Diagnostic Ophthalmic Ultrasonography
Yale L. Fisher
Fabio Nogueira
Danielle Salles
Developed in the late 1950s and early 1960s,1,2,3,4,5 diagnostic ophthalmic ultrasonography remains a critical ancillary test for clinical evaluation of the opaque media globe and abnormal orbit. In clear media, its capabilities for tissue characterization have made it extremely useful for intraocular and orbital tumor identification, especially when coupled with clinical, radiographic, and magnetic imaging information. Real-time, kinetic ultrasound technique and improved image quality also permit hyaloid positional evaluation of clear vitreous. Further, recent computer advances have made possible tomographic three-dimensional (3D) ultrasonic imaging of the anterior and posterior segment.
BASIC PRINCIPLES
Ultrasound is an acoustic wave with a frequency greater than 20 kHz. The frequencies used in diagnostic ophthalmic ultrasonography are 8 to 25 MHz for imaging the posterior segment of the eye and orbit and 50 MHz for imaging the anterior segment.
Clinical diagnostic forms of ultrasound are based on physical principles of pulse-echo technology and tissue acoustic impedance mismatch. Pulse-echo technology employs synthetic crystal transducers to produce ultrasonic wavefront pulses and retrieve echoes for electronic display and processing. Send-receive sequences are repeated thousands of times each second.
Echoes are generated at adjoining tissue interfaces that display differences in acoustic impedance: the greater the difference, the stronger the echo. Retinal tissue, for example, is sonically much different than vitreous (which is essentially water). Therefore, strong reflections occur at such interfaces. When adjoining tissues have relatively small differences in acoustic impedance, as with mild vitreous hemorrhages or clumped intravitreal cells, small or weak reflections are seen.
Similar interpretation principles are useful in evaluating types of ocular and orbital tumor tissue. Some lesions demonstrate low-amplitude internal reflectivity; few echoes are seen, and such lesions are described as ultrasonically homogeneous. In contrast, others demonstrate high-amplitude internal reflections; multiple strong retinal echoes are seen, and these lesions are described as ultrasonically heterogeneous. Either tissue type may in addition shadow deeper structures by absorbing or reflecting sonic energy. The presence or degree of shadowing often aids interpretation of ocular and orbital lesions by demonstrating sonic attenuation.
Once reflected, echoes are received, amplified, and electronically processed. They are displayed in visual format as A-scan or B-scan images. A-scan (A-mode or time-amplitude) ultrasonography is used predominantly for tissue characterization and interpretation. The horizontal axis of this display represents time or distance and is useful for linear measurements, whereas the vertical dimension reveals echo amplitude and is useful for characterizing tissues (Fig. 1). A-scan may be performed independently of B-scan. B-scan (B-mode or intensity-modulated) ultrasonography provides a cross-sectional image of the globe and orbit (Fig. 2). B-scans are used predominantly for topographic information. There are overlaps of information from A-scan and B-scan; most physicians rely on both forms for adequate ultrasound interpretation, and instruments are available that present B-scan and A-scan images simultaneously (Fig. 3). The display images are presented horizontally in contact scanning and vertically with waterbath (immersion) scanning6,7,8 (Fig. 4).
![]() Fig. 1. A-scan time amplitude ultrasonogram. The horizontal axis represents time or distance. The vertical deflections represent echo amplitude. |
EXAMINATION TECHNIQUES
The techniques developed for clinical examination have remained essentially unchanged for the past 20 years.9 A formal examination technique is used for architectural evaluation and tissue characterization.
The B-scan image, a two-dimensional crosssectional display of the globe and orbit, is more easily interpreted than A-scan, especially by examiners familiar with interpretation of ophthalmic pathology, where microscopic and gross crosssectional evaluation of the globe and orbit are routine and often topographically similar to B-scan cross-sections.
Several concepts are critical to accurate interpretation of B-scans. These concepts, although relatively easy to define, require considerable experience before the examiner feels comfortable with diagnosis in opaque media situations. These concepts are real time, gray scale, and threedimensional thinking.
Real time refers to the display of motion or movement during B-scan imaging. This capability is one of the greatest advantages of B-scan. Differences in mobility of a variety of movable intraocular abnormalities can be easily detected. For example, the rapid movement of vitreous hemorrhage is usually easy to appreciate and differentiate from the slower, undulating movements of a recent, rhegmatogenous retinal detachment. On occasion, movement of intraocular structures can be used for detecting abnormalities that lie immediately behind vitreous opacification and may be seen only when the vitreous opacities are moved away from the ocular wall, set in motion by command voluntary movements of the patient’s globe (Fig. 5). Gray scale refers to the variable gray tone of display screen echoes. Strong returning signals (echoes) appear white, whereas weaker reflections are seen as gray, the shade depending on echo strength. Examples of strong reflectors of sound include retinal tissue, sclera, and calcifications of any type (Fig. 6). Weaker echoes from clotted or clumped cells within the vitreous are usually easy to differentiate from the stronger reflectors of sound (Fig. 7). Grading of echo strength may be performed only when the examining ultrasound beam is perpendicular to the object of interest, ensuring the strongest possible reflections. However, in the world of ultrasound interpretation, there are many exception to the rules. Tissues that are usually mild reflectors of sound, such as hemorrhage, can at times appear quite strong, especially in complex or multiple pattern disorders, as frequently occur in severe ocular trauma. These apparent inconsistencies often cause misinterpretation by the novice. With experience obtained by multiple examinations, careful follow-up, and clinical correlation whenever possible, diagnostic acumen rapidly improves.
![]() Fig. 7. Contact B-scan. Clotted or clumped cells, such as those seen in mild vitreous hemorrhage, appear pale gray. |
3D thinking is the third B-scan concept necessary for accurate interpretation, and the most difficult to master. During B-scan examinations, the contact probe is almost always in motion, creating multiple, ever-changing two-dimensional B-scan cross-sections that must be mentally combined to create a 3D image of the globe and orbit. This technique is most easily mastered by those familiar with 3D spatial relations. The recent development of tomographic ultrasonography makes this concept easier to master because the student can use the computerized 3D image repeatedly, creating a wide variety of theoretical tomographic slices from the 3D image. Recognition of these patterns during future examinations becomes easier.
During contact B-scan imaging, each quadrant of the globe is scanned systematically from probe positions that avoid, if possible, any passage of the examining or returning sound through the artifact-inducing lens system (Fig. 8). For the beginner, practice with clear media patients, such as those with total or partial retinal detachment, is essential (Fig. 9). The ultrasonically derived vitreoretinal drawing can then be compared to standard optical examinations. As experience increases, similar but more difficult cases can be attempted. Specifically, B-scan examination of diabetic patients with traction retinal detachments and clear media should be attempted and compared to visually controlled drawings. These self-testing exercises, especially in complex cases, provide the examiner a feeling for his or her level of competence and reliability in 3D thinking. Opaque media cases can then be approached with greater confidence and diagnostic accuracy.
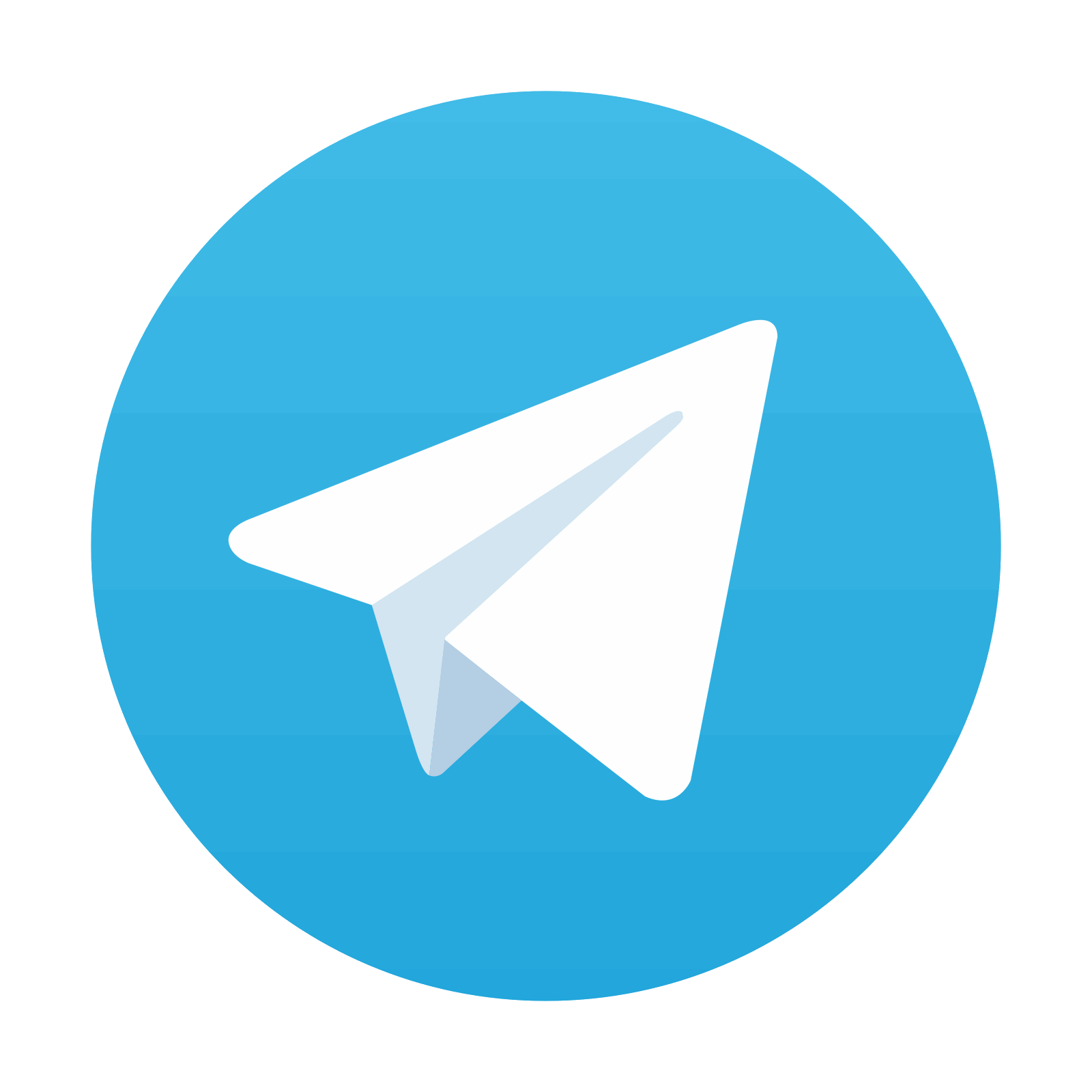
Stay updated, free articles. Join our Telegram channel

Full access? Get Clinical Tree
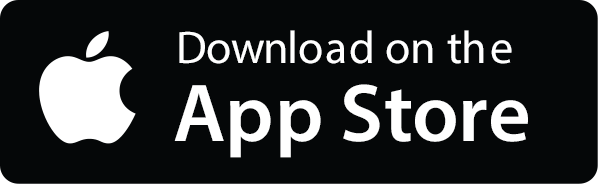
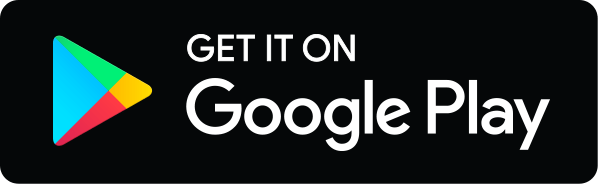