Anatomy & Histology
The normal thyroid gland is located anterior to the trachea and midway between the apex of the thyroid cartilage and the suprasternal notch (Figure 42–1). Important neighboring posterior structures include the four parathyroid glands situated behind the upper and middle thyroid lobes, and the recurrent laryngeal nerves coursing along the trachea. The thyroid consists of two pear-shaped lobes connected by an isthmus. The typical dimensions of the lobes are 2.5–4.0 cm in length, 1.5–2.0 cm in width, and 1.0–1.5 cm in thickness. Also, in about 50% of patients, a small pyramidal lobe is present at the isthmus or adjacent part of the lobes. The functional unit of the thyroid is the follicle consisting of a central collection of colloidal material (thyroglobulin) surrounded by a single layer of polarized epithelial cells.
A normal thyroid gland weighs approximately 10–20 g, depending on dietary iodine intake, age, and weight. The thyroid gland usually grows posteriorly and inferiorly, since it is limited from upward extension by the sternothyroid muscle. In large multinodular goiters, substernal extension is not uncommon.
The thyroid gland has a rich blood supply, derived from the superior, inferior, and the small inferior ima artery (Figure 42–2). Venous flow returns via multiple surface veins draining into the superior, lateral, and inferior thyroid veins.
Thyroid Hormones
The essential steps to thyroid hormone production are as follows (Figure 42–3).
Iodide is actively transported across the basal membrane of the thyroid cell by membrane-bound sodium-iodide symporters (NIS). The iodide concentration inside the cell is about 30 to 40 times greater than in the plasma. NIS action is stimulated by thyroid-stimulating hormone (TSH).
Iodide entering the thyroid cell is oxidized by locally produced hydrogen peroxide to an active iodide intermediate which then covalently binds to the tyrosyl residues in thyroglobulin at the apical-colloid border. The thyroglobulin molecule is a dimer of two identical chains and about 1/3 of the tyrosyl residues of the molecule undergoes iodination. Thyroid peroxidase (TPO) catalyzes both iodide oxidation and iodination of tyrosyl residues.
Linking Pairs of Iodotyrosine Molecules Within Thyroglobulin to Form Thyroxine (T4). and Triiodothyronine (T3) (Coupling)
The coupling of iodotyrosyl residues in thyroglobulin is also catalyzed by TPO. Two molecules of diiodotyrosine (DIT) couple to form T4; and one molecule of monoiodotyrosine (MIT) and DIT couple to form T3. In thyroglobulin molecule containing 0.5% iodine by weight, there are approximately three molecules of T4 and one molecule of T3.
After a variable period of storage in the thyroid follicles, thyroglobulin is taken up into the thyroid cell by pinocytosis. The colloid vesicles fuse with lysosomes containing proteolytic enzymes releasing T4, T3, inactive iodotyrosines, peptides, and aminoacids. The T4 and T3 enter the circulation, whereas MIT and DIT are deiodinated and their iodide conserved.
A small amount of intact thyroglobulin is normally released into the circulation. The amount is markedly increased in thyroiditis, nodular goiter, and Graves’ disease. It is a very useful tumor marker in patients who have had total thyroidectomy and ablation for differentiated papillary or follicular thyroid cancer. Detectable thyroglobulin in these circumstances indicates the presence of thyroid cancer cells.
Thyroid hormone synthesis is mostly controlled by the hypothalamic-pituitary-thyroid axis, as illustrated in Figure 42–4.
Figure 42–4.
The hypothalamic-pituitary thyroid axis. The hypothalamus secretes thyroid-releasing hormone (TRH), which causes the pituitary to release thyroid-stimulating hormone (TSH). In turn, TSH stimulates most of the thyroid hormone formation of T4 and some of the formation of T3. T4 and T3 negatively feed back on the hypothalamus and pituitary, completing the regulatory cycle.
Thyroid hormones are mostly bound to carrier proteins; 99.96% of T4 and 99.60% of T3 are bound in the serum (Figure 42–5). The small fraction of unbound T4 and T3 hormones are responsible for biologic activity. Thyroid hormones are transported throughout the body bound to three carrier proteins in the serum: (1) thyroxine-binding globulin (TBG) which has a single binding site for T4 or T3. In patients who have TBG deficiency, the total T4 and T3 levels are low but the free hormone levels are normal and so the patients are clinically euthyroid. TBG levels are increased during pregnancy and with estrogen therapy. (2) Thyroxine-binding prealbumin (TBPA), also known as transthyretin which binds about 10% of circulating T4; and (3) albumin which binds about 15% of circulating thyroid hormones.
Biologic activity is dependent on the degree and location of iodination (see Figure 42–5). T3 is three to eight times more potent than T4. T4 is the predominant circulating thyroid hormone, whereas T3 is the main peripherally active hormone. The thyroid normally secretes about 100 nmol of T4 and 5 nmol of T3. Most of the peripheral T3, therefore, is derived from circulating T4 by the action of peripheral 5′-deiodinases. Certain drugs can inhibit the conversion of T4 to T3: propylthiouracil, amiodarone, ipodate, glucocorticoids, and propranolol. T4 has a half-life of approximately 7 days, whereas T3 has a half-life of 1 day.
Assessment of Thyroid Function
The most commonly used thyroid function tests in clinical practice are serum immunoassays for TSH (or thyrotropin) and free thyroxine (or free T4, also known as FT4). TSH can be used alone in screening for overt thyroid disease, but both TSH and FT4 are needed for the diagnosis, especially if pituitary or hypothalamic disease is suspected. With a normal hypothalamus and pituitary, TSH maintains an inverse relationship with FT4. Figure 42–6 provides an algorithm for the evaluation of thyroid function tests. The common profiles of thyroid function tests in different disease states are outlined in Table 42–1. TSH is an extremely sensitive pituitary indicator of thyroid disease, but it requires 4–6 weeks to reflect changes in thyroid hormone levels. FT4 is a less sensitive indicator of thyroid hormone production, but it may be helpful in monitoring more acute changes in thyroid activity. In evaluating hyperthyroidism, it may also be helpful to obtain a total T3 or FT3 to rule out T3 thyrotoxicosis.
TSH | Free T4 | T3 | Diagnosis |
---|---|---|---|
Normal | Normal | Normal | Euthyroid |
Normal | Low normal or low | Low or normal | Central hypothyroidism or sick euthyroid or drugs |
High | Low | Normal or low | Primary hypothyroidism |
High | Normal | Normal | Subclinical hypothyroidism |
High | High | High | TSH resistance syndromea |
Low | High or normal | High | Hyperthyroidism |
Low | Normal | Normal | Subclinical hyperthyroidism or drugs |
Low | Normal | High | T3 toxicosisa |
Low | Low | Low | Central hypothyroidism or sick euthyroid or drugs |
The TSH assays currently used are immunoassays based on two monoclonal antibodies detecting different epitopes of the TSH. Most laboratories use either a second- or third-generation TSH assay, which detects levels as low as 0.10 Â and 0.01 mU/L, respectively. TSH is a sensitive measure of the response of the pituitary gland to circulating FT4 levels.
The reference range for most TSH assays is 0.4 to 4.0 mIU/L. It is likely, however, that some individuals with thyroid dysfunction, especially subclinical hypothyroidism, were included when this reference range was established. The TSH level for truly euthyroid subjects lies in the 1.0 to 1.5 mIU/L with a normal range of 0.4 to 2.5 mIU/L
TSH levels are elevated mostly in primary hypothyroidism and are accompanied by a low level of FT4. Table 42–2 lists situations in which the TSH is elevated (other than in hypothyroidism), including drugs, recovery from an acutely ill state, acute psychiatric admission, and the very rare TSH-secreting pituitary tumor.
True hyperthyroidism | Iodine and iodine-containing drugs (amiodarone, IV contrast), lithium, interferon alfa, interleukin 2 |
True hypothyroidism | Iodine and iodine-containing drugs (amiodarone, IV contrast), lithium, interferon alfa, interleukin 2 |
Suppressed TSH secretion | Glucocorticoids, dopamine, dobutamine, octreotide, amphetamines, opioids, nifedipine, and verapamil, dopamine antagonists, atypical antipsychotics, phenothiazines |
Low T4 by decreased absorption of T4 | Cholestyramine, soy-based foods, colestipol, aluminum hydroxide, calcium carbonate, iron sulfate, sucralfate |
Low T4 by increased T4 clearance | Phenytoin, carbamazepine, phenobarbital, rifampin |
High T4 by inhibited T4 to T3 conversion | Amiodarone, iodine and iodine-containing substances, glucocorticoids, propylthiouracil, propranolol |
A decreased TSH level should be interpreted in conjunction with the FT4 level. A decreased TSH level with an elevated FT4 level suggests primary hyperthyroidism. A low FT4 level with a normal to decreased TSH level may indicate secondary or central hypothyroidism (<5% of all cases of hypothyroidism), which are due to a pituitary or hypothalamic tumor. A number of situations, including drugs and nonthyroidal illness, can also cause both low TSH and FT4 levels (see Table 42–1).
Direct FT4 determination by an immunoassay has largely replaced indirect measurements of FT4 concentrations, such as the free T4 index (FT4I), which is the product of resin T3 (or T4) uptake and total T4. Most laboratories use a chemiluminescent immunoassay to measure FT4 levels. It is valid in most cases, except in patients with very high or low thyroid-binding proteins or severe illness. Under these circumstances, the measurement of FT4 levels by equilibrium dialysis is more reliable. FT4 is elevated in hyperthyroidism and decreased in hypothyroidism. Table 42–2 lists the conditions that affect FT4 levels. Of note, the antiepileptic drugs phenytoin, carbamazepine, and rifampin can cause a significantly increased hepatic metabolism of T4. Also, drugs and illness rarely suppress the TSH to undetectable levels. The measurement of FT4 levels by dialysis is spuriously elevated by heparin, which activates lipoprotein lipase, which in turn generates fatty acids that displace T4 from TBG.
Total T3 measures both the free and bound T3 in circulation. Total T3 is helpful in diagnosing hyperthyroidism with elevated T3 levels but normal T4 levels (ie, T3 toxicosis). The preferential secretion of T3 can be seen in early Graves’ disease or toxic multinodular goiter.
Free T3 (FT3) is a newer test that allows for the direct measurement of FT3 levels via a chemiluminescent assay or a radioassay.
TPO is the key enzyme that catalyzes the iodination of thyroglobulin and the coupling of iodinated tyrosyl residues to form T3 and T4. TPO is located on the microvilli at the thyroid-colloid interface. Almost all patients with Hashimoto’s thyroiditis have antithyroid peroxidase (anti-TPO) antibodies present—these antibodies are usually measured to diagnose Hashimoto’s thyroiditis. A large number of patients with Graves’ disease also have anti-TPO antibodies.
The prevalence of TPO antibody positivity in the population is in the range of 5% to 12%. Its prevalence is increased in patients with other autoimmune diseases such as type 1 diabetes and pernicious anemia. A detectable TPO antibody in the absence of an overt thyroid disease is a risk factor for future development of hypothyroidism. TPO antibody positivity is also a risk factor for development of thyroid dysfunction in patients taking amiodarone, interferon-alpha, interleukin-2, and lithium therapies.
Autoantibodies against thyroglobulin are present in patients with autoimmune thyroid disease. Most of these patients are also positive for TPO antibodies. Thyroglobulin antibody is primarily measured in conjunction with estimation of thyroglobulin level where its presence can lead to spurious results (vide infra).
Two classes of TSH receptor antibodies are associated with autoimmune diseases of the thyroid: (a) antibodies that activate the TSH receptor (thyroid-stimulating antibodies (TSAb)) resulting in Graves’ hyperthyroidism; and (b) antibodies that block binding of TSH to its receptor (thyroid stimulation blocking antibody (TBAb)). Both TSAb and TBAb can be detected alone or in combination with Graves’ disease and Hashimoto’s thyroiditis. The relative contributions of the two classes of the antibodies may modulate the severity of Graves’ hyperthyroidism and may change in response to treatment. TSAb, also referred to as thyroid-stimulating immunoglobulin (TSI), is an indirect test that confirms the diagnosis of Graves’ disease. TSI is positive in approximately 90% of patients with Graves’ disease, and negative both in normal patients and patients with Hashimoto’s thyroiditis. Patient serum is incubated with either human thyroid cell culture or hamster ovary cells that express recombinant human TSH receptor; cyclic adenosine monophosphate (AMP) activity is measured. TSI is also of diagnostic value in patients with normal thyroid function who have exophthalmos. The measurement of TSI is helpful during pregnancy—high titers increase the risk of neonatal thyrotoxicosis.
Serum thyroglobulin is the precursor protein required for the synthesis of T4 and T3. The normal measure is <40 ng/mL in individuals with normal thyroid function, and <5 ng/mL in patients after a thyroidectomy. Thyroglobulin is raised when the thyroid is overactive, such as with Graves’ disease or multinodular goiter. In very large goiters, the elevated levels of thyroglobulin reflect the gland size. In subacute or chronic thyroiditis, thyroglobulin is released as a consequence of tissue damage.
Thyroglobulin is a very useful marker for thyroid cancer, both to assess treatment efficacy and to monitor for recurrence after total thyroidectomy and radioiodine 131I therapy. Because thyroglobulin is made only by the thyroid gland, its level serves as an indicator of the presence of thyroid tissue, as in well-differentiated thyroid cancer. It is necessary to measure for endogenous thyroglobulin antibodies as part of interpreting the measurement of the thyroglobulin level. These antibodies can interfere with the assay and give spuriously high or low levels, depending on the measurement method used.
Radionuclide imaging of the thyroid with 123I or 99mTc is useful in evaluating the functional activity of the thyroid. The two tests that use radioactivity to assess the thyroid are the radioactive uptake and scan. Radioactive uptake evaluates thyroid function by reporting the percentage uptake of iodine, whereas the scan produces an image of the distribution of iodine in the thyroid. The radioactive scan gives information regarding the size and shape of the thyroid, as well as information about nodules that are either functioning (“hot” nodules) or nonfunctioning (“cold” nodules). 123I can be used to assess both radioactive uptake and scan, but 99mTc can only be used for scanning. A 99mTc study gives results within 30 minutes, whereas 123I images are obtained at 4–6 and at 24 hours. 123I delivers less radiation than 131I because of its short half-life of 13 hours and the absence of beta radiation. Its gamma photo energy of 159 keV is ideally suited for thyroid scanning. Both 123I and 99mTc are contraindicated in pregnancy.
123I allows assessment of the turnover of iodine by the thyroid gland. After 100–200 μCi of 123I, radioactivity over the thyroid area is measured by scintigraphy at 4 or 6 and at 24 hours. The normal ranges of uptake vary with iodine intake. In areas of low iodine intake and endemic goiter, uptake may be as high as 60–90%. In the United States, with a relatively high intake, the normal uptake is 5–15% at 6 hours, and 8–30% at 24 hours.
Both 123I uptake and scan are useful in delineating the cause of hyperthyroidism. Uptake is elevated in thyrotoxicosis due to Graves’ disease and toxic multinodular goiter. Uptake is low in subacute thyroiditis, the active phase of Hashimoto’s thyroiditis with the release of preformed hormone, exogenous thyroid hormone ingestion, excess iodine intake (from amiodarone, iodinate contrast dyes, or kelp pills), and hypopituitarism. More rare causes include ectopic thyroid hormone production from HCG (human chorionic gonadotropin), struma ovarii, and metastatic follicular thyroid carcinoma. 131I uptake and scans are very useful in monitoring the recurrence of well-differentiated thyroid cancer. Typically, 2–3 mCi of 131I is given to the patient, and images of the thyroid and the whole body are taken to look for recurrence or metastases. If the patient is treated with high-dose 131I to ablate remnant thyroid cancer, a post-treatment thyroid and whole-body scan is often helpful to look for tumor tissue that weakly uptakes iodine.
Severely ill patients exhibit altered thyroid function tests. Most hospitalized patients have lower serum T3 concentrations due to inhibition of the peripheral conversion of T4 to T3 by 5′-deiodinase. Severely ill patients (50% of patients in the ICU and 15–20% of hospitalized patients) can have a low serum T4 level. This low level is mostly due to very low levels of thyroid-binding proteins, but the exact mechanism remains to be elucidated. The degree of T4 depression has been directly correlated with the overall patient outcome. Most hospitalized patients also have slightly depressed but detectable levels of TSH. It has been suggested that hospitalized patients may have a subtle form of central hypothyroidism as a protective mechanism against their ill health and an increased catabolism. Studies have shown that administering thyroxine to patients who are ill has no benefit and may, in fact, be harmful. In the recovery phase of nonthyroidal illness, the TSH level tends to transiently rise before it returns to normal levels.
The assessment of thyroid function in the setting of nonthyroidal illness is difficult and should be undertaken only when there is a strong suspicion of thyroid disease. A straightforward approach to thyroid function tests in a patient who is hospitalized is to measure both TSH and FT4. An elevated TSH, especially >20 μU/mL, is suggestive of primary hypothyroidism. In 75% of cases, patients with an undetectable TSH using a third-generation TSH assay are likely to have primary hyperthyroidism. A depressed but detectable TSH usually accompanied by a low T4 level could indicate nonthyroidal illness, drug effect, subclinical hyperthyroidism, or central hypothyroidism. In these situations, other aspects of the patient history and examination may be helpful in making the diagnosis. The presence of a goiter, known pituitary disease, and thyroid test results obtained before the illness can direct the diagnosis and treatment. If nonthyroidal illness or a drug effect is highly suspected, the intermittent monitoring of thyroid function tests may be warranted.
There are three basic maneuvers in examining the thyroid. The patient should be seated with only a slightly flexed neck to relax the sternocleidomastoid muscles. The thyroid should first be observed while the patient swallows a sip of water. An enlarged gland or nodules can be observed as the gland moves up and down. The thyroid gland should then be palpated from behind the patient, with the middle three fingers on each lobe of the gland. While the patient swallows, thyroid nodules or an enlargement can be noted as the gland passes beneath the examiner’s fingers. A normal thyroid is usually found to be 2 cm in length and 1 cm in width. A generalized enlargement of the thyroid is called a diffuse goiter (from gutta, Latin for “throat”), whereas an irregular enlargement is termed a nodular goiter.
Thyroid Masses
The prevalence of palpable thyroid nodules is approximately 5% in women and 1% in women. High resolution ultrasonography can detect thyroid nodules in 19% to 67 % of randomly selected individuals. The nonpalpable nodules discovered on ultrasound or other imaging studies are referred to as incidentally discovered nodules or “incidentalomas.” The clinical importance of having a thyroid nodule rests on the need to exclude thyroid cancer. About 5% to 15% of nodules are malignant depending on age, sex, radiation exposure, and family history.
The initial evaluation of a thyroid nodule involves a careful history taking and physical examination (Table 42–3). Generally, incidental nodules over 1 cm in size discovered on imaging studies should undergo further evaluation since they have a greater potential to have clinical significant malignancies. Nonpalpable nodules have the same risk of malignancy as palpable nodules of the same size. Smaller than 1 cm incidental nodules, however, should be evaluated if there are suspicious sonographic findings or have additional risk factors for thyroid cancer. Risk factors include age, with adults younger than 30 or older than 60 years of age carrying a high risk for thyroid cancer; history of childhood head or neck irradiation or total body irradiation for bone marrow transplantation; and family history of thyroid cancer or thyroid cancer syndrome such as Cowden’s syndrome, familial polyposis, Carney complex, multiple endocrine neoplasia [MEN] 2 syndrome in a first-degree relative. Recent growth, or evidence of hoarseness, dysphagia, or obstruction, should also raise suspicion. Incidental thyroid nodules are found in approximately 1–2% of people undergoing 2-deoxy-2[18F]fluoro-d-glucose positron emission tomography (18FDG-PET) imaging. The risk of malignancy in these 18FDG-positive nodules is about 33% and the cancers may be more aggressive and therefore undergo prompt evaluation.
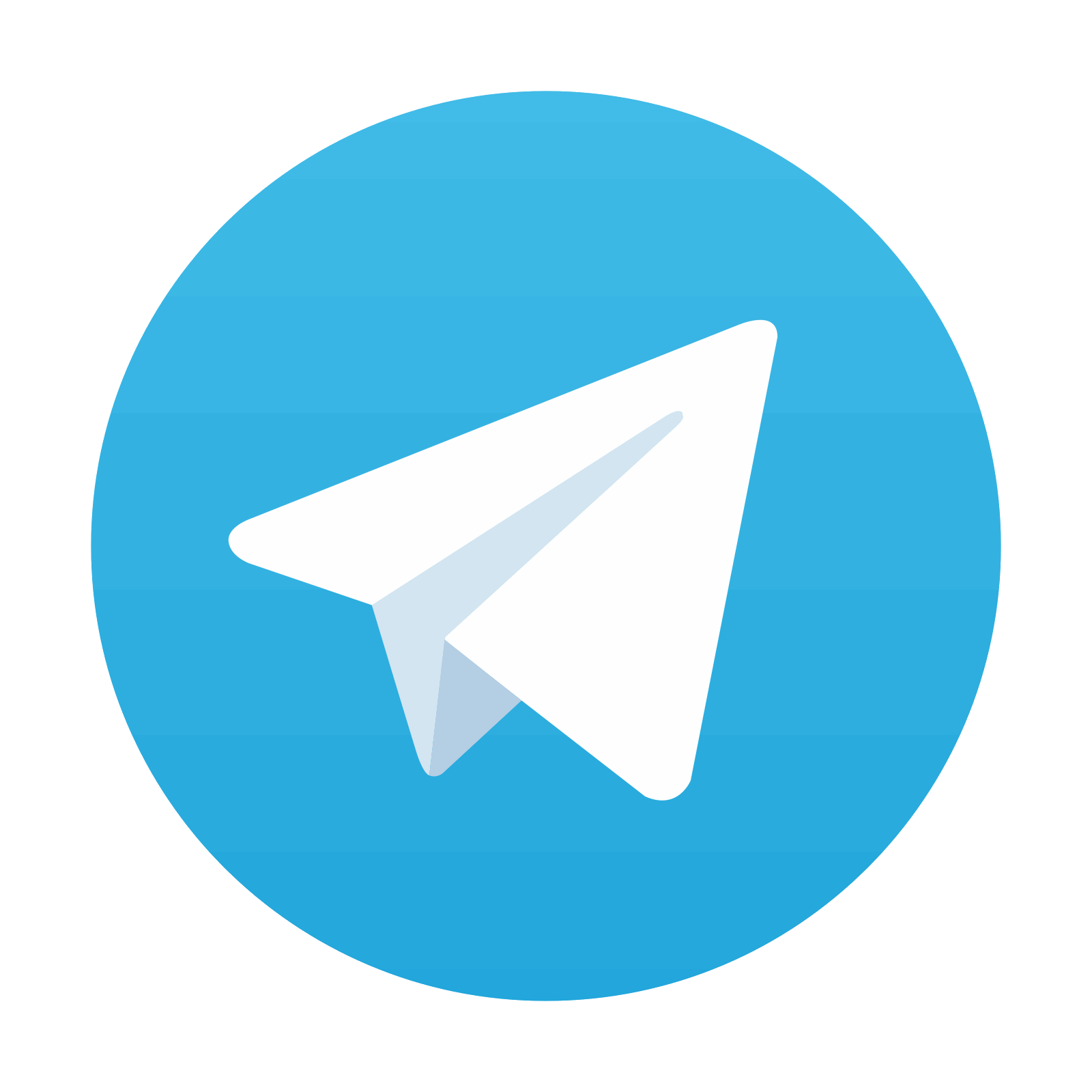
Stay updated, free articles. Join our Telegram channel

Full access? Get Clinical Tree
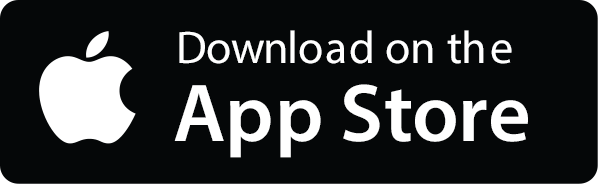
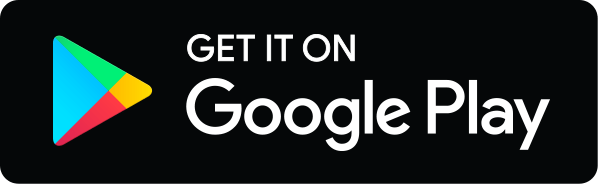
