Purpose
To evaluate the quality of vision and depth of focus induced by controlled amounts of negative spherical aberration in patients implanted bilaterally with light-adjustable intraocular lenses.
Design
Prospective, nonrandomized clinical trial.
Methods
Seventeen patients were implanted and treated with appropriate spatial irradiance light profiles. One eye was set for emmetropia, and the fellow eye received an additional aspheric light treatment to induce controlled amounts of negative spherical aberration. We used a Hartmann-Shack sensor to measure the eye’s refraction and aberrations for a 4-mm pupil diameter. Decimal visual acuity (VA) was measured using a micro-display placed at 10 m, 60 cm, 40 cm, and 30 cm.
Results
Eyes treated with aspheric profiles were divided into 2 groups depending on the final amount of induced negative spherical aberration: low [−0.05, −0.10 μm] and high [−0.13, −0.23 μm]. In both groups, the mean uncorrected decimal VA at 60 cm was over 0.90. In the first group, distance VA was 0.97 ± 0.16, but in the second group it was lower (0.76 ± 0.16). As expected, the VA for nearer distances is higher in the eyes with a larger magnitude of spherical aberration ( P value < .01): 0.94 ± 0.10 and 0.73 ± 0.16 at 40 and 30 cm, respectively, in comparison with 0.71 ± 0.15 and 0.50 ± 0.14. Binocular summation with the fellow eye, adjusted for emmetropia, produces an excellent binocular distance VA (>1.10) in both groups.
Conclusions
Controlled amounts of negative spherical aberration and defocus can be induced in eyes implanted with adjustable intraocular lenses to enhance near vision.
In the last decades, cataract surgery has become a successful procedure to restore vision in many patients. Phacoemulsification, foldable intraocular lenses (IOL), and advances in the calculation of the IOL power have improved the visual outcomes. However, and despite the most recent approaches, refractive surprises (refractive errors higher than 0.5 diopters [D]) after cataract surgery are still frequent, in particular in eyes with a longer-than-normal axial length and in eyes with previous corneal refractive surgery. In addition, values of postoperative astigmatism between 0.5 and 2.0 D, mainly attributable to preoperative corneal astigmatism in combination with what is induced by corneal incisions, are also common.
The light-adjustable lens should allow the physician to obtain optimum refractions after cataract surgery. These intraocular lenses were successfully irradiated with the appropriate spatial irradiance patterns for in vitro correction of spherical and astigmatic refractive errors. In the last years, several clinical studies have reported good results in the correction of spherical refractive errors (myopia and hyperopia) and also astigmatism with light-adjustable lenses. In addition to the correction of refractive errors, light-adjustable lenses can also create higher-order patterns. This offers the possibility to induce controlled amounts of spherical aberration (SA) to increase the depth of focus in patients, allowing for near vision. This approach is implemented and demonstrated in the current study.
Methods
The study was a prospective, nonrandomized clinical trial approved by the ethics committee of the University Hospital “Virgen de la Arrixaca” in Murcia, Spain. All clinical examinations and surgeries were conducted in the Department of Ophthalmology at the Hospital “Virgen de la Arrixaca,” Murcia, Spain. Surgeries were completed during 2010 and 2011 in all patients and performed by the same surgeon (one of the authors, J.M.M.). The complete study followed the tenets of the Declaration of Helsinki. Informed consent was obtained by all patients after they were fully informed about the nature and the possible consequences of the procedures.
Seventeen patients were implanted bilaterally with light-adjustable lenses. In 1 of the eyes, postoperative refractive error was corrected with a first light adjustment, and subsequently a second aspheric treatment induced negative spherical aberration to increase depth of focus. Light profiles weighted with varying amounts of asphericity were customized to produce the desired amounts of induced spherical aberration. The fellow dominant eye was treated to achieve emmetropia. The effects of the binocular summation were also evaluated.
Light-Adjustable Lenses and Spatial Light Intensity Patterns
Light-adjustable lenses (Calhoun Vision Inc, Pasadena, California, USA) contain photosensitive silicone molecules that enable noninvasive postoperative change of their shape by irradiation with ultraviolet light. The posterior surface of each lens is molded with a UV-absorbing layer to impart the pseudophakic patient with additional UV protection for the retina during the irradiation treatment procedure.
Defocus and astigmatism of an implanted light-adjustable lens can be changed by the application of the appropriate spatially resolved irradiance profile and energy dose. Once the final desired refractive state in the patient’s eye is achieved, it is necessary to consume the remaining, unreacted material in the lens by a photo lock-in process. The light-adjustable lens is irradiated using a digital light delivery system (Carl Zeiss-Meditec, Jena, Germany) described elsewhere. It consists of a UV light source, projection optics, and control interface built around a standard slit lamp. A pixelated digital mirror device is used to define a specific high-resolution spatial intensity profile to irradiate the light-adjustable lenses. To adjust the eye’s refraction, custom intensity profiles to correct myopia, hyperopia, and astigmatism were used. Secondary treatments used aspheric profiles with different weighted amounts of asphericity to extend depth of focus. Figure 1 shows examples of different spatial irradiance profiles used to adjust refraction (myopia, hyperopia, and astigmatism) and aspheric patterns to change spherical aberration.
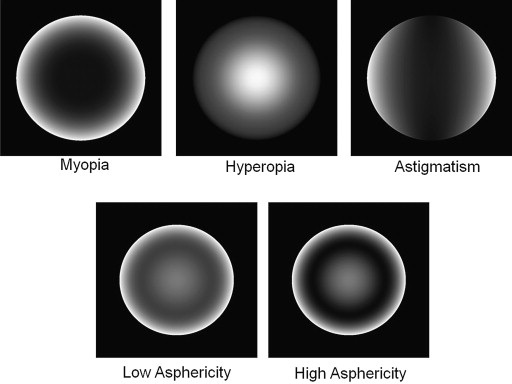
Patients and Surgical Procedure
Light-adjustable lenses were implanted binocularly in 17 subjects. The age of patients ranged between 52 and 79 years old (mean 65 ± 9 years). Initial refractions (mean, SD, and range) before treatments are displayed in Table 1 . At every visit, the clinical examination included slit-lamp biomicroscopy, intraocular pressure, corneal topography (Atlas; Carl Zeiss-Meditec, Dublin, California, USA), biometry, ocular aberrometry, wavefront-guided refraction, and best-corrected and uncorrected visual acuity. At the preoperative, primary adjustment, and 7-month-postimplantation clinical examinations (see below), ophthalmoscopy and retinal optical coherence tomography were also performed. Owing to the limit in astigmatism correction of light-adjustable lenses in vitro, patients with preoperative corneal astigmatism higher than 2.00 D were not included in the study. The IOL powers were selected based on an internally optimized regression analysis program to obtain a spherical equivalent after surgery near to zero or slightly hyperopic. The IOL powers implanted ranged from 17-25 D.
Means ± SD (Min; Max) | ||
---|---|---|
Initial Refraction | Refraction After All Treatments | |
Distance eyes | ||
Sphere | +0.85 ± 1.05 (−1.50; +2.25) | −0.05 ± 0.33 (−0.50; +0.50) |
Cylinder | −0.98 ± 0.57 (−2.25; 0) | −0.33 ± 0.26 (−0.75; 0) |
Equivalent sphere | +0.36 ± 0.97 (−2.00; +1.38) | −0.22 ± 0.38 (−0.75; +0.25) |
Initial Refraction | Refraction After First Adjustment | |
---|---|---|
Near eyes | ||
Sphere | +0.68 ± 0.83 (−0.50; +2.50) | +0.70 ± 0.42 (0; +1.25) |
Cylinder | −0.92 ± 0.59 (−2.00; 0) | −0.55 ± 0.27 (−0.75; 0) |
Equivalent sphere | +0.23 ± 0.73 (−0.88; +1.88) | +0.43 ± 0.43 (−0.38; +1.00) |
The light-adjustable lenses were implanted using standard microsurgical techniques for phacoemulsification extracapsular-type cataract extraction. A temporal corneal incision of 2.85 mm was employed. A dispersive viscoelastic material (Visthesia, Zeiss, Germany, Viscoat 0.5 mL, Alcon Laboratories, Fort Worth, Texas, USA) was introduced into the anterior chamber, and a capsulorrhexis equal to or higher than 5.5 mm was made. Cataract was extracted by stop-and-chop phacoemulsification technique and then cohesive viscoelastic (Healon 10 mg/mL, Abott Laboratories, Abbott Park, Illinois, USA) was also introduced in the anterior chamber. The IOL was introduced in the capsular bag using a lens injector. Residual viscoelastic was aspirated using bimanual technique. The incision was not sutured. A steroidal anti-inflammatory and antibiotic were applied to the eye. The patient was instructed to use UV protective glasses until the second lock-in treatment was completed and the whole process finalized.
Treatment Schedule and Follow-up Visits
Preoperative tests were performed within 15 days prior to surgery. The visit schedule was as follows: first light adjustment: 2 weeks after surgery; second light adjustment, or first lock-in (depends on refraction evaluation): 2-3 days after first adjustment; first or second lock-in: 2-3 days after second adjustment or first lock-in; second lock-in: 2-3 days after first lock-in; post second lock-in: 7-30 days after second lock-in; 3-month visit: 10-14 weeks after second lock-in; 6-month visit: 22-26 weeks after second lock-in; 1-year visit: 50-54 weeks after second lock-in.
Measurements of Refraction and Spherical Aberration
Wavefront aberrations of the eye were measured using a research prototype near-infrared Hartmann-Shack sensor built in our laboratory and adapted to the clinical environment. This system has more than 220 microlenses for a 5-mm-diameter pupil (the size of each microlens on the eye’s pupil is 0.3 mm). The images were recorded in a dark room, allowing a natural pupil diameter larger than 4 mm. To estimate objective refraction, we computed the wave aberration expressed by Zernike coefficients for 3-mm pupil diameter. Spherical aberration was estimated for a 4-mm pupil diameter. The aberrations for the cornea (from corneal topography data using a ray-tracing approach ) were also determined. By direct subtraction, the wavefront aberration of the implanted light-adjustable lenses was then determined. This is a similar procedure to that previously used to determine the aberrations of the natural crystalline lens.
Objective refractions of whole eye and cornea are calculated from the second-order Zernike coefficients:
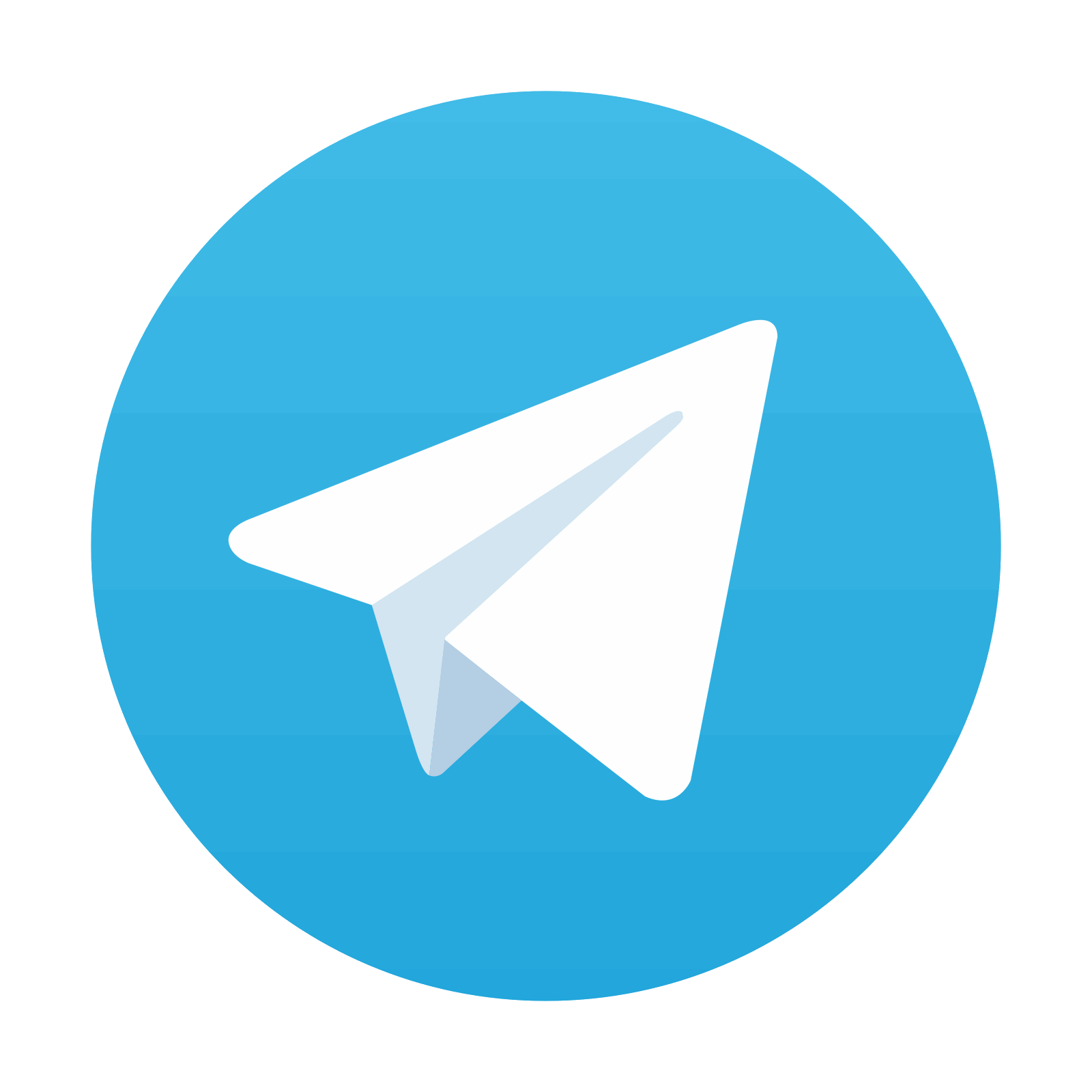
Stay updated, free articles. Join our Telegram channel

Full access? Get Clinical Tree
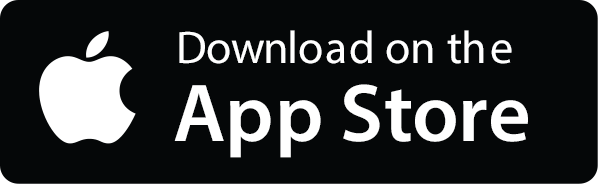
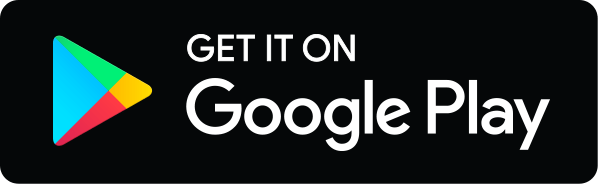