Endoscopic Orbital and Lacrimal Surgery
Erik Kent Weitzel
Raymond I. Cho
With the advent of endoscopic approaches to the sinuses, creative surgeons recognized additional potential for these techniques and quickly developed approaches to the medial and inferior orbit. The benefits were obvious when targeting the orbital apex that is exposed safely and completely with endoscopic techniques. Over time, the techniques have expanded to a wide range of procedures including dacryocystorhinostomy (DCR), optic nerve decompression, and anterior ethmoidal artery (AEA) ligation.
PERTINENT ORBITAL ANATOMY
Bony Anatomy
The orbit is defined as the bony confines circumferentially surrounding the eye and the extraocular musculature. The bony anatomy of the orbit involves a complex contribution of seven different bones of the skull. The medial orbital bone progresses anterior to posterior from the robust frontal process of the maxilla, to a thin lacrimal bone, then to an extremely thin ethmoid bone contribution also known as the lamina papyracea, and finally to the lesser wing of the sphenoid that houses the optic foramen. It is important when performing endoscopic DCR to understand that the lacrimal fossa is formed by fairly equal contributions of two bones joined vertically (see Fig. 43.1): anteriorly, the thicker frontal process of the maxilla and posteriorly, the very thin lacrimal bone. The orbital floor slopes superiorly as it courses from anterior to posterior and includes the orbital surface of the maxillary bone, zygomatic bone (anterolateral), and the orbital process of the palatine bone. The lateral wall consists of the orbital surface of the zygomatic bone anteriorly and the greater wing of the sphenoid posteriorly. The roof consists of the orbital surface of the frontal bone anteriorly and the lesser wing of the sphenoid posteriorly.
Vascular Anatomy
The endoscopic orbital surgeon must be an expert on the vascular anatomy of the orbit, particularly the ethmoidal arteries. Virtually, the entire arterial supply of the orbit arises from the ophthalmic artery, the first branch of the internal carotid artery, which enters the orbit through the optic foramen and gives rise to the central retinal, ethmoidal, lacrimal, zygomatic, supraorbital, and supratrochlear arteries. The AEA enters the anterior ethmoidal foramen through the frontoethmoidal suture at or just under the level of the skull base (fovea ethmoidalis) (Fig. 43.2). Because of its consistent relationship to the skull base, the AEA is safely used as an upper limit of entry into the anterior ethmoidal sinuses when entering the ethmoid sinuses through an orbital approach to prevent cerebrospinal fluid (CSF) leak (also known as external ethmoidectomy). The AEA bridges across the anterior ethmoid sinus between the second and third ethmoidal lamellae (1) along a posterolateral to anteromedial trajectory and back into the intracranial space through the lateral lamellae of the cribriform plate. There is often a natural dehiscence of the orbital bone around the anterior ethmoidal foramen that may be evident after anterior ethmoidal dissection when pushing on the eye. The posterior ethmoidal foramen lies 12 mm posterior to the anterior ethmoidal foramen and houses the posterior ethmoidal artery (PEA). Unlike the AEA, the PEA will not lie in a mesentery and is positioned at the junction of the anterior face of the sphenoid’s intersection with the skull base.
The infraorbital artery, a branch of the maxillary artery, does not normally enter the orbital space, but deserves specific mention because of the potential for the endoscopic orbital surgeon to encounter it during medial orbital decompression. Traveling with the infraorbital nerve and vein, it enters the infraorbital groove/canal from the pterygopalatine fossa, sending a perforating branch into the
orbit through the floor before exiting the maxillary bone in the medial midface.
orbit through the floor before exiting the maxillary bone in the medial midface.
An intimate knowledge of the vascular anatomy of the orbit is essential for the endoscopic orbital surgeon to avoid the potentially devastating consequences of orbital hemorrhage. Maintaining patency of the central retinal artery and ophthalmic veins are critical for vision, and temporary interruptions of the blood supply can lead to permanent visual loss in less than 2 hours. Orbital compartment syndrome frequently develops as a result of retrobulbar hemorrhage or volume-occupying orbital lesions, and the orbit must be decompressed when the orbital pressure nears the occlusion pressure of the central retinal artery. Signs and symptoms of impending visual loss include blurred vision, loss of color vision, afferent pupillary defect, severe proptosis, or ophthalmoplegia. Monitoring intraocular pressures during such events with tenotomy is critical to indicate vascular perfusion to the retina. Intraocular pressures are normally between 10 and 21 mm Hg with a mean of 16 mm Hg. Pressures exceeding 30 to 40 mm Hg necessitate emergency surgical intervention, including lateral canthotomy with inferior and/or superior cantholysis, and orbital decompression if necessary. Studies have shown that lateral canthotomy and inferior cantholysis can effectively reduce intraocular pressures by 30 mm Hg (2). Involvement of an ophthalmologist in the management of orbital compartment syndrome is mandatory.
Neuromuscular Anatomy
Relevant neuromuscular anatomy includes the optic nerve and orbital apex contents. The orbital apex is variably defined, but typically described as the region of the posterior orbit starting at the annulus of Zinn where the rectus muscles originate and extending posteriorly to the optic foramen. Anatomical organization of the posterior orbit is best discussed in relation to the foramina. The superomedially located optic foramen transmits the optic nerve and ophthalmic artery and is located 6 mm posterior to the posterior ethmoidal foramen. The superior orbital fissure (SOF) transmits structures from the middle cranial fossa and is split in two by the origin of lateral rectus located in the annulus of Zinn (Fig. 43.3). The relationship of structures transmitted through the SOF relative to the origin of the lateral rectus defines them as either intra- or extraconal. The superior extraconal neurovascular structures include the lacrimal (V1 branch), frontal (V1 branch), trochlear (IV) nerves, and superior ophthalmic vein. Intraconal nerves include the superior and inferior divisions of the oculomotor (III), nasociliary (V1), and abducens (VI) nerves (3). The inferior orbital fissure provides a passageway into the pterygopalatine fossa and transmits the maxillary nerve (V2), infraorbital artery, and inferior ophthalmic vein (4).
No discussion of the orbit is complete without a discussion of cranial nerve II. The optic nerve is segmented into four parts including the intracranial, intracanalicular, intraorbital, and intraocular segments. From the chiasm, the intracranial component courses approximately 10 mm before entering the optic foramen. The next 8 mm of the optic nerve, called the intracanalicular segment, courses through the optic canal and is the only portion of the nerve confined within bone. The nerve then emerges from the optic canal and extends 30 mm to the globe as the intraorbital segment. The last portion of the nerve is the very small intraocular segment that lacks the three meningeal sheaths and is not myelinated (5).
Lacrimal Anatomy
The lacrimal system lies just anterior to the medial insertion of the orbital septum and therefore is by definition extraorbital although typically discussed in conjunction with orbital topics (6). The overall flow of tears derives from the combined output of the two lobes of the lacrimal gland (orbital and palpebral lobes), the major reflex lubricators of the eye, and small conjunctival glands responsible for basal secretion. After moisturizing the eye, tears enter the superior or inferior lacrimal puncta, course through the superior or inferior canaliculi into the common canaliculus, and emerge into the lacrimal sac through the valve of Rosenmuller. Ten percent of patients will not have a common canaliculus so that the inferior and superior canaliculi penetrate the sac separately. Approximately one-third of the lacrimal sac lies above the common canaliculus (6). This superior lacrimal sac region, known as the fundus, extends above the axilla of the middle turbinate, which becomes important when attempting to marsupialize the entirety of the lacrimal sac. Inferiorly, the sac transitions into the lacrimal duct before emerging under the inferior turbinate through Hasner valve. The lacrimal system is driven by the pump action of blinking, thereby forcing tears through the horizontally oriented canalicular anatomy (Fig. 43.4).
ADULT DACRYOCYSTORHINOSTOMY
Background
The most common patients considered for DCR are females in their early 60s complaining of continuous tearing of a single eye (7). The causes of epiphora are diverse but predictable relative to the patient’s age. Children typically will have a congenitally imperforate Hasner valve effectively treated with short-term lacrimal intubation. Young adults’ epiphora is most likely caused by either trauma related anatomical disturbances or herpetic inflammation with stenosis of the canalicular system. Epiphora in middleaged women is generally caused by a dacryolith formed in the lacrimal sac. Finally, the etiology in elderly women is usually from idiopathic stenosis of the distal lacrimal duct. The two etiologies typical of middle-aged and elderly women are the only ones amenable to DCR. Incomplete obstructions of the lacrimal system will differ from typical
presentations as they tend to only have epiphora in cold or windy weather, but fail to have tearing when inside, and may not develop the infections that are common with complete obstructions.
presentations as they tend to only have epiphora in cold or windy weather, but fail to have tearing when inside, and may not develop the infections that are common with complete obstructions.
The purpose of the lacrimal system is to protect and hydrate the cornea through the continuous production, movement, and drainage of tears. The tear film is a delicately balanced multilayered conglomeration of three different liquid components. The largest contribution comes from the central aqueous layer largely produced by the two lobes of the lacrimal gland with minor contributions from the glands of Wolfring and Krause found within the eyelids. The deep layer of the tear film consists of mucus produced by conjunctival goblet cells that cause the aqueous layer to adhere to the cornea. The superficial layer of tear film is a very thin layer of oil produced by the meibomian glands that prevents evaporation of the aqueous layer.
Evaluation
In true epiphora, tears run down the face, which is to be distinguished from a “watery eye” in which tears do not
drain onto the skin. This distinction is important because epiphora is usually caused by obstructions within the canalicular system or nasolacrimal duct and is treated with surgery, while disorders that cause a watery eye are generally treated medically. While watery eyes also drain through the lacrimal system, the cause is usually an excessive accumulation of tears on the surface of the eye due to heterogeneous tear distribution from ineffective blinking or deficits in tear film layering, which leads to ocular irritation and reflex tearing.
drain onto the skin. This distinction is important because epiphora is usually caused by obstructions within the canalicular system or nasolacrimal duct and is treated with surgery, while disorders that cause a watery eye are generally treated medically. While watery eyes also drain through the lacrimal system, the cause is usually an excessive accumulation of tears on the surface of the eye due to heterogeneous tear distribution from ineffective blinking or deficits in tear film layering, which leads to ocular irritation and reflex tearing.
A comprehensive examination of epiphora begins with careful study of the lids. The position of the punctum should approximate the globe. Ectropion or entropion will lead to abnormal punctal position and prevent normal drainage of tears. The eyelashes should also be inspected to make sure they are not rotated and causing ocular irritation. Evaluation of lacrimal pump problems includes assessment of lid deformities, incomplete blink due to facial nerve weakness, or lid laxity. Lid laxity is tested with the two-part snap test that involves gently pulling the lid away from the eye and releasing it. A normal lid cannot be pulled 6 mm away from the conjunctiva and quickly snaps back into normal position.
The lacrimal system is studied by means of the dye disappearance test, probing of the canaliculi, and irrigation. The examination is begun by anesthetizing the eye topically, followed by placement of a large drop of 2% fluorescein in both eyes. In a normal eye, the fluorescein should clear from the eyes within 5 minutes. Recovery of dye from the nares with a cotton-tipped applicator (CTA) or noseblowing (the primary dye or Jones 1 test) also indicates functional patency of the lacrimal drainage system. Any sign of dacryocystitis on exam suggests obstruction.
If the above tests reveal the presence of obstruction, further testing can identify the location of the obstruction. Lacrimal probing is performed with a 0 or 00 Bowman probe through the canaliculi with care not to create a false passage during probing. A normal exam threads the probe along the canaliculus of interest into the common canaliculus, through the common internal punctum (valve of Rosenmuller), and “hard-stops” when it impacts the mucosa-covered bone of the medial lacrimal sac. If the probe cannot pass soft tissue in the common canaliculus or in the sac, the probe is said to have had a “soft-stop” implying proximal lacrimal system pathology. Finally, lacrimal irrigation is performed with a lacrimal irrigation cannula through the lower or upper canaliculus. It should be easy to irrigate and cause flow into the nose that the patient can taste. Reflux around the needle or out of the opposite canaliculus is abnormal. Probing and irrigation performed after the Jones 1 test is known as the Jones 2 test, and can help to localize the level of lacrimal system obstruction. Reflux of clear fluid from the same punctum being irrigated indicates obstruction proximal to the common canaliculus; reflux of clear fluid from the opposite punctum indicates obstruction of the common canaliculus or common internal punctum; and reflux of fluoresceinstained fluid through the opposite punctum indicates nasolacrimal duct obstruction. A final element of the exam includes a thorough nasal exam to exclude obstructing intranasal pathology (8).
An abnormality detected on this exam suggests lacrimal obstruction, and coupled with appropriate symptoms, is justification for DCR. However, sometimes patients complain of epiphora, but the exam is not convincing for obstructive pathology. In these cases, lacrimal scintillography is indicated to identify those cases with partial obstruction that can still benefit from DCR. Dacryocystogram has also been described as a useful study for identification of complete anatomic blockages; however, this study may be considered redundant to a high-quality exam (Fig. 43.5).
Technique Options
Lacrimal duct and sac obstructions are commonly treated by three procedures including external, endoscopic, and laser-assisted DCR. Outcomes with endoscopic procedures are highly dependent on how the sac is managed, thus any discussion on endoscopic DCR must specify extent of sac marsupialization. The two most commonly discussed variations on endoscopic technique involve either complete preservation and marsupialization of the medial sac or medial lacrimal sac wall excision. Unsuccessful options to be avoided include placement of a grommet through the medial sac wall or a vertical sac slit without
marsupialization, both associated with over 65% failure rates (9). Less common techniques include transcanalicular laser-assisted, balloon dacryoplasty, and conjunctivodacryocystorhinostomy (CDCR).
marsupialization, both associated with over 65% failure rates (9). Less common techniques include transcanalicular laser-assisted, balloon dacryoplasty, and conjunctivodacryocystorhinostomy (CDCR).
TABLE 43.1 EXTENSIVE REVIEW OF THE LITERATURE BY LEONG ET AL. (7) SUMMARIZED TYPICAL OUTCOMES OF POPULAR DCR TECHNIQUES | |||||||||||||||
---|---|---|---|---|---|---|---|---|---|---|---|---|---|---|---|
|
Procedure outcomes vary widely, with typical success rates for the most commonly reported procedures summarized in Table 43.1. Success rates are similar for endonasal and external DCR (10) and decisions regarding choice of procedure are highly dependent on surgeon experience. Laser-assisted DCR suffers from worse long-term results than the other two popular techniques. For atrophic sacs, an alternative technique maintains permanent silastic tubes through an opened sac. If the patient can tolerate the tubes, success rates approximate sac marsupialization (9). However, most patients will complain of foreign body sensation that requires frequent stent adjustment swaying surgeons toward a nonstented option in a majority of these patients.
Adjunctive therapies widely used at the conclusion of DCR including the use of antimetabolite agents and postoperative silicone tubing of the lacrimal system may not be beneficial. Research now suggests that antimetabolites including Mitomycin C or 5-Flourouracil most likely do not improve results (7); however, a few controversial studies have reported better outcomes (14,15). Stenting the proximal lacrimal system with silicone tubing for 1 to 6 months has been a standard postoperative measure to encourage the system to heal in a patent configuration. However, recent reports suggest that tubing may be considered optional with little added benefit to outcomes (16,17).
Endoscopic “access” septoplasty is performed in a majority of cases prior to beginning DCR. This limited septoplasty selectively addresses the anterior-superior septum allowing full exposure to the axilla of the middle turbinate. Additional preparatory work includes utilizing a 30-degree scope for the procedure angled in an upward/lateral direction, injection of the middle turbinate axilla with 1:100,000 epinephrine, and placement of 1:1,000 epinephrine-coated pledgets in the anterior nasal cavity to create vasoconstriction for hemostasis (Fig. 43.6).
The DCR flap is started posterosuperiorly at a point 3 mm posterior to and 8 to 10 mm above the axilla. The incision is developed 10 mm anterior to the axilla and then dropped inferiorly down to the level of the insertion of the inferior turbinate. A final horizontal incision is created at the level of the insertion of the inferior turbinate from the uncinate to the base of the existing incision. A sharp suction freer is used to elevate this flap over the frontal process of the maxilla, off of the lacrimal bone, and on to the uncinate where it will remain posteriorly pedicled throughout the case. It is also important to elevate the flap off of the axilla. The mucosal flap is protected during the case by rolling it medial to the middle turbinate. Hemostasis is maintained by periodically placing pledgets with 1:1,000 epinephrine (Fig. 43.7).
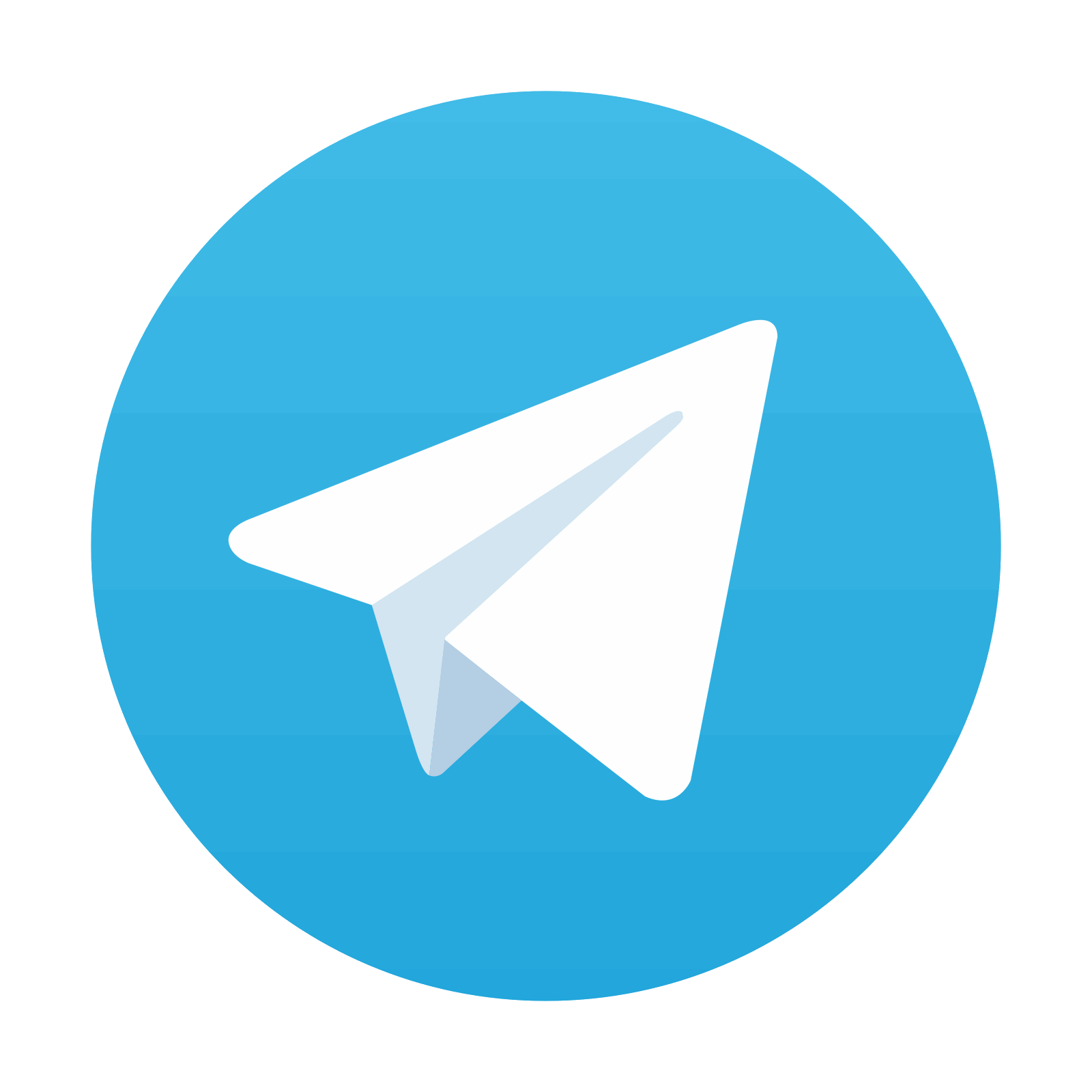
Stay updated, free articles. Join our Telegram channel

Full access? Get Clinical Tree
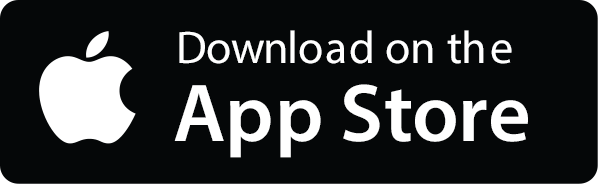
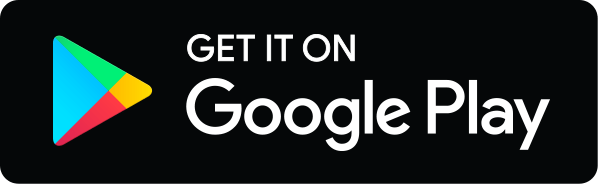