly the same plane of action. (Modified from Hering E: The Theory of Binocular Vision. New York, Plenum Press, 1977.) |
The eye muscles grow with the eye and orbit in early childhood.65,66,67 The lengths in infancy and at age 1 year are listed in Table 3. Lengths in adults are the same within measurement error. Note that in Figure 8, which shows an infant eye and an adult eye superimposed, the angular proportions stay the same. Eye muscle lengths increase by about the same proportion (40% to 50%). Thus the percent contraction of each muscle stays the same for a given size eye movement, and it seems probable that the central innervation patterns need only minor adjustments to compensate for growth.
Table 3. Growth in Length of the Rectus Muscles with Age (mean ± standard deviation) | |||||||||||||||||||||||||||||||||||||||||||||||||
---|---|---|---|---|---|---|---|---|---|---|---|---|---|---|---|---|---|---|---|---|---|---|---|---|---|---|---|---|---|---|---|---|---|---|---|---|---|---|---|---|---|---|---|---|---|---|---|---|---|
|
![]() Fig. 8. Growth of the eye and extraocular muscles based on measurements of the adult (Volkmann)55 and infant (Schneller66) Dimensions are given in millimeters. Note how angular relationships are preserved even though lengths change. (Modified from Mühlendyck H: Wachstum und Lange der ausseren Augenmuskeln. Ber Dtsch Ophthalmol Ges 75:449, 1978.) |
The monkey is commonly used as a model for human anatomy and physiology.68 The orbit in the monkey is somewhat different in shape from that in humans; consequently the muscles have qualitatively different primary position actions. The monkey orbital axis is 2 degrees more lateral and points upward by 15 degrees, compared to 1 degree downward in humans. Monkey horizontal recti have a significant torsional action, unlike those in humans Monkey vertical recti ack the adducting action present in humans. In the primary position, the oblique muscles of the monkey have a more vertical than torsional action.
BLOOD SUPPLY
The extraocular muscles are supplied by the muscular branches of the ophthalmic artery, which usually occur as two main stems and a varying number of smaller twigs.44,45 Further blood supply may arise from the lacrimal and infraorbital arteries. The venous drainage of the extraocular muscles is into the superior and inferior orbital veins.
The lateral (or upper) muscular branch of the ophthalmic artery supplies the lateral and superior rectus, superior oblique, and levator muscles. The levator may also receive twigs from the supraorbital artery. The medial (or inferior) muscular branch, the larger of the two, supplies the medial and inferior recti and the inferior oblique. The infraorbital artery sends a branch to the inferior oblique. The lacrimal artery sends branches to the lateral and superior recti.
The extraocular muscles have fibers in two layers: orbital and global. Each contains different types of muscle fibers (vide infra). The orbital layer has continually contracting, mitochondria-rich fibers that use aerobic metabolism; therefore, the blood supply to the orbital layer is greater than that to the global layer. The orbital layer thus has more vessels per unit of area, more vessels per muscle fiber, and more vascular lumenal area than the global layer.69
The anterior ciliary arteries, which supply blood to the anterior segment of the eye, pass through the rectus muscles and penetrate the sclera at the muscle insertions. There are two anterior ciliary arteries in each rectus except the lateral, which has only one. The anterior ciliary arteries merely pass through the rectus muscles and do not supply blood to these muscles. Anterior segment ischemia may result from interruption of the anterior ciliary arteries; a clinical rule of thumb is to avoid surgery on more than two rectus muscles at a time to minimize this risk.
INNERVATION
The third cranial nerve (oculomotor) divides within the orbit into superior and inferior branches. The superior division innervates the superior rectus and levator palpebrae. The inferior division innervates the inferior and medial recti and the inferior oblique. The fourth (trochlear) nerve innervates the superior oblique, and the sixth (abducens) cranial nerve innervates the lateral rectus.44,45
The nerves enter the rectus muscles from within the muscle cone at approximately the junction of the middle and posterior thirds of the muscle (for the superior, medial, and inferior recti) and just posterior to the middle (for the lateral rectus). The superior oblique is supplied by its nerve in several branches that enter the anterior half of the posterior third of the muscle, on its orbital surface. The inferior oblique is supplied at about the middle of its posterior border.
ORBITAL TISSUES
The orbital tissues of importance for the extraocular muscles are Tenon’s capsule70 and the system of connective tissue septa that span the orbit. The literature contains numerous descriptions of the orbital connective tissue and Tenon’s capsule based on gross dissection or injection of preserved specimens. These techniques tend to produce nonexistent fascial planes and ligaments. Whitnall’s study of Tenon’s capsule in fresh postmortem preparations has given us one of the most accurate descriptions of this structure.44
Tenon’s Capsule
Tenon’s capsule, or the fascia bulbi, is a layer of delicate connective tissue that completely envelops the globe from the limbus to the optic nerve, forming a potential space free of any tissue attachments that might restrict globe rotation. Posteriorly, it is loosely attached by extremely fine connective tissue filaments to the sclera. This episcleral space contains no fluid or endothelium and is not a full-fledged ball-and-socket joint in function. The globe can rotate some within the capsule; however, beyond small rotations, the globe and capsule move together, deflecting the orbital fat attached behind. This is to be expected, since the capsule is attached firmly to the eye at the limbus and to the muscles at their points of entrance.
The anterior one third of the capsule lies anterior to the rectus muscle insertions and is thinner and more adherent to the globe, fusing to the sclera at the corneoscleral limbus. On its outer surfaces, Tenon’s capsule in front is loosely adherent to the conjunctiva and can be readily separated from it. In contrast, the posterior capsule is firmly attached to the orbital fat and connective tissue meshwork.
Tenon’s capsule is penetrated by the optic nerve, the extraocular muscles, and the blood vessels and nerves serving the globe. About the optic nerve the capsule is penetrated and broken up by multiple posterior ciliary arteries and nerves, and some have considered it to cease a few millimeters away from the optic nerve. There is no sheath continuing backward around the optic nerve.
The rectus muscles pierce Tenon’s capsule anterior to the equator of the globe and pick up sleevelike extensions of the capsule. The intracapsular portion of the rectus muscles is 7 to 10 mm in length. From the point of capsular penetration to the insertion, each muscle has fascial expansions from its edges that attach to the overlying Tenon’s capsule, forming falciform folds,72 and insert into the sclera lateral and posterior to the muscle insertion. Small attachments between the undersurface of the muscle and the sclera just behind the muscle insertion are called footplates. Outside Tenon’s capsule, in their posterior one third, the muscles have only a very thin sheath. Closer to Tenon’s capsule the muscle sheaths become thicker and more opaque and, for a distance of 10 to 12 mm, give off lateral expansions that connect between adjacent muscles to form an intermuscular membrane. The intermuscular membrane has only a limited extent and is not found more than a short distance behind the globe.
The medial and lateral rectus muscle sheaths give off attachments from their external surfaces that are known as ailerons or check ligaments. These arise from the muscle sheath anterior to the point of muscle passage through the capsule and insert on the zygomatic tubercle (for the lateral rectus) and on the lacrimal bone behind the lacrimal crest (for the medial rectus). The muscles do not move within their sheaths so that these attachments limit extreme rotations of the eye; their severance or fibrosis can free the rotation even more or can restrict it. In addition to “checking” rotation, these attachments help to anchor the whole fascial apparatus in position.
The orbital fat is prevented from attaching to the globe by Tenon’s capsule. Sometimes Tenon’s capsule is perforated during surgery and the orbital fat has been sufficiently traumatized that adhesions can form between the fat and the globe. Such an adhesive syndrome significantly impairs eye rotations. Penetration of Tenon’s capsule can release fat as forward as 10 mm behind the limbus.
The sheath of the superior rectus blends with that of the overlying levator palpebrae superioris and assists synergic action between the two muscles. This explains the apparent or “pseudo” ptosis that accompanies a hypotropia. The forward expansion of the inferior rectus passes from the muscle undersurface above the inferior oblique and inserts between the tarsus and orbicularis oculi muscle. This mechanical linkage helps to lower the lid on downgaze. It also explains a change in lid fissure width after inferior rectus surgery. Resections of this muscle tend to raise the lower lid and narrow the lid fissure, whereas recessions tend to widen the lid fissure by lowering the lid. Each rectus muscle sends connective tissue expansions that attach to and retract the conjunctival cul-de-sac. When the eye moves, this attachment prevents the conjunctiva from dragging over the cornea.72
Pulleys
The structure and organization of the orbital connective tissue have long been neglected. Koornneef, using a thick section histologic technique,73 has demonstrated the consistent architecture of this system.74,75,76 Periocular and retro-ocular orbital spaces are filled with fat pads organized as adipose tissue space, surrounded by collagenous strands and septa. The septa are 0.3 mm or more in thickness and subdivide the orbital volume. The strands consist of large, meshed, collagenous tissue containing fibroblasts and collagen fibers. Anteriorly, the septa are radial around the globe and connect the periorbita to Tenon’s capsule and the intermuscular membrane (Figs. 9 and 10).
Sleeves or pulleys anchor the positions of the rectus muscles near and posterior to the equator of the eye.77,78 The pulleys lie within Tenon’s fascia and are composed of dense collagen bands woven at right angles and supported from the orbital walls by elastin and smooth muscle bundles.79 These bands determine the functional origin of the muscles and have consistent locations across all subjects. Coronal plane coordinates have a 95% confidence interval of less than 1 mm.80 The muscles telescope within the pulley, sliding on thin collagenous sheets (Figs. 11 and 12).
The smooth muscle is innervated by projections from the superior cervical ganglion using norepinephrine, from the pterygopalatine ganglion using nitroxidergic innervation, and from the pterygopalatine or ciliary ganglion using acetylcholine.83 Innervation of the smooth muscle anchoring the pulleys may have a functional role of maintaining or correcting pulley location. Magnetic resonance imaging (MRI) studies have shown that the pulleys have stable locations with varying directions of gaze, generally shifting less than a millimeter transversely in a coronal plane with eye rotations. Excessive shifts in pulley location result in incomitant strabismus.83 The inferior oblique and inferior rectus pulleys are mechanically coupled via Lockwood’s ligament. Smooth muscle in the pulley suspension runs from the medial to the inferior rectus pulley, constituting Muller’s peribulbar muscle.
Each extraocular muscle has two layers: orbital and global (vide infra). The orbital layer inserts on the pulleys so that muscle contraction will translate the pulley posteriorly. The orbital layer of the inferior rectus inserts on the lateral rectus pulley and the inferior oblique pulley. Partial coupling of the inferior rectus with the inferior oblique pulley moves its axis with vertical gaze. The orbital layer of the inferior oblique inserts on the inferior rectus pulley, translating it nasally with contraction of the inferior oblique. The orbital layer of the superior oblique inserts on a dense collagenous sheath posterior to the trochlea. The sheath goes through the trochlea and then inserts on the nasal border of the superior rectus pulley, pulling it nasally when the superior rectus contracts.84
The so-called check ligaments and the suspensory ligament of Lockwood are part of this system. Postoperative fibrotic changes in these septa are important in restricting ocular motility. Lockwood’s ligament is an expansion of the inferior rectus sheath to the surrounding septa to form a suspensory hammock for the globe.
The orbital connective tissue constrains the position of the eye muscles. The rigidity of the resulting musculo-orbital coupling determines how far the muscles shift with eye movements and consequently plays a role in determining the exact eye movement resulting from a change in muscle innervation. Thus, when the eye looks up, the horizontal rectus muscles move upward only in the portion distal to an anchoring point near the ocular equator. This preserves the purely horizontal movement produced by the horizontal rectus muscles and limits cross-coupling of the action of one muscle to another.
MICROSCOPIC ANATOMY
CONNECTIVE TISSUE
The connective tissue surrounding the extraocular muscles is more delicate than that around ordinary skeletal muscles. The muscle fibers are distributed in a capillary-rich, loose connective tissue. The capillaries in extraocular muscle are of the closed type, with the bidirectional transfer of macromolecules achieved by transendothelial vesicular transport.85
Adjacent to the surface of each muscle fiber (cell) is a layer about 50-nm thick that stains as a mucopolysaccharide. This, in turn, is surrounded by a delicate reticulum of argyrophilic (collagenous) fibers (Fig. 13) and elastin fibers (Fig. 14).86,87 The fibers are uniformly distributed, and the muscle is penetrated by a few intramuscular septa (the internal perimysium) that consist of collagenous fibers and contain many nerves and blood vessels. Argyrophilic fibers occur at the muscle fiber–connective tissue junction. The internal perimysium is continuous with the external muscle sheath or capsule (external perimysium or epimysium). Between the muscle fibers are numerous nerve fibers. Capillary vessels are most numerous on the outer, orbital surface of the muscle, which contains the smaller muscle fibers that are constantly active. Blood flow measurements in the cat show that the average flow in the six extraocular muscles is greater than that in any skeletal muscles and exceeded only by that in the myocardium.
MUSCLE FIBERS
Eye muscle histology is a subject vexed by confusion and controversy. This is partly due to interspecies variation in the eye muscles but also to difficulty in making correlations between structure and function. The basis for understanding the extraocular muscle fiber types is the work that has been done on skeletal muscle. With the use of a variety of techniques—physiological,88 histochemical,89,90 biochemical,91,92 immunocytochemical,93,94,95 and ultrastructural93,96,97,98—three or four fiber types have been delineated in skeletal muscle. When studied in detail, the extraocular muscle fibers are not exactly like those of any skeletal muscle.
In broad outline, extraocular muscle shares with skeletal muscle a spectrum of twitch fibers, differing in rapidity of contraction and in fatiguability (“white” versus “red”). In addition, there are tonically contracting fibers, which do not occur at all in skeletal muscle. Skeletal muscles differ one from another in the proportion of fiber types. In contrast, except for one fiber type, all extraocular muscles are similar in fiber type composition. Like skeletal muscle, all fibers innervated by one axon are of the same type.
Light Microscopy
A gross muscle contains clusters of adjacent parallel muscle cells that are delimited by connective tissue septa. Such a cluster is a fasciculus. The muscle cells, which are also called fibers, are roughly cylindrical, multinucleated cells bounded by a plasma membrane. The ends fuse with the inelastic tissue of the muscle tendon.
The extrinsic eye muscles have structural features not found in limb muscle, and the microscopic appearance is therefore somewhat different from that of ordinary skeletal muscle. This is not surprising in view of the unique functional abilities of the extraocular muscles—constancy of activity, rapidity, and fine gradation of contraction. Extraocular muscles have a lower innervation ratio, greater vascularity,99 and a looser connective tissue envelope, rich in elastic fibers,86,87 than are found in skeletal muscle.
Normal extraocular muscle has features that in skeletal muscle are associated with myopathy. The fiber shape and size are variable, and there are more small fibers.100 There is usually a mild mononuclear inflammatory cell infiltration. Compared with skeletal muscle, there are more fibrous connective tissue and internal nuclei. Thus, ordinary myopathy and neuropathy cannot be distinguished in extraocular muscles using conventional morphologic criteria.101 Furthermore, individual fibers may show changes, observable by light or electron microscopy, which in skeletal muscle are considered pathologic. Such changes are whorled fibers, ringbinden fibers, vacuoles within myofilament bundles, subsarcolemmal inclusions, sarcomere disruption, nemaline rods, smearing of Z lines, mitochondrial clumping, mitochondrial abnormalities, zebra bodies, and Hirano bodies.102 These findings occur in children as well as in adults.
The early histologic literature contains evidence of more than one fiber type in extraocular muscle. Fibers were distinguished by diameter, content of sarcoplasm, size and location of nuclei, fibrils, and presence of hypolemmal rings. Thus, in 1910, Cilimbaris103 noted clear fibers with few nuclei and little sarcoplasm, dark fibers with many nuclei and plentiful sarcoplasm, and intermediate types. Thulin,104 in 1914, described three muscle types in human and monkey extraocular muscle. The first contained irregular fibrils of varying size (i.e., Felderstruktur). Furthermore, he mentioned that this type had nerve terminals of a special structure (which he did not describe). A second type showed abundant cytoplasm with a regular arrangement of fibrils (i.e., Fibrillenstruktur). The third type had a fibrillar, cross-striated appearance but also had hypolemmal, circularly arranged fibrils. These are now known as ringbinden fibers and probably represent a degenerative change.
Kato, in 1938,51 distinguished two zones within extraocular muscle, differing in cell size and staining reactions: an inner layer next to the eye itself (the global layer) and an outer, orbital layer. Sometimes an additional intermediate zone can be distinguished. In rectus muscles the orbital layer is C-shaped. The oblique muscles are more circular in cross section and have a concentric arrangement of orbital and global layers. The intermediate zone is conceived of as having an admixture of cell types from the orbital and global layers. It is now known that in the rectus muscles each layer has a different insertion.105 The orbital layer inserts on a connective tissue pulley that constrains the muscle path in the orbit, and the global layer inserts on the globe itself. The global layer extends the entire length of the muscle and through the pulley without inserting. The orbital layer does not extend as far because it inserts on the pulley, located in the equatorial region of the eye.106
Siebeck and Kruger, in 1955,107 clearly identified two types of muscle fiber in extraocular muscle on the basis of light microscopic appearance and introduced the names Fibrillenstruktur and Felderstruktur. Functionally, Fibrillenstruktur fibers are similar to the twitch fibers of ordinary skeletal muscle and Felderstruktur fibers to tonically contracting muscle, most studied in amphibians. The muscle fibers (cells) are arranged longitudinally, many fibers probably running most of the length of the muscle,108 although there have been reports of fibers terminating or arising along the course of the muscle.108,109,110 Most muscles have fibers that are shorter than the muscle length.111 Cross sections of the muscle at various points along its length reveal a varying proportion of different fiber sizes.110,112,113,114 In humans, the global layer contains cells with a mean diameter about 50% greater than that of the orbital layer.
Serial sections show fibers to be most numerous in mid orbit.106 Mean numbers across four human specimens, ranging in age from 17 months to 93 years, were approximately 5,900 for the medial, 5,600 for the lateral, and about 4,000 for the vertical recti. In an older study of human inferior rectus muscles, a predominance of small fibers was found proximally95; in rabbits, a predominance was found in the distal part of the muscle.109
The fiber numbers also differ by layer. The human rectus muscle global layers all have approximately 12,000 fibers. The orbital layers vary from 37% to 49% of the total fiber count. Thus the orbital layer has a little less than half the fibers. The global layer is expected to be more uniform because the globe has the same inertia and viscosity in all directions. The orbital layers insert on the orbital connective tissue (pulleys) which couples to the orbital wall, equatorial Tenon’s capsule, and adjacent extraocular muscles with elastin, collagen, and smooth muscle. The fiber counts vary in proportion to the connective tissue load constituted by each pulley.
Serial sections of rabbit rectus muscles have been performed114 and show marked, systematic variation of fiber structure and, in some fibers, of diameter, with position along the fiber; this has not been shown in the mouse.113 Relatively little is known about short fibers, which may occur at either end of the muscle, although they may be muscle stem cells (satellite cells).38,113,114,115,116,117 Such variation could mean that all the large fibers do not travel the full length of the muscle, that the fibers taper, or that the fibers split into several smaller branches—as observed by Hines109 in rabbits at either end of the muscle. Lockhart and Brandt108 be lieved the fibers extended the entire length of the muscle in human eyes, as did Cooper and Daniel.115 This is significant because if some fibers do not run full length, a cross section of the muscle will not show every fiber, and the number and proportion of fiber types determined at one point may be invalid elsewhere in the muscle.
By using plastic resins for embedding, it is possible to section the tissue thin enough to obtain adequate resolution to count the small muscle fibers accurately. In cat inferior oblique muscle, Alvarado and van Horn110 found the greatest number of fibers in the middle third of the muscle, the count dropping toward either end of the muscle. Some fibers terminated several millimeters short of the ends. The longest fibers ran the entire length of the muscle. A significant number of small fibers terminate within the muscle. Other departures from a simple parallel arrangement have been found in animals—branching of muscle cells near their ends in the rabbit109 and sheep117 and myomyous junctions between short cells in the cat,118,119 rat,120,121 and sheep.117 A similar arrangement of fibers exists in skeletal muscle.
In human material, Voss112 found only small fibers at the muscle end but larger fibers as well in the typical layered arrangement toward the middle. However, he did not do quantitative fiber counts along the length of the muscle. In gross serial sections of the orbit, Nakagawa53 found that the rectus muscle area was maximum at the level of the posterior surface of the globe (5 mm behind the posterior surface of the globe for the lateral rectus), and the muscles tapered uniformly anterior and posterior to the zone of maximum area. Muscle cell branching and myomyous junctions have not been reported in human material.
In cross section, fibers in the eye muscle are not as tightly packed together as those in skeletal muscle.100 In skeletal muscle, the fibers are arranged in compact bundles and each fiber is polygonal in cross section. There is a scant amount of connective tissue between fibers, but thick septums penetrate between bundles. In the extraocular muscles each cell is separated enough from its neighbors to be approximately round in shape and to have its own fine connective tissue sheath. There are few large septa between fibers, and the interfiber spaces contain a multitude of nerve fibers.
Light and electron micrographs of muscle show a typical cross-striated pattern formed by a registration of sarcomeres in adjacent myofibrils (Fig. 15). The transverse striations are due to the arrangement of filaments into alternating isotropic (I) and anisotropic (A) (i.e., doubly refractile) bands. The I band is transversely bisected by a dark Z line. The sarcomere varies in length from about 1.5 to 3.5 μm depending on the amount of shortening of the muscle.
![]() Fig. 15. Electron micrograph of a twitch fiber in human extraocular muscle. This fiber shows less development of the sarcoplasmic reticulum and T system than the cell in Figure 17. Mitochondria occur in rows between the myofibrils. This cell would be classified as a “red” twitch fiber. The diagram below the electron micrograph shows how the filaments produce the banding pattern. (Courtesy of Dr. T. Iwamoto.) |
The I band contains only thin filaments 5 to 6 nm thick and approximately 1 μm in length composed of three proteins: actin, troponin, and topomyosin. The A bands contain thicker (1.1 nm) filaments, which are about 1.5 μm in length and are composed of the protein myosin. The thick and thin filaments overlap within the A band, where they are linked by crossbridges; a central zone free of thin filaments in the center of the A band is called the H zone (Hensen’s stripe). An inconsistent feature is a line centered within the H zone, the M line (Mittelscheibe); its presence depends on the species.
Fiber Types
The variety of fiber types is bewildering, all the more so considering the difficulty in drawing correspondences among various species. There are two basic classes of muscle cells in extraocular muscle: singly innervated (twitch, conducted action potential) and multiply innervated (tonic, graded contraction, no conducted action potential). The different types of muscle fiber can be classified by their location within the muscle (orbital versus global layer), innervation (single or multiple), metabolism (oxidative versus glycolytic), and speed of contraction (fast or slow twitch versus sustained, graded contraction). A cross section of extraocular muscle shows a layered organization, in which a layer of smaller diameter cells (5 to 15 μm) is on the orbital surface (orbital layer), and the bulk of the muscle adjacent to the globe (global layer) contains larger cells of 10 to 40 μm diameter51,100,101,112 (Table 4). The muscle cells of various diameters may have many other differing structural features, and the muscle fibers may be grouped into several classes on the basis of structure and function (Table 5).
Table 4. Average Fiber Diameters in Human Extraocular Muscle* | ||||||||||||||||||||
---|---|---|---|---|---|---|---|---|---|---|---|---|---|---|---|---|---|---|---|---|
|
Table 5. Correspondence of Human Muscle Fiber Type Properties | |||||||||||||||||||||||||||||||||||||||||||||||||||||||||||||||
---|---|---|---|---|---|---|---|---|---|---|---|---|---|---|---|---|---|---|---|---|---|---|---|---|---|---|---|---|---|---|---|---|---|---|---|---|---|---|---|---|---|---|---|---|---|---|---|---|---|---|---|---|---|---|---|---|---|---|---|---|---|---|---|
|
The two basic functional types of muscle fiber were first observed in the frog as fast and slow contractile systems by Sommerkamp,122 and the two morphologic patterns were described and named by Kruger.123 Ordinary light microscopy shows two anatomic patterns of the contractile fibrils within the muscle fibers that correspond to the two innervation patterns. The fibrils in singly innervated fibers are arranged in discrete bundles separated by sarcoplasmic reticulum (Fibrillenstruktur). Multiply innervated fibers have a poorly developed sarcoplasmic reticulum and irregular, larger myofibrils that are partly fused (Felderstruktur). In hematoxylin-eosin stained light micrographs, Fibrillenstruktur fibers stain palely and have a finely stippled appearance, regular spacing of fibrils, and mostly peripherally located nuclei. Felderstruktur fibers stain more darkly and have irregularly clumped fibrils that are not clearly separated; their nuclei are central as well as subsarcolemmal.
In addition to light microscopy, electron microscopy and histochemical reactions are used as an aid in classification. Through testing the activity of various metabolic enzymes, it is possible to obtain a better estimate of the functional capabilities of a cell.
The overall plan of organization of fiber types is not as complex as it first seems if one does not too closely compare detailsof morphology across species. Table 6 shows that, in fact, there is considerable agreement among species in the broad outlines of fiber architecture. All the muscles listed have similar fiber arrangements (Table 7). Each muscle has two layers. The outer or orbital surface layer contains small-diameter, singly innervated twitch fibers and small-diameter, multiply innervated tonic fibers, both containing many mitochondria. Morphologically, these fibers are capable of prolonged contractile activity without fatigue. The inner or global layer of muscle contains a spectrum of three types of singly innervated twitch fibers (“red,” “intermediate,” and “white,” according to mitochondrial content, development of sarcoplasmic reticulum, and diameter) and a “white,” multiply innervated tonic fiber. If the global, red twitch fiber is considered to be the same as the similar cell in the orbital layer, there are only five types of fiber. The orbital twitch fiber is distinct, however, in the extreme extent to which its morphology is affected by botulinum toxin chemodenervation.124
Table 6. Fiber Types in Extraocular Muscle | ||||||||||||||||||||||||||||||||||||||||||||||||||||||||||||||||||||||||||||||||||||||||
---|---|---|---|---|---|---|---|---|---|---|---|---|---|---|---|---|---|---|---|---|---|---|---|---|---|---|---|---|---|---|---|---|---|---|---|---|---|---|---|---|---|---|---|---|---|---|---|---|---|---|---|---|---|---|---|---|---|---|---|---|---|---|---|---|---|---|---|---|---|---|---|---|---|---|---|---|---|---|---|---|---|---|---|---|---|---|---|---|
|
Table 7. General Schema of Mammalian Extraocular Muscle Fiber Architecture | ||||||
---|---|---|---|---|---|---|
|
Within a species, the extraocular muscles may differ from one another in the proportion of the fiber types.125 In the human, the largest difference is in the percentage of twitch fibers in the orbital layer. The medial rectus has the highest percentage of twitch fibers in the orbital layer. The medial rectus has the highest percentage and the lateral rectus the lowest percentage of these fibers.101
The orbital layer twitch fiber shows the most variation in mitochondrial content between species. Mitochondrial content is most highly developed in primates. In lower species, rows of numerous and large mitochondria lie between myofibrils in the center of the cell. In primates, this fiber type has, in addition to the central mitochondria, large subsarcolemmal clusters of mitochondria, correspondingly appropriate oxidative enzyme profile on histochemistry and a rich capillary supply. In the global layer, the most prominent species dif ference is the diameter of the muscle fibers.
Electron Microscopy
Extraocular muscles were first examined with the electron microscope in the guinea pig by Hess in 1961.126 His work clearly showed two morphologic fiber types. Hess and Pilar,127,128 in 1963, extended this work to the cat and combined it with physiologic studies establishing two types of contractile fiber. Initial work on other species confirmed these two broad types of muscle fiber in cats,129 rabbits,130,131 monkeys,132,133 and humans.134,135,136 Combined with histochemistry, five muscle fiber types were shown in monkeys.137
Beyond fiber size and location in the muscle (orbital or global layer) and features observable by light microscopy, electron microscopy enables observation of many morphologic details by which fibers may be classified. The features used for classification include diameter; position in the muscle; number of mitochondria; development of endoplasmic reticulum; size and development of transverse tubules; arrangement of fibrils; fine structure of fibrils, such as presence of the M line or thickness of the Z line; various biochemical properties, such as the amount of glycogen or lipid droplets, or the activity of various metabolic enzymes; and the innervation pattern, which is single or multiple.
Generally, the grouping of features for any single parameter is loose, specifying, for example, more or less sarcoplasmic reticulum. However, after observation of enough fibers, associations between these morphologic parameters are evident. In transverse electron micrographs, the most reliable criteria to classify fiber types are size and delineation of myofibrils; size, number, and location of mitochondria; and development of sarco plasmic reticulum and T tubules. These subcellular morphologic details relate directly to the functional specialization of the various fiber types. Detailed electron microscopic work has shown five to seven types of muscle fiber based on ultrastructural morphology in each species studied: mouse,113 rat,116,138 rabbit,139 sheep,117 cat,110,140 and monkey.141,142 Spencer and Porter have reviewed the comparative ultrastructural morphology of the various fiber types in detail.143 Table 6 lists some correspondences among several electron microscopic studies of extraocular muscle fibers that have been done in mammals.101,113,114,116,117,137,140,144
The external cell membrane, the sarcolemma, is a bilamellar leaflet 7.5- to 10-nm thick that is universal to all cells. This, in turn, is surrounded by a basement membrane 30- to 50-nm thick and a very delicate connective tissue meshwork. Some authors consider the sarcolemma to contain both the plasma and basement membranes. Each cell contains internal substructures, known as filaments, that are arranged in repeating subassemblies, called sarcomeres, and into parallel structures called fibrils (or myofibrils), which produce the contractile force of the muscle. The bulk of each fiber consists of myofibrils that lie parallel to the length of the cell and run almost end to end. The very ends of the cell taper. The myofibrils are cylinders and have no membrane sheath. In eye muscle they range from 0.1 to 0.5 μm in diameter. In addition to myofibrils the cytoplasm of the muscle cell contains multiple nuclei, Golgi apparatus, mitochondria, glycogen granules, ribosomes, lipid bodies, transverse tubules, and sarcoplasmic reticulum.
The spaces between the fibrils are interlaced with two membranous systems, the transverse tubular system (T system) and the sarcoplasmic reticulum, a specialization of the endoplasmic reticulum of cells in general. The T system is continuous with the sarcolemma, and large molecules added to the bathing medium may be found within the T system. The T system penetrates between the fibrils, in places seeming to encircle them, at a location along the sarcomere that is species specific; in human extraocular as well as skeletal muscle it is at the junction of the A and I bands. The sarcoplasmic reticulum contains irregular tubules and sacs that also lie in the spaces between the fibrils and, at the A–I junction, forms small cisternae that closely abut the transverse tubule.145,146 This close association, however, does not form a tight junction. The cluster of T tubule plus cisternae on either side is called a muscle triad.
The multiple nuclei are elongated in the direction of the long axis of the cell and are up to 11 μm in length. The nuclei are surrounded by an inner and an outer membrane 50 nm apart that are penetrated by pores 50 to 70 nm wide. Near the nucleus lies the Golgi apparatus, consisting of a closely spaced group of flattened smooth tubules.
The mitochondria can occur under the plasma membrane, aggregated around nuclei and under synapses, and between myofilaments. They have a double membrane separated by 8 nm. The inner membrane infolds to form the cristae within the mitochondrion. The mitochondria contain oxidative enzymes.
Lipid bodies, 0.5 to 1 μm in diameter, are sometimes membrane bound. They occur under the plasma membrane, near mitochondria, and around the nuclei. Lipid bodies are commonly accepted as being a type of lysosome.147
Ribosomes, particles 10 to 15 nm in diameter, are difficult to distinguish from glycogen granules. They are somewhat smaller than glycogen granules but are not usually seen in muscle sarcoplasm.148
Human extraocular muscle has been less exhaustively studied by electron microscopy, although three types of muscle fiber can be easily demonstrated in humans (Figs. 15, 16, 17, and 18). Mukuno149 found evidence for up to six fiber types in human extraocular muscle, but these were not exhaustively studied (Table 8). Electron microscopy of Fibrillenstruktur fibers shows several morphologic varieties based on distribution of mitochondria, sarcoplasmic reticulum, glycogen, and M lines. This suggests more than a simple division into red and white fibers. However, electron microscopic studies of human extraocular muscle have focused on the Fibrillenstruktur and Felderstruktur distinction,134,135 and no comprehensive subdivision of Fibrillenstruktur fibers has appeared.
Table 8. Electron Microscopic Classification of Human Extraocular Muscle Neuromuscular Junctions and Corresponding Fiber Morphology | |||||||||||||||||||||||||||||||||||||||||||||||||||||||||||||||||||||||||||||||||||||||||||||||||||||||||||||||||||||||||||||||||||||||||||||||||||||||||||||||||||||||||||||||
---|---|---|---|---|---|---|---|---|---|---|---|---|---|---|---|---|---|---|---|---|---|---|---|---|---|---|---|---|---|---|---|---|---|---|---|---|---|---|---|---|---|---|---|---|---|---|---|---|---|---|---|---|---|---|---|---|---|---|---|---|---|---|---|---|---|---|---|---|---|---|---|---|---|---|---|---|---|---|---|---|---|---|---|---|---|---|---|---|---|---|---|---|---|---|---|---|---|---|---|---|---|---|---|---|---|---|---|---|---|---|---|---|---|---|---|---|---|---|---|---|---|---|---|---|---|---|---|---|---|---|---|---|---|---|---|---|---|---|---|---|---|---|---|---|---|---|---|---|---|---|---|---|---|---|---|---|---|---|---|---|---|---|---|---|---|---|---|---|---|---|---|---|---|---|---|
|
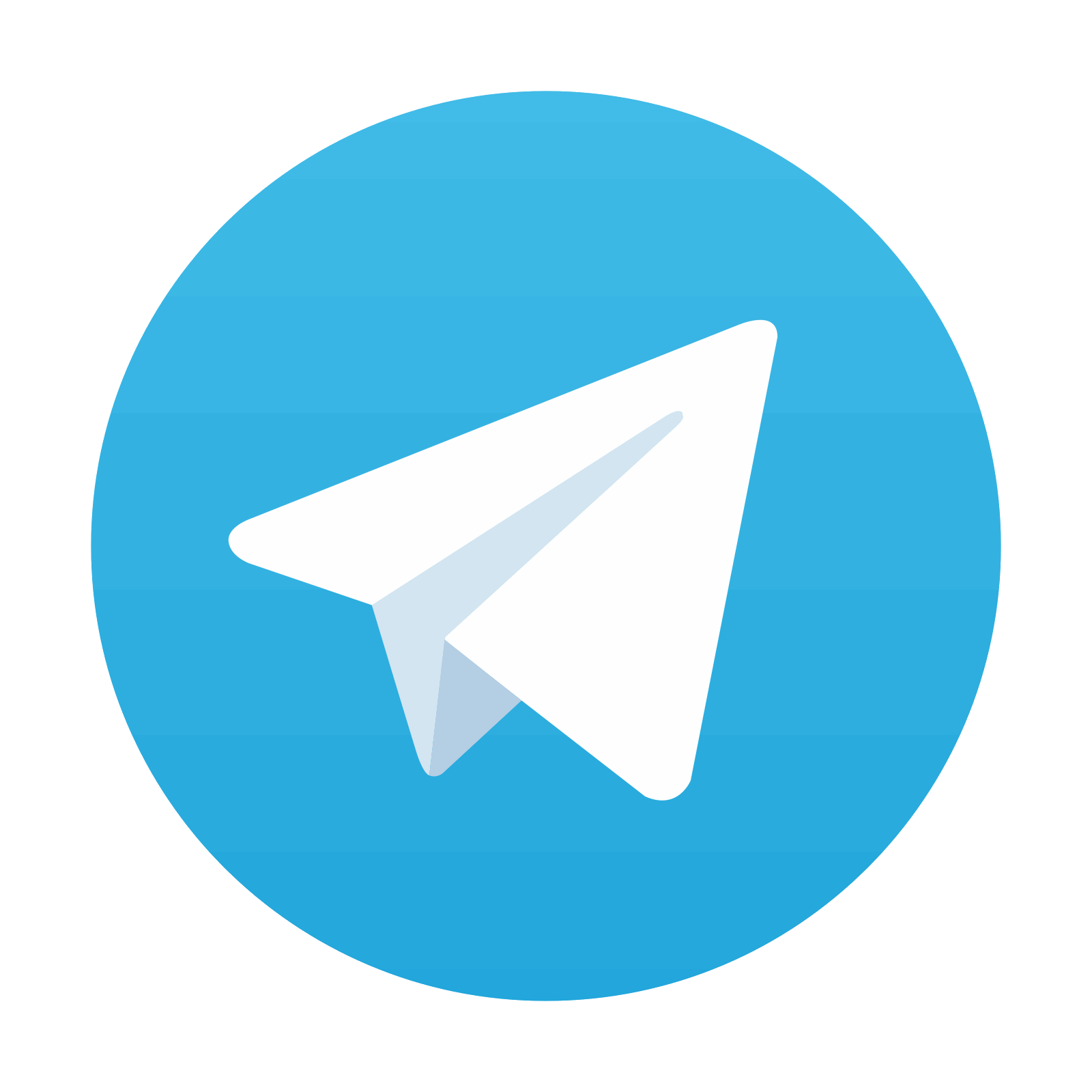
Stay updated, free articles. Join our Telegram channel

Full access? Get Clinical Tree
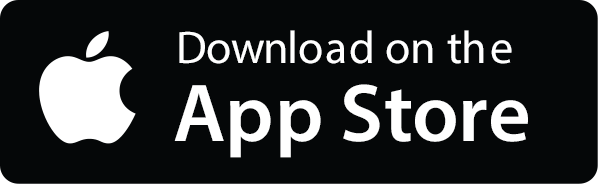
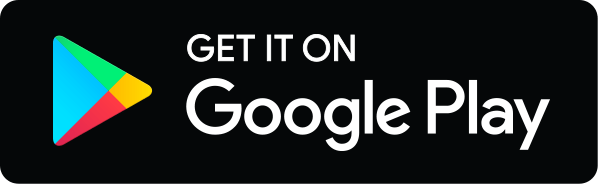
