Cancers of the oral cavity account for approximately 3% of malignancies diagnosed annually in the United States. As with other upper aerodigestive tract cancers, 5-year survival rates for oral cavity cancers decrease with delayed diagnosis. Cancers of the oral cavity are thought to progress from premalignant/precancerous lesions, beginning as hyperplastic tissue and developing into invasive squamous cell carcinoma. Despite the general accessibility of the oral cavity during physical examination, many malignancies are not diagnosed until late stages of disease. To prevent malignant transformation of these oral precursor lesions, multiple screening and detection techniques have been developed to address this problem.
Oral cavity cancer accounts for approximately 3% of all malignancies and is a significant worldwide health problem. The American Cancer Society estimates that there will be approximately 5300 deaths in the United States in 2010, with more than 23,000 new cases of oral cancer. Oral cancer occurs most commonly in middle-aged and elderly individuals; however, recent evidence suggests these demographics may be changing. Surveillance, Epidemiology, and End Results (SEER) data demonstrated an increase in the incidence of tongue cancer in young individuals (aged <40 years), from 3% in 1973 to approximately 6% in 1993, and many of the affected individuals are without traditional risk factors. Additionally, research indicates that the traditional male predominance is less overt in young individuals with oral squamous cell carcinomas (SCC).
The majority of oral malignancies occur as squamous cell carcinomas and despite remarkable advances in treatment modalities, the 5-year survival rate has not significantly improved over the past several decades, hovering at about 50% to 60%. The unfavorable 5-year survival rate may be attributable to several factors. First, oral cancer is often diagnosed at a late stage, with late stage 5-year survival rates as low as 22%. Additionally, the development of secondary primary tumors in patients with early stage disease has a major impact on survival. The early detection and surveillance of oral cancer and premalignant lesions offers the promise to not only detect disease at early stages but also to improve the monitoring of disease progression or regression during treatment.
It is well accepted that many oral SCCs develop from premalignant conditions of the oral cavity. A wide array of conditions have been implicated in the development of oral cancer, including leukoplakia; erythroplakia; palatal lesion of reverse cigar smoking; oral lichen planus; oral submucous fibrosis; discoid lupus erythematosus; and hereditary disorders, such as dyskeratosis congenital and epidermolysis bullosa. Much of the published information describing the prevalence of potentially malignant disorders varies by geographic location and population studied. Despite this limitation, a generally accepted prevalence rate ranges between 1% and 5%. The majority of affected patients are middle-aged or elderly men. Potentially malignant disorders are discovered most commonly on the buccal mucosa, lower gingiva, tongue, and floor of the mouth, with the remaining cases distributed throughout the remainder of the oral cavity.
Although it is generally accepted that early detection and screening for oral cancer has the potential to decrease the morbidity and mortality of disease, methods for screening have yet to be proven successful. The United States Preventive Services Task Force found no new good quality evidence that screening for oral cancer leads to improved health outcomes for either high-risk adults (ie, those over the age of 50 who use tobacco) or for average- risk adults in the general population. Additionally, a recent Cochrane review stated “there is insufficient evidence to support or refute the use of a visual examination as a method of screening for oral cancer using a visual examination in the general population. Furthermore, no robust evidence exists to suggest that other methods of screening, toluidine blue, fluorescence imaging or brush biopsy, are either beneficial or harmful”. The following text provides a review of several of the investigated techniques for oral cancer detection, as well as an introduction to optical techniques for oral cancer detection.
The early detection of oral cancer
With the development and success of screening programs for breast, cervical, and colon cancer, the potential to reduce the morbidity and mortality of oral cancer through early detection modalities is of critical importance. Data indicates that the diagnosis of oral squamous cell carcinoma at an early stage of disease allows for less aggressive treatment, improves quality of life, and improves the overall 5-year survival rate when compared with SCCs diagnosed at late stages. The major modalities designed to reduce this burden include oral cavity examination, supravital staining, oral cytology, and optical detection systems.
Oral cavity examination
The examination of the oral cavity has traditionally been the preferred approach for the detection of oral mucosal abnormalities. As a noninvasive technique, the oral cavity examination can be performed quickly, is without additional diagnostic expense to patients, and may be performed by health care professionals across a multitude of disciplines. The evidence regarding oral examination as an effective screening technique, however, remains controversial. In a recently published randomized controlled trial with nearly 130,000 participants, investigators concluded that there was insufficient evidence to support or refute the use of oral examination as a screening program. However, this study, performed by the Kerala group in India, demonstrated improved survival rates at 9 years among men with high-risk habits (tobacco use). Although there was no increase in survival for the overall population, this study was the first to clearly support the efficacy of an oral cancer screening program in a high-risk population.
Oral cavity examination
The examination of the oral cavity has traditionally been the preferred approach for the detection of oral mucosal abnormalities. As a noninvasive technique, the oral cavity examination can be performed quickly, is without additional diagnostic expense to patients, and may be performed by health care professionals across a multitude of disciplines. The evidence regarding oral examination as an effective screening technique, however, remains controversial. In a recently published randomized controlled trial with nearly 130,000 participants, investigators concluded that there was insufficient evidence to support or refute the use of oral examination as a screening program. However, this study, performed by the Kerala group in India, demonstrated improved survival rates at 9 years among men with high-risk habits (tobacco use). Although there was no increase in survival for the overall population, this study was the first to clearly support the efficacy of an oral cancer screening program in a high-risk population.
Supravital staining
Toluidine blue (TB) is an acidophilic dye designed to stain acidic cellular components, such as DNA and RNA. Its use in the detection of precancerous/cancerous tissue is based on the fact that dysplastic tissue contains quantitatively more DNA and RNA than nondysplastic tissue. To perform the staining, a 1% solution is placed on the oral mucosa and removed after 1 to 2 minutes with 2% acetic acid. The clinician then examines the oral mucosa for areas of increased cellular staining. In the evaluation of a potentially malignant oral lesion, TB staining may provide better demarcation of lesion margins, guide biopsy site selection, and is thought to be valuable in identification and visualization of lesions in high-risk patients. Though useful as an adjunct to clinical examination, the specificity of TB staining is limited as cells undergoing inflammatory changes and benign hyperplasia may also retain dye leading to false-positive results. Overall, the sensitivity of TB staining ranges from 0.78 to 1.00 and the specificity from 0.31 to 1.00.
Oral cytology
Oral cytology describes a diagnostic technique employed to sample oral tissue for histomorphologic analysis. To obtain a tissue sample, the clinician applies a stiff brush to the oral mucosa with enough pressure to induce pinpoint bleeding which ensures a full-thickness or trans-epithelial tissue sample. These cellular samples can then be analyzed by a variety of unique diagnostic measures, including cytomorphometry, DNA cytometry, and immunocytochemical analysis.
Computerized image analysis of brush biopsy samples (OralCDx Laboratories Suffern, NY, USA) uses a computer program to perform morphologic and cytologic analysis of tissue samples. The computerized analysis ranks cells based on the amount of abnormal morphology, which are then presented to a pathologist for further distinction and classification. The sensitivity of the OralCDx ranges from 0.71 to 1.00 and specificity as low as 0.32.
DNA cytometry uses a DNA specific Feulgen dye to quantify and identify deviations in DNA content in sampled tissue. Although data are still limited, the addition of DNA measurements to cytologic analysis has been shown to increase the sensitivity and specificity of brush biopsies.
The use of oral cytology in the detection of dysplastic lesions shows considerable promise, but has been limited thus far by variable false-positive and false-negative results.
The optical detection of oral cancer
The field of optical diagnostics comprises a variety of techniques designed to characterize the relationship between the optical and biologic properties of tissue. Through the detection of changes in light after interaction with tissue, optical technologies provide information on the physiologic condition of the tissue at a molecular level. Early research in optical diagnostics suggested that alterations in light-tissue interactions can be used to differentiate normal from malignant tissue. Subsequent advances in molecular biology, genomics, and proteomics, have vastly improved our scientific understanding of the complex biochemical and morphologic changes that occur as tissue undergoes the transformation from normal to neoplasia. Many of these early biologic events have been shown to alter the optical properties of precancerous and cancerous tissue. Light-based detection systems identify these optical signatures created during tissue transformation to provide a real-time assessment of tissue structure and metabolism.
Although the pathways responsible for the development of cancer are complex, it is widely accepted that cancer arises through the accumulation of DNA mutations that lead to unregulated proliferation. The proliferation of squamous cell cancer cells forms a morphologically distinctive spectrum of disease ranging from mild dysplasia to invasive carcinoma. The current identification and diagnosis of precancerous and cancerous lesions relies on the histologic and cytologic examination performed by a pathologist after suspicious tissue is biopsied. Although these methods represent the gold standard for cancer diagnosis, they have several limitations. Tissue biopsy is invasive, expensive, and often time consuming. The diagnostic interpretation of the tissue sample has been shown to vary among pathologists, and the pathologic criteria for the identification of precancerous lesions are not well defined. In addition, early precancerous changes are frequently undetectable by conventional visual inspection, leading to missed opportunities for diagnosis. Optical technologies have the potential to improve these limitations in several ways. Although the benefits provided vary with each technology, optical techniques offering objective data analysis may reduce the variation in pathologic diagnosis. Furthermore, optical technologies show the potential to provide a real-time tissue assessment through a minimally invasive route, eliminating lengthy waits and the need for tissue biopsy. Although the benefits of optical technologies are currently limited in clinical practice, the achievement of a highly sensitive and specific optically determined histopathologic diagnosis, an optical biopsy, has the potential to revolutionize medical practice.
Techniques for the optical detection of cancer
Spectroscopy
The use of spectroscopic techniques for the detection of cancerous and precancerous lesions is based on the analysis of specific light-tissue interactions to assess the state of biologic tissue. As tissue undergoes the carcinogenic sequence from normal to neoplasia, complex morphologic and molecular transformations occur that modify the manner in which light is absorbed and reflected in the tissue. With the delivery of specific wavelengths of light to tissue through an optical probe, a spectral pattern is collected that contains diagnostic information for tissue classification. Using histologically confirmed tissue specimens from benign and neoplastic tissue, scientists have assembled a spectral database of known light-tissue interactions. The spectra collected from an unknown tissue sample can then be analyzed through various empirical and statistical techniques to produce a histologic diagnosis. Spectroscopic techniques, such as fluorescence spectroscopy, light scattering spectroscopy, and Raman spectroscopy, use the unique spectral patterns that are created as tissue progresses toward cancer to offer the potential to detect diseased tissue during the initial stages of carcinogenesis.
Fluorescence Spectroscopy
Fluorescence spectroscopy is based on the biologic emission of fluorescent light from tissue exposed to ultraviolet (UV) or short wavelength visible (VIS) light. To better understand fluorescence, a brief review of the interaction of light and tissue is warranted. Light is formed by packages of energy termed photons. When tissue is exposed to light, photons may be absorbed, reflected, or scattered by specific molecules within the tissue. As light illuminates the targeted tissue, these biomolecules, termed fluorophores, absorb the energy in the illuminating light and respond by emitting fluorescent light of lower energy (and longer wavelength). The change in wavelength then allows fluorescent light to be differentiated from illuminating light (UV or VIS light). Each group of fluorophores will respond to specific excitation wavelengths, and in turn, emit a different range of wavelengths resulting in a spectral pattern that ideally represents the biochemical and metabolic status of the tissue undergoing optical interrogation.
Fluorescent light may be generated by the administration of an exogenous agent, such as in drug-induced fluorescence, or by the excitation of endogenous fluorophores (autofluorescence). As tissue undergoes the biochemical and morphologic progression to neoplasia, the concentration and distribution of the fluorophores are transformed. Known fluorophores include components of the connective matrix (collagen, elastin), metabolic coenzymes (reduced nicotinamide adenine dinucleotide, flavin adenine dinucleotide, flavin mononucleotide), aromatic amino acids (tryptophan, tyrosine, phenylalanine), byproducts of the heme biosynthetic pathway (porphyrins) and lipopigments (lipofuscin, ceroids). Factors influencing tissue autofluorescence include tissue architecture, light absorption and scattering properties of each tissue layer, the distribution and concentration of the fluorophores in the different tissue layers, the biochemical environment, and the metabolic status of the tissue. Though complex, tissue autofluorescence patterns reflect changes in tissue composition and have been shown to be capable of distinguishing benign from malignant tissue.
Elastic Scattering (Reflectance) Spectroscopy
Elastic scattering spectroscopy, also known as diffuse reflectance spectroscopy, uses the principle of white light (400–700 um) reflectance to determine the structural characteristics of illuminated tissue. Elastic scattering occurs when photons from visible light are reflected from tissue constituents without a change in wavelength (or energy). The intensity of this back scattered light is measured resulting in a reflectance spectrum that describes the interaction of white light with tissue following multiple scattering events. As tissue transitions to dysplasia or neoplasia, the relative concentrations, density, and size of endogenous scatterers is affected. The measurement of the intensity of back scattered light is then influenced by the characteristics of the scatterers (ie, nuclei, mitochondria, connective tissue) and absorbers (ie, hemoglobin). For example, dysplastic change is often characterized by enlarged nuclei, crowding, and hyperchromaticity. These changes lead to characteristic reflectance spectra used to identify the structural composition of tissue and aid in clinical diagnosis.
Raman Spectroscopy
Raman spectroscopy is a novel optical technique employed to provide detailed information about the molecular composition of tissue. In contrast to elastic scattering spectroscopy, Raman spectra are generated from the molecule-specific inelastic scattering of light. Following exposure to a light source (generally near-infrared light 700–1300 um), a minute fraction of the scattered light undergoes a wavelength shift caused by the energy transfer between incident photons and tissue molecules. The wavelength shift (and change in energy) is achieved when the incident photon alters the vibrational state of an intramolecular bond. A Raman emission spectrum is generated from the combination of the wavelengths scattered by the molecules in a tissue sample. These spectral features provide detailed and specific information about the molecular composition of tissue. Although Raman spectroscopy is sensitive to a wide range of specific biomolecules, such as proteins, lipids, and nucleic acids, the Raman effect only compromises a small fraction (1 in 1 million) of scattering events and signals may be weak and difficult to implement. Other modifications of the Raman technique, such as surface enhancing Raman spectroscopy and coherent antistokes Raman scattering, are designed to amplify the signal.
Fluorescence Imaging
Fluorescent imaging systems use spectroscopic principles to capture fluorescence emission spectra from a larger tissue sampling area than is possible with point spectroscopy. The acquisition of an image requires tissue illumination with a light source, often in the near-UV to green range. The subsequent fluorescence produced from the absorption and scattering events is recorded with a camera and results in a real-time image. In addition, fluorescence imaging systems are capable of sampling larger tissue areas and provide 2-dimensional information allowing for the detection of lesion-specific features, such as homogeneities.
Optical Coherence Tomography
Optical coherence tomography (OCT) is an innovative optical imaging technique designed to provide high-resolution (approximately 10 to 20 um), cross-sectional images of microscopic subsurface tissue structures. As the optical analog of high-frequency, B-scan ultrasonography, an imaging technique that detects back scattered sound waves, OCT images are generated by measuring the intensity of back scattered light after the tissue is probed with a low-power, near-infrared light source (wavelengths ranging from 750 to 1300 um). Based on the principle of low-coherence interferometry, OCT is able to provide real-time images at a resolution 10 times greater than endoscopic ultrasound, thereby allowing for the identification of microscopic tissue features, such as villi, glands, crypts, lymphatic aggregates, and blood vessels. Despite this high resolution, OCT imaging is limited by a depth of penetration of 1 to 3 mm.
To obtain images, infrared light is delivered to tissue through an optical probe, typically 2.0 to 2.4 mm in diameter. Various OCT system designs allow for tissue to be scanned in a linear, transverse, or radial fashion and are easily interfaced with endoscopes, laparoscopes, catheters, and hand-held probes. Initially applied to obtain images in the field of ophthalmology, technological advancements have resulted in the clinical application of OCT imaging in a diverse set of medical specialties, including gastroenterology, dermatology, cardiology, and oncology.
Narrow-Band Imaging
Narrow-band imaging (NBI) is a recently developed optical technique designed to enhance the visualization of microvasculature on the mucosal surface. Developed to improve the quality of endoscopic images, NBI systems limit the depth of light penetration into tissue through red, green, and blue optical interference filters. These 3 filters divide the visible wavelength ranges into 3 shorter wavelength bands, while increasing the relative contribution of blue filtered light. The increased contribution of blue light is fundamental to creating a narrow-band image because blue light corresponds to the peak absorption of hemoglobin. The resulting image demonstrates preferential enhancement of the vascular network of the superficial mucosa. To differentiate normal from dysplastic tissue, the microvascular patterns present in the narrow-band image are analyzed. Areas of nondysplastic tissue generally have fine capillary patterns of normal size and distribution; whereas, areas harboring dysplasia demonstrate abnormal capillary patterns with increased size, number, and dilation. The clear visualization of vascular patterns through NBI has been shown to enhance the diagnostic capability of endoscopy and offers promise for the early detection of cancer.
Multimodal Optical Imaging
Advances in bioengineering and the continued refinement of optical detection techniques have led to the development of multimodal optical detection systems. These multimodal devices often function in real time to provide complementary diagnostic information and wide tissue surveillance capability. The ultimate goal of the optical detection systems centers on the achievement of an optical biopsy. This achievement would allow clinicians to determine a tissue diagnosis based on in-vivo optical measurements and would eliminate the need for conventional biopsy and histopathologic interpretation. Furthermore, the reliable detection of malignant change through the optical biopsy will provide the clinician the ability to immediately determine definitive treatment and optimally improve patient outcomes.
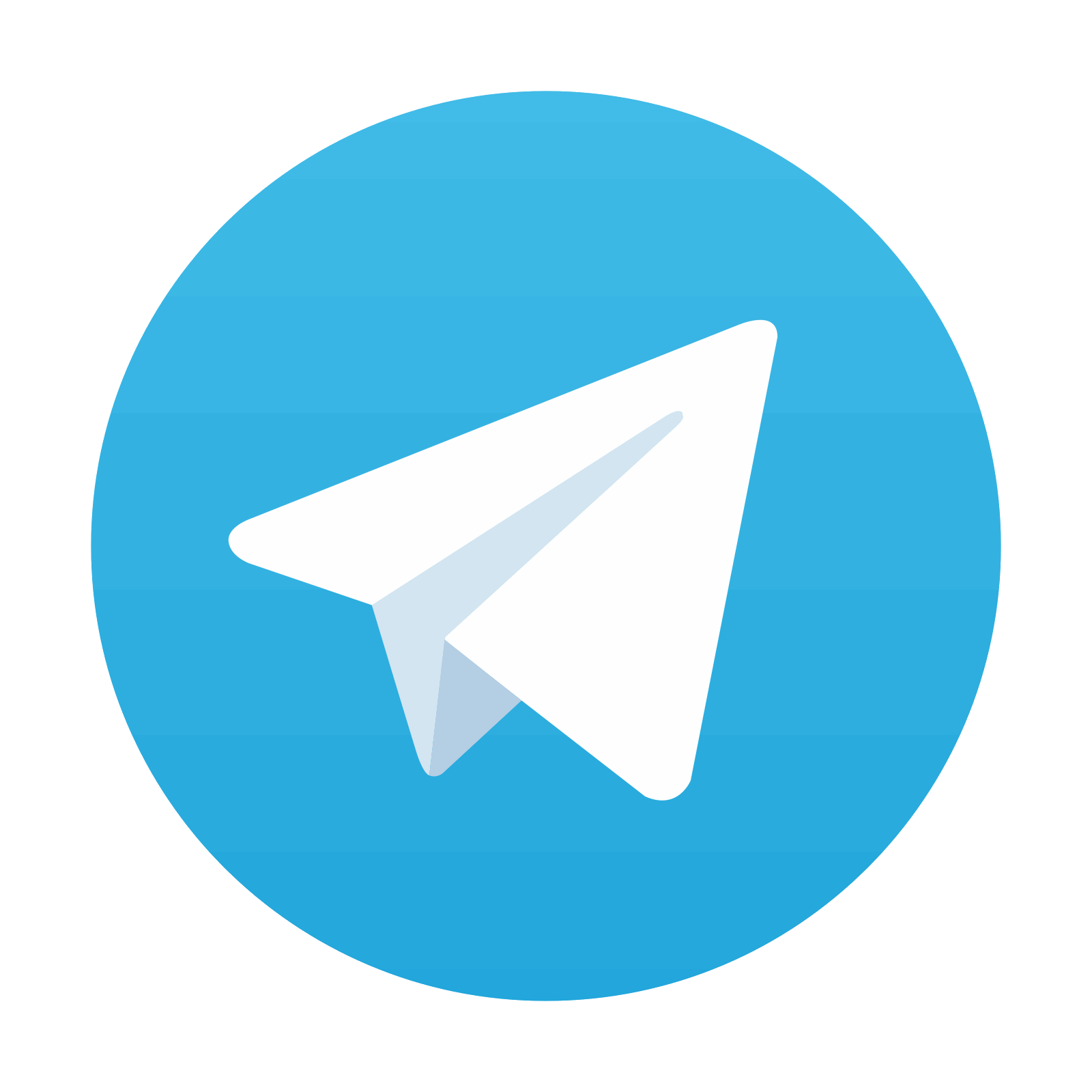
Stay updated, free articles. Join our Telegram channel

Full access? Get Clinical Tree
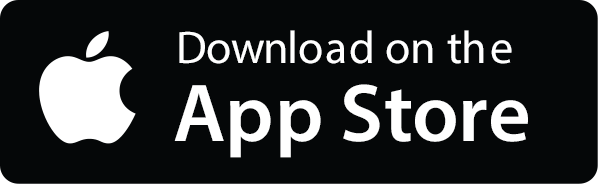
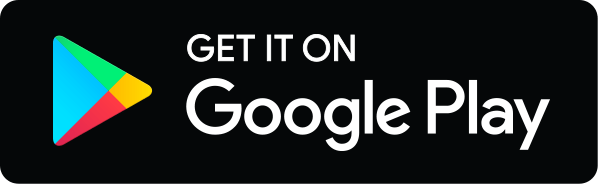